The most common pattern of walking impairment poststroke is hemiparetic gait, which is characterized by asymmetry associated with an extensor synergy pattern of hip extension and adduction, knee extension, and ankle plantar flexion and inversion. There are characteristic changes in the spatiotemporal, kinematic and kinetic parameters, and dynamic electromyography patterns in hemiparesis, which may be assessed most accurately in a motion studies laboratory. An understanding of normal human gait is necessary to assess the complex interplay of motor, sensory, and proprioceptive loss; spasticity; and/or ataxia on hemiparetic gait.
Key points
- •
The most common pattern of walking impairment poststroke is hemiparetic gait.
- •
Hemiparetic gait is characterized by asymmetry associated with an extensor synergy pattern of hip extension and adduction, knee extension, and ankle plantar flexion and inversion.
- •
There are characteristic changes in the spatiotemporal, kinematic and kinetic parameters, and dynamic electromyography (EMG) patterns in hemiparesis, which may be assessed most accurately in a motion studies laboratory.
- •
Increased energy cost of walking is due to the muscle work necessary to lift the body’s center of mass (CM) against gravity during the paretic limb swing phase.
- •
An understanding of normal human gait is necessary to assess the complex interplay of motor, sensory, and proprioceptive loss; spasticity; and/or ataxia on hemiparetic gait.
Introduction
Seventy-five percent of patients who sustain a stroke have limitations in walking, and the most common pattern of walking impairment poststroke is hemiparetic gait. This review provides a comprehensive overview of the characteristic features and analysis of poststroke hemiparetic gait. An understanding of normal human gait, including basic concepts of the neural control of gait and gait cycle terminology, is a prerequisite for analyzing and treating poststroke patients with hemiparetic gait dysfunction. This article also defines spatiotemporal and kinematic parameters of normal gait as well as key changes in these same parameters in hemiparesis. Although patients with poststroke hemiparesis may demonstrate classic changes in spatiotemporal, kinematic, and kinetic parameters, they also demonstrate greater gait variability than neurologically intact controls. More advanced assessments of gait, including kinematic, kinetic, and dynamic electromyography (EMG) analyses, can also be done using quantitative gait analysis. The energy cost of normal walking and the increase in energy costs associated with maintaining walking velocity in poststroke hemiparesis are described. Lastly, the authors assert that good clinical care of patients for poststroke hemiparetic gait does not always require the sophisticated analysis provided by a gait laboratory but instead an astute clinician’s understanding of the complex interplay of motor strength deficits, variable spasticity, sensory and proprioceptive loss, and/or ataxia on motor control and functional gait performance. Thus, a rubric for the clinician to describe hemiparetic gait in the clinical setting is presented.
Introduction
Seventy-five percent of patients who sustain a stroke have limitations in walking, and the most common pattern of walking impairment poststroke is hemiparetic gait. This review provides a comprehensive overview of the characteristic features and analysis of poststroke hemiparetic gait. An understanding of normal human gait, including basic concepts of the neural control of gait and gait cycle terminology, is a prerequisite for analyzing and treating poststroke patients with hemiparetic gait dysfunction. This article also defines spatiotemporal and kinematic parameters of normal gait as well as key changes in these same parameters in hemiparesis. Although patients with poststroke hemiparesis may demonstrate classic changes in spatiotemporal, kinematic, and kinetic parameters, they also demonstrate greater gait variability than neurologically intact controls. More advanced assessments of gait, including kinematic, kinetic, and dynamic electromyography (EMG) analyses, can also be done using quantitative gait analysis. The energy cost of normal walking and the increase in energy costs associated with maintaining walking velocity in poststroke hemiparesis are described. Lastly, the authors assert that good clinical care of patients for poststroke hemiparetic gait does not always require the sophisticated analysis provided by a gait laboratory but instead an astute clinician’s understanding of the complex interplay of motor strength deficits, variable spasticity, sensory and proprioceptive loss, and/or ataxia on motor control and functional gait performance. Thus, a rubric for the clinician to describe hemiparetic gait in the clinical setting is presented.
Neural control of normal human gait
The neural control of human gait is dependent on both spinal and supraspinal mechanisms; thus, neurologically based gait impairment results from an interruption at either level. The concept of a locomotor center located at the level of the spinal cord was originally proposed in 1911 by T.G. Brown. In his research, Brown noted that cats with transected spinal cords and cut dorsal roots were still capable of producing rhythmic alternating contractions in the ankle flexors and extensors. One century later, the term, central pattern generator (CPG) , is now commonly used to describe a spinal locomotor center. CPGs, a term first coined and described in invertebrates and fish, are innate neural networks that are capable of generating self-sustained, rhythmic patterned output, independent of sensory input. Although only indirect evidence exists for human pattern generation, it is generally believed that CPGs, existing within the low thoracic and lumbar regions of the human spinal cord, generate rhythmic patterned outputs largely responsible for control of human locomotion. CPGs are capable of responding to multiple afferent inputs, which modulate human gait patterns, and include a complex interplay of supraspinal afferents originating from the brainstem reticular formation, basal ganglia, premotor and motor cortex, and cerebellum as well as sensory afferents from vestibular, visual, and proprioceptive systems. Information regarding limb positioning and kinesthesia is relayed from proprioceptors within tendons, muscles, ligaments, and joints via the medial leminiscus within the dorsal column of the spinal cord. The convergence of spinal reflex pathways and descending pathways on common spinal interneurons are known to be integrative. In human and other primate gait patterns, however, it is believed that supraspinal input may play a greater role in the regulation of gait than in other species, due to the importance and prominence of the corticospinal tract. Supraspinal afferents specifically play a prominent role in the volitional control of gait, such as occurs with change in walking speed, walking direction, or obstacle avoidance. In humans, damage within the central nervous system, secondary to a cerebrovascular accident, ultimately results in an alteration of the normal neural control of gait.
Normal human walking
Normal human walking is characterized by a repetitive sequence of limb motion whereby an ipsilateral lower limb alternately provides support for the contralateral lower limb as the body advances forward. Optimal human walking results in the forward translation of the body, using bipedal support, with maximal symmetry, stability, and energy efficiency. Although there is no specific standard for measurement of poststroke gait symmetry, Patterson and colleagues recommend using a symmetry ratio equation, which includes step length, swing time, and stance time as parameters in the equation. Gait stability may be defined as the body’s ability to remain safely in an upright posture with minimal susceptibility to perturbations during walking. Stability during walking is determined by a functional balance between the alignment of the body and the muscle activity at each lower limb joint. Gait stability may be influenced by 3 important factors: (1) the generally disproportionate amount of total body weight that relies on the bilateral lower limbs for support, (2) the multisegmented nature of the 2 supporting lower limbs, and (3) the unique mechanical characteristics of each of the lower limb joints. Poststroke gait stability is particularly important due to an increased risk of falls after stroke. Lastly, the efficiency of walking is defined as the ratio of the work accomplished to the energy expended. At a basic level, the cyclic movement of the limbs that characterizes walking requires energy for muscle contraction and thus any disruption to the normal gait cycle, due to alterations in the intensity or pattern of muscle activation, can result in an increase in the energy expenditure of walking.
Normal human gait cycle
Spatiotemporal Parameters of Gait
The human gait cycle is defined as the temporal sequence of events, which begins at heel strike of an ipsilateral lower limb and ends at the subsequent heel strike of that same limb. The term, stride , is used interchangeably with the term, gait cycle , and thus the term, stride length , refers to the distance to complete 1 gait cycle. The term, step length , refers to the distance between heel strike of an ipsilateral limb to heel strike of the contralateral limb. Cadence is defined as the number of steps per unit time (minute). Walking speed , distance per unit time, is the most important predictor of ambulation status after stroke, and the efficacy of any rehabilitation intervention is most commonly assessed by change in comfortable or self-selected walking speed.
The gait cycle is characterized by alternating single-support and double-support periods . During a single-support period, only 1 lower limb is in contact with the ground. During a double-support period, both lower limbs are concurrently in contact with the ground. In normal walking, double-support periods occur twice during 1 gait cycle. The first occurs when the ipsilateral limb is at heel-strike and the contralateral limb is at toe off (early gait cycle of ipsilateral limb) and the second occurs when the ipsilateral limb is at toe off and the contralateral limb is at heel strike (midgait cycle of ipsilateral limb). Double-support time decreases as walking speed increases and is eliminated altogether during running. During normal walking, each lower limb spends approximately 80% of the gait cycle in single support and 20% of the gait cycle in double support. More sophisticated gait analysis is required to measure single- and double-support time as well as step width, all of which are parameters indicative of gait symmetry and stability.
The gait cycle is divided into 2 primary phases, called the stance phase and the swing phase . Stance phase is defined as the period of time during which the ipsilateral lower limb is in direct contact with the ground. During normal walking, the stance phase comprises approximately the first 60% of the gait cycle. Stance phase is further divided into the following periods: (1) initial contact of the limb (heel strike) or early stance, (2) midstance (weight shifting from heel strike anteriorly over a flat foot), (3) terminal stance (weight shifting anteriorly from flat foot to forefoot), and (4) preswing (heel rise to toe off). Swing phase is defined as the period of time that the ipsilateral lower limb is advancing forward while in the air (ie, not bearing weight). The swing phase comprises the latter 40% of the gait cycle and is further divided into the following periods: (1) initial swing (immediately after toe off), (2) midswing, and (3) terminal swing (immediately prior to heel strike). Ankle plantar flexion at preswing, hip flexion, and forward inertia of the tibia are the primary determinants of swing onset. The specific duration of each gait cycle phase has an inverse relationship to walking speed. Thus, when walking speed increases, the durations of both the stance and swing periods of the normal gait cycle decrease. Fig. 1 demonstrates the normal human gait cycle.
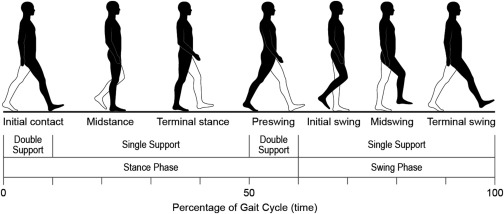
Kinematics and Kinetics of Gait
Although a full discussion of segmental lower limb movement throughout the gait cycle falls outside the scope of this review, a basic understanding of normal pelvis, hip, knee, and ankle joint movements through stance and swing phases is specifically important when considering kinematic and kinetic changes in hemiparetic gait. Kinematics provides a description of joint angles and movement without specific consideration to the forces acting at each joint. Kinetics describes the forces, moments, and mechanical energy that act on each joint during ambulation. For the purposes of this review, primarily the hip, knee, and ankle joint angles in the sagittal plane are focused on.
Although hip angle is commonly believed to define the relationship between the thigh and the vertical, pelvic tilt significantly influences the arc of hip motion created by displacement of the thigh. At heel strike, the ipsilateral hip is in a flexed position of approximately 20°. The hip then begins a progressive movement arc from flexion to extension as the limb transitions through stance with the normal arc of hip motion in stance being approximately 40°. At preswing, the hip begins a gradual reverse progression of hip extension to reach approximately 25° of flexion by terminal swing. Thus, the hip moves through 2 arcs of motion during 1 gait cycle.
Movement of the knee in the sagittal plane (knee flexion and extension) is essential for lower limb advancement. In normal walking, the knee is generally flexed approximately 5° at heel strike and provides shock absorption at limb loading in early stance. Through midstance, the knee gradually extends until nearly fully extended and provides stability for weight bearing throughout stance. By preswing, the knee flexes to approximately 40° and reaches maximal knee flexion at early swing, which helps facilitate forward advancement of the lower limb and toe clearance. In normal walking, 60° of ipsilateral knee flexion are needed to achieve toe clearance.
At the ankle, there are 2 critical periods of ankle plantar flexion, which occur at early stance after heel strike and at preswing prior to toe off. An important transition at the ankle is the gradual dorsiflexion that occurs from midstance to just prior to heel rise. This period of increasing dorsiflexion, during the single-support interval of stance, is a primary determinant of ipsilateral tibial progression. The gastroc-soleus complex stabilizes the ankle at heel rise. During swing, the ankle dorsiflexion muscles contract to realign the ankle to neutral for toe clearance.
Muscle activation during the gait cycle
Muscles function to provide stability at the pelvis, hip, knee, and ankle, through coordinated patterns of muscle activation, which are phasic and well described in normal gait. The pattern of contraction of any given muscle may be concentric (muscle shortening), isometric (muscle contraction without change in length), or eccentric (muscle lengthening), depending on the demands of the gait cycle phase. Alterations in the normal activation and termination patterns of muscle fiber activity, including early activation, prolonged duration, delayed activity, curtailed activity, absent activity, and cocontraction of agonist and antagonist muscles groups, are common after a neurologic injury, such as stroke. Dynamic EMG recorded while walking may identify abnormalities in neurologic control and muscle weakness as well as compensatory muscle substitutions.
During stance, hip motion transitions from flexion at heel strike and progresses to extension by heel rise. The hip extensor muscles are responsible for limb deceleration at terminal swing (eccentric contraction) and for restraint of forward momentum as the limb is loaded at heel strike. The primary hip extensors are the gluteus maximus muscle and the 3 hamstring muscles (semimembranosis, biceps femoris, and semitendinosis). The role of the hip abductors (gluteus medius and minimus, tensor fasciae latae) is to counter the contralateral pelvic drop during the cyclic intervals of non–weight bearing throughout ipsilateral stance. The primary hip flexors are the iliopsoas and rectus femoris muscles, both of which contract concentrically at early swing. Although there are 14 specific muscles providing stability at the knee during the gait cycle, the quadriceps complex (vastus medialis longus and oblique, vastus intermedius, and vastus lateralis) is the dominant muscle group for knee extension. Stance stability is also largely reliant on the soleus muscle to control the forward motion of the tibia, iliotibial band tension, and the actions of the rectus femoris, upper portion of the gluteus maximus, and hamstring muscles. Limb deceleration during terminal swing is largely influenced by the balance between the eccentric contraction of the hamstring muscles and the concentric contraction of the quadriceps muscles. The tibialis anterior muscle is the primary ankle dorsiflexor, contracting eccentrically during stance phase (heel strike to foot flat) and concentrically during swing phase. Lastly, the gastroc-soleus complex is the primary ankle plantar flexor, concentrically contracting during terminal stance. Fig. 2 presents the pattern of lower muscle activation during the normal human gait cycle.
