Hemodynamic Management and Shock
Lucas P. Neff
Michael C. Chang
Shock is defined as a functional uncoupling between the cardiovascular system and the peripheral tissues which leads to inadequate delivery of oxygen, energy, and other vital nutrients to the cells of the body. This uncoupling can be due to one or more of several different mechanisms, ranging from primary cardiac failure to inadequate red blood cell mass and subsequent failure of oxygen delivery. A thorough knowledge of the causes and progression of this disorder is essential to treating patients with shock, as management of the shock state is directly determined by a careful and comprehensive knowledge of the etiology of shock in any given patient. Failure to identify and treat the stimulus for shock, coupled with inadequate resuscitation, leads to multiple organ failure (MOF), the leading cause of delayed mortality in the surgical intensive care unit (ICU). Shock is much more than simply a process marked by hemodynamic dysfunction. It has a very latent, yet dangerous inflammatory component as well. Prolonged periods of uncompensated shock are an important stimulus for dysregulated systemic inflammation, and failure to downregulate this inflammatory response leads to persistent hypermetabolism, functional malnutrition, and MOF. This delayed organ failure and mortality can be minimized by detecting shock in its early stages and aggressively resuscitating the patient while addressing the underlying causes.
The conceptual outline of this chapter encompasses the various causes and classifications of shock, clinical findings in the shock patient, and the pathophysiology of shock on both the hemodynamic level and the biochemical level. The chapter focus then turns to methods of hemodynamic monitoring with respect to treatment strategies and resuscitation endpoints.
CAUSES AND CLASSIFICATION OF SHOCK
There are many ways to classify shock. Although classification schemes may vary, all share a common pattern. There is an initial period of compensation which, lacking adequate stimulus control and resuscitation, will give way to decompensated shock. Although both are reversible to a point, failure to address either compensated or decompensated shock leads to an irreversible condition, which eventually leads to MOF and death.
Cardiogenic
Cardiogenic shock can be defined as failure of the heart to generate enough power to deliver oxygen, energy, and nutrients to meet the demand of the peripheral tissues. This failure is attributable to inadequate myocardial contractility. Myocardial contractility is defined as intrinsic ventricular function that is independent of preload and afterload. Inadequate contractility can be due to a number of causes, including myocardial ischemia, infection, and hypertrophy or atrophy of myocardial tissue. Cardiogenic
shock can also result from the diastolic dysfunction of a stiff, noncompliant ventricle that cannot adequately relax to allow ventricular filling to occur.
shock can also result from the diastolic dysfunction of a stiff, noncompliant ventricle that cannot adequately relax to allow ventricular filling to occur.
Regardless of the underlying cause, increased strain is put on the already taxed heart as its own metabolic demands increase in the context of the entire body needing more perfusion and greater effort from the heart. This vicious cycle can lead to rapid decline in already compromised cardiac performance and worsening of the patient’s condition.
Other “cardiac-related” causes of shock include constrictive and obstructive shock. Although these two categories differ in name, the pathology is similar. The underlying issue is that the venous return to the heart is impeded, thereby reducing cardiac preload and leading to reduced cardiac output. Constrictive shock generally relates to processes that prevent ventricular filling, such as cardiac tamponade. Obstructive shock, on the other hand, encompasses situations like tension pneuthoraces in which the blood never even returns to the right atrium.
Hypovolemic Shock
Inadequate preload leads to suboptimal ventricular performance, independent of afterload and myocardial contractility. Decreased intravascular volume results in inadequate stretching of the myocardial fibers before contraction. Regardless of the reason, low intravascular volume leads to decreased transport of oxygen, energy, and nutrients to end organs. The intravascular depletion can be due to any pathologic state that causes blood loss, increased capillary permeability, or loss of venous capacitance.
Neurogenic Shock
Neurogenic shock typically occurs in the setting of a high spinal cord injury that also includes the sympathetic chain. Loss of the sympathetic tone on the highly compliant venous system leads to an increase in unstressed venous capacitance, which in turn leads to decreased preload and subsequent functional cardiac failure.
Septic Shock (Distributive Shock)
Septic shock differs little from the previously mentioned types with respect to what is ultimately occurring at the vascular level. In fact, it is very similar to anaphylactic shock in that the real causative agent is the body’s immune response to a threat, whether real (sepsis from bactremia and inflammation) or perceived (the benign antigens that evoke the activation of innate immunologic systems in anaphylaxis). Essentially, massive immunologic activation from bacterial toxins and dying or injured tissues evokes the same pathophysiologic process of vascular leakage and subsequent loss of intravascular volume. Although there are various subsets of septic shock, endotoxic shock is one of the more common types. Endotoxin is actually lipopolysaccharide (LPS), an integral protein embedded in the cell wall of gram-negative organisms. As the bacteria are destroyed by antibiotics and the body’s own defense mechanisms, LPS is elaborated and free to circulate in the blood. The massive inflammatory response comes as LPS interacts with immune cell surface receptors (toll-like receptors) that can recognize a wide array of primitive antigens that humans have encountered for eons. Therefore, in an attempt to eliminate invading pathogens, the host receives one final blow from the dying bacteria as the immune system undergoes the devastating consequences of unregulated activation.
Although several etiologies of shock are mentioned previously, the causes of shock are even more varied than the types. Although it is important to know the many causes for shock in patients, there is also a simple principle that unifies them. Different types of shock and their particular causes can overlap and exist simultaneously. This may not be intuitive, but quickly makes sense as one plays out the various scenarios of a patient’s course. For example, a patient in septic shock may put such a demand on the heart to maintain cardiac output in the face of low systemic pressure that the myocardium cannot meet that demand and begin to fail. This will lead to cardiogenic shock and a rapid downward decline. For trauma patients it may be a different situation. Perhaps the hypovolemia of hemorrhagic shock leads to decreased gastrointestinal (GI) mucosal blood flow and eventual bowel ischemia. The clinical picture and resuscitation are further complicated by the dying tissue. Therefore, a patient can be in shock for more than one reason. It is imperative that all the data is correlated with the clinical picture and good judgment to ensure that one key component of shock is not overlooked.
CLINICAL FINDINGS
A thorough physical examination and collection of all the clinical and laboratory data can help establish a diagnosis of shock and the degree of severity. Furthermore, physical and clinical findings should always be taken in context, as multiple pathophysiologic states can exist simultaneously. Important clinical signs include abnormal vital signs, changes in neurologic status, decreased urine output, and changes in extremity temperature (see Table 1). These clinical signs can be either a direct result of the source of shock (tachycardia, bradycardia, hypotension) or a clinical finding resulting from functional hypoperfusion (oliguria, agitiation).
Other Markers of Shock Severity
Measurement of blood lactate levels can be useful in determining shock status, both as a marker of adequacy of ongoing resuscitation and as a predictor of occult shock
not manifest by clinical findings. Produced by cells that have converted to anaerobic metabolism to sustain cellular function, elevated lactate levels are an indicator that tissues are not supplied with adequate oxygen to meet their metabolic demands. The sole source of lactate is pyruvate, the end product of glycolysis. This transformation takes place in the cytoplasm of cells during periods of hypoxia and results in grossly inefficient production of adenosine triphosphate (ATP) when compared to ATP production during aerobic conditions. This anaerobic process can occur in any of the body’s tissues, but most lactate production takes place in skeletal muscle, the brain, red blood cells, and the renal medulla. Lactate is cleared both by renal elimination and by the conversion back to pyruvate in the liver. The elevation of lactate occurs when production exceeds normal clearance mechanisms. This is especially true in terms of hepatic metabolism of lactate. During periods of hypoperfusion and hypoxia, the inability of the liver to metabolize lactate through lactate dehydrogenase is juxtaposed with its own increased production of the anaerobic by-product.
not manifest by clinical findings. Produced by cells that have converted to anaerobic metabolism to sustain cellular function, elevated lactate levels are an indicator that tissues are not supplied with adequate oxygen to meet their metabolic demands. The sole source of lactate is pyruvate, the end product of glycolysis. This transformation takes place in the cytoplasm of cells during periods of hypoxia and results in grossly inefficient production of adenosine triphosphate (ATP) when compared to ATP production during aerobic conditions. This anaerobic process can occur in any of the body’s tissues, but most lactate production takes place in skeletal muscle, the brain, red blood cells, and the renal medulla. Lactate is cleared both by renal elimination and by the conversion back to pyruvate in the liver. The elevation of lactate occurs when production exceeds normal clearance mechanisms. This is especially true in terms of hepatic metabolism of lactate. During periods of hypoperfusion and hypoxia, the inability of the liver to metabolize lactate through lactate dehydrogenase is juxtaposed with its own increased production of the anaerobic by-product.
TABLE 1 CLINICAL FINDINGS OF SHOCK | |||||||||||||||||||||||||||||||||||
---|---|---|---|---|---|---|---|---|---|---|---|---|---|---|---|---|---|---|---|---|---|---|---|---|---|---|---|---|---|---|---|---|---|---|---|
|
Rapid clearance of lactate has been associated with a decreased incidence of MOF and death. Elevated lactate levels at admission and the inability to normalize these levels after 48 hours have been correlated with much higher mortality levels. In a prospective resuscitation study of trauma victims, there was an 86% mortality rate for patients who were unable to clear their elevated lactate levels within the first 48 hours. This is a stark contrast to the 100% survival of all patients in the study who normalized lactate values in the first 24 hours and 25% mortality of those who normalized within the 24- to 48-hour period. Therefore, lactate levels provide a fair prognostic indicator and can be used as one of the many variables to assess resuscitation efficacy. Although this observation does not conclude that lactate is the direct cause of mortality, it should be noted that lactate itself has deleterious effects of physiologic function. The acidosis caused by increased levels of lactate can decrease cardiac performance, increase sympathetic discharge of catecholamines, and increase protein catabolism and many other counterproductive processes.
Arterial base deficit (BD) is another readily available marker of widespread metabolic acidosis. Used as a marker of lactate levels, it is easily calculated from the pH and Paco2 values in arterial blood gases and is a powerful predictor of survival in critically ill patients. Furthermore, the utility of BD extends longer than just the initial resuscitative period. Elevated levels of BD over long periods of time (up to 96 hours) can indicate ongoing oxygen debt and herald adverse outcomes, especially given the fact that acid-base homeostasis is usually achieved rapidly. Persistent elevation of BD is a good indicator of ongoing and cumulative dysoxia throughout the resuscitation of the critically ill patient.
SHOCK AT THE MACRO AND MICRO LEVEL
As with many diseases, shock is not a static process. Rather, a fairly dynamic progression of events takes place. Hemodynamic compromise and subsequent decrease in delivery of oxygen to the tissues (regardless of the etiology) leads to a progressive inflammatory response that leads to MOF. Moreover, this progression can cycle back on itself to exacerbate any step in this continuum. Aggressive resuscitation and removal of the underlying stimulus are the cornerstones of attenuating this damaging inflammatory response. Surgeons in generations past understood that shock lead to death, but they often missed the key phenomenon of inflammation now observed because their patients simply did not survive past the initial resuscitative efforts. As medicine advanced and new technologies developed, better resuscitation and the technical ability to support or replace the physiologic function of organs with machines gave clinicians a chance to see beyond the initial encounter in very sick patients to
witness an entirely new obstacle—systemic inflammatory response syndrome (SIRS). SIRS came to the forefront in Vietnam as battle-wounded GIs started to fall victim to “Da Nang lung,” the condition now known as acute respiratory distress syndrome (ARDS), the pulmonary manifestation of SIRS. This “new” inflammatory phenomenon was actually an uncovering of the natural progression of a pathologic process, which was not seen until the 1960s because those that would have gone on to develop SIRS never survived long enough.
witness an entirely new obstacle—systemic inflammatory response syndrome (SIRS). SIRS came to the forefront in Vietnam as battle-wounded GIs started to fall victim to “Da Nang lung,” the condition now known as acute respiratory distress syndrome (ARDS), the pulmonary manifestation of SIRS. This “new” inflammatory phenomenon was actually an uncovering of the natural progression of a pathologic process, which was not seen until the 1960s because those that would have gone on to develop SIRS never survived long enough.
Conceptually, shock is first a disorder of the bulk transport of oxygen to the body’s tissues. To achieve the goal of adequate tissue perfusion the body must have oxygen available and must be able to carry it in the blood. The first requirement is ensured by a secure airway, sufficient ventilation and oxygenation, and lung parenchyma that will allow the diffusion of oxygen across the alveoli. The second requirement of tissue perfusion is that oxygen gets to the end organ. A blood pressure sufficient to ensure flow to all tissues commensurate to their oxygen needs and an adequate hemoglobin concentration and composition to facilitate the delivery of oxygen are essential. Although seemingly simplistic, a thorough grasp of this concept allows systematic identification of the underlying source of shock. This is the typical cardiopulmonary understanding of shock. Yet, recent scientific advances make it dear that shock is no longer simply concerned with the bulk transport of oxygen, fluid status, and gas exchange. The biochemical component of systemic inflammation is just as important, and needs to be taken into consideration in clinical settings. As with many other systems in the body, the immune system relies on complex regulatory mechanisms to ensure proper function. The destructive nature of the body’s defenses must be harnessed to protect against autoimmune reactions and destruction of healthy tissue, while still maintaining the ability to eliminate foreign pathogens. For example, proinflammatory cytokines interleukin (IL)-1, IL-2, and IL-6 must be held in check by IL-10 and other anti-inflammatory molecules. It is this very balance of stimulation and inhibition that is thought to be lost in shock and its massive inflammatory component. The activation of endothelial cells, macrophages, T lymphocytes, neutrophils, and other immune cells by these cytokines leads to massive immunologic stimulation. The resulting inflammation then leads to coagulation abnormalities, increased endothelial permeability, and enzymatic tissue destruction.
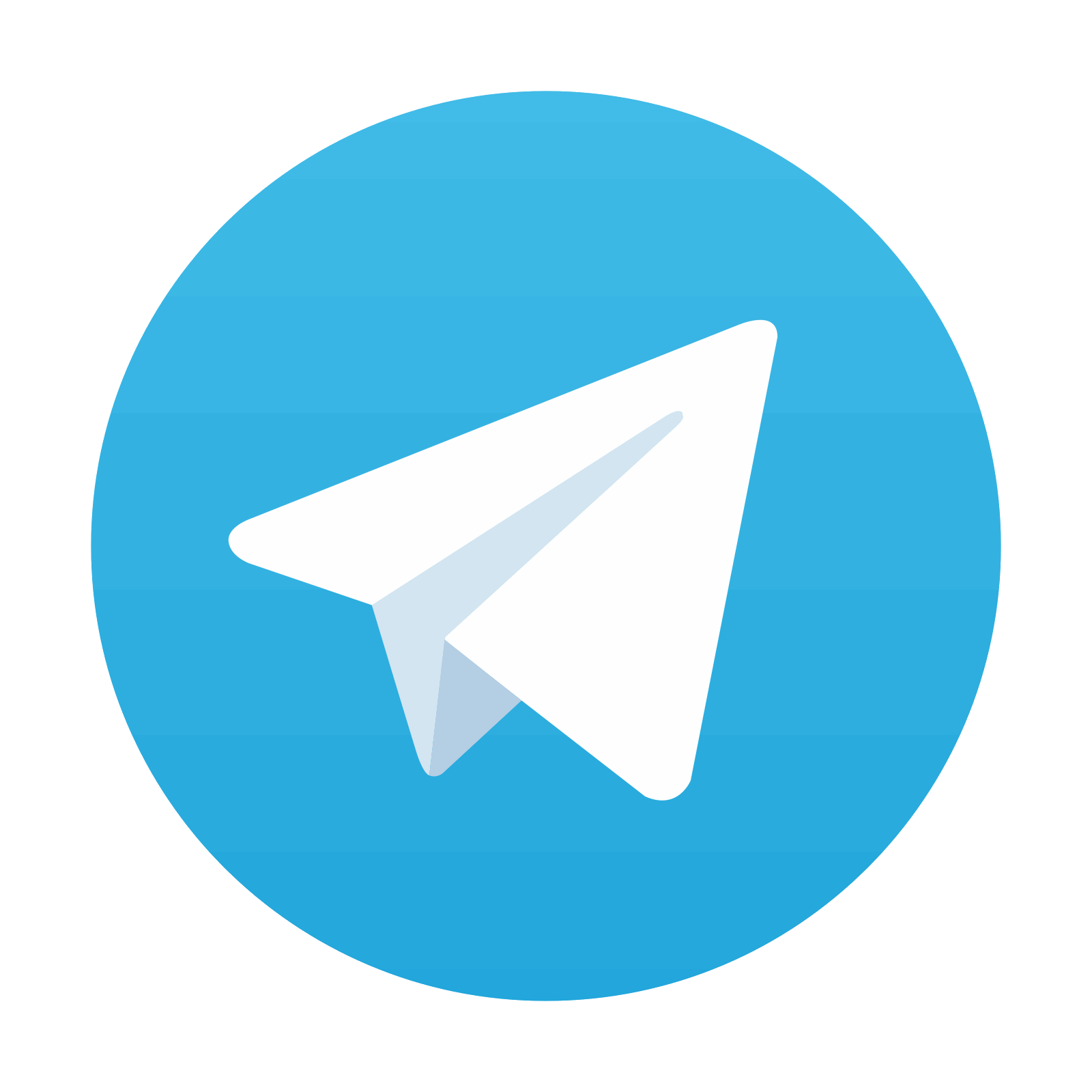
Stay updated, free articles. Join our Telegram channel

Full access? Get Clinical Tree
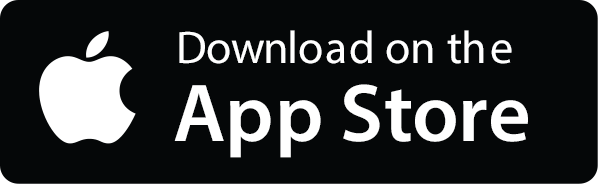
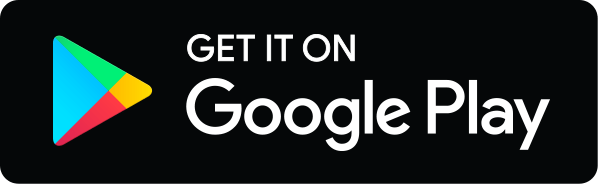