Fig. 2.1
Diagram of bone metabolism remodeling in response to stress. Top: Balance between factors that contribute to microdamage accumulation and variables that affect the body’s natural healing response. Bottom: Spectrum of bone remodeling. As rate of demands outweight the body’s healing response, further damages increase risk of stress injury and complete fracture
Timing to allow an athlete to return to play is among the most challenging decisions for practitioners. Factors to take into account include duration and progression of symptoms, location of injury and its propensity for fracture completion, risks of nonunion or consequences of complete fracture, timing in season of sport, as well as proposed treatment plan and rehabilitation. Overtreatment of low-risk stress fractures may lead to athlete deconditioning and need for increased duration of rehabilitation. Conversely, some high-risk fracture patterns have significant risk of nonunion or progression to complete fracture and may be more amenable to surgical fixation in order to allow faster union and return to activity [1]. Nonoperative treatment protocols for lower extremity stress fractures may range from non-weight bearing with crutches, allowing aerobic cross-training without impact activities, or avoidance of only pain-provoking activities. With careful attention, a significant proportion of stress fractures that arise in athletes may be successfully treated with aggressive nonoperative management and the patient and practitioner may be able to avoid unnecessary surgical intervention and risk.
Initial Evaluation
Once a stress fracture has been identified in an athlete, it is prudent for the practitioner to identify possible contributing factors for why the injury occurred, both to allow the fracture to heal as well as to prevent recurrence. These risk factors may be divided into extrinsic or intrinsic variables. Extrinsic factors are defined by the training environment and include training duration and intensity, exercise surface and footwear, and nutritional status. Intrinsic factors are specific to the patient and include anatomic variations (femoral version, leg length discrepancies, genu valgum/varum, pes planus/cavus), gender, smoking status, medical and endocrinologic history, and medications [2].
It is well known and understood that stress fractures are associated with large increases in intensity and duration of athletic participation or practice over a short period of time. Thus, a history of training and play is critical in the evaluation of the athlete with a stress fracture. The majority of studies evaluating stress fractures stem from individuals participating in military boot camp—likely due to the marked increase in duration and intensity of training during the initiation of military duty. Additionally, an informal study performed in 1999 on college athletes at an NCAA Division 1 program found that 50 % of stress fractures occurred in freshman athletes. This is also likely due to the significant increase in the duration and intensity of training at the collegiate level, as compared to the high school level.
In conjunction with treatment, a diagnostic evaluation is recommended to rule out any metabolic pathology that would increase an athlete’s risk for developing a fracture or delay healing [3]. A careful history and physical examination is necessary to evaluate for eating disorders, smoking and alcohol abuse, hormonal imbalance, sexual dysfunction, thyroid disorder, and menstrual irregularities. Laboratory work-up includes urinalysis, complete metabolic panel with analysis of serum calcium, albumin, alkaline phosphatase, and serum vitamin D levels [3]. DEXA (dual-energy X-ray absorptiometry) scan may be considered in patients with multiple or recurrent stress injuries.
Of special consideration in female patients is the “Female Athletic Triad,” traditionally defined as the inter-related combination of eating disorders, amenorrhea, and osteoporosis [4]. However, a new description in 2014 by the Female Athlete Triad Coalition describes the constellation of “Low Energy Availability” with or without disordered eating, menstrual dysfunction, and low bone mineral density as another definition that places female athletes at risk for amenorrhea, osteoporosis, and stress fractures [4, 5]. Circulating estrogens have been shown to increase bone marrow density through decreased calcium resorption by osteoclasts. Patients with Female Athletic Triad and amenorrhea are thought to subsist in a lower estrogen state with resultant osteopenia and increased risk for stress fractures. Treatment focuses on adjusting caloric intake and reducing activity to support an overall anabolic metabolic rate [4]. If the Female Athlete Triad with amenorrhea is suspected, treatment should be initiated consisting of evaluation by a multidisciplinary team including physicians, sports dietitians, and mental health professionals.
Lloyd et al. retrospectively reviewed the relationship of menstrual status and stress fractures in collegiate women athletes. The authors found the frequency of stress fractures in women with irregular menses (15 %) was nearly four times that of female athletes who had regular menses (4 %) [6]. Similarly, Barrow et al. stratified the prevalence of stress fractures in competitive collegiate long distance runners according to regularity of menstrual cycles. There was an inverse relationship with menstrual consistency and injury; stress fractures occurred in 29 % of athletes who had 10–13 menses/per year, 39 % of athletes who had 6–9 menses/year, and 49 % of athletes who had 0–5 menses/year [7]. Additionally, 47 % of those who had less than 5 menses per year admitted to an eating behavior disorder.
In order to further evaluate the contributions of hormonal therapy, Cobb et al. randomized 150 female runners between 18 and 26 years of age to treatment with oral contraceptive (30 mcg of ethinyl estradiol and 0.3 mg of norgestrel) or no treatment [8]. After 2 years, the authors found a nonsignificant trend towards decreased stress fracture incidence with oral contraceptive use; the authors also did report a high noncompliance rate in the treatment group. Further studies are needed to elucidate the interaction between hormonal status and stress fractures incidence. Certainly in the female athlete, identifying those at risk for Female Athletic Triad/Low Energy Availability is crucial and may prevent further progression of serious health consequences.
In addition to hormonal factors, lifestyle choices may place athletes at risk for further injury. A study of female military recruits entering basic training found those who reported alcohol intake of more than ten drinks per week, smoking history, and exercising fewer than three times per week prior to start of basic training had increased risks of stress fractures [9].
Extrinsic Factors
One population that has been studied extensively in evaluation of both risk factors and treatment efficacy of stress fractures are military personnel. These typically are relatively young patients subjected to standardized rigorous activity requirements in multiple intervals per year, often with sudden increase in activity level from baseline. This demographic of patients is at high risk for stress fractures, with some reports of up to 31 % incidence and the majority occurring in either the femoral or tibial shaft [10]. Milgrom et al., in a study of Israeli military recruits, randomized 390 recruits to 14 weeks of basic training in either standard infantry boots or high-top basketball shoes to evaluate the role of shoe design and cushioning. The authors found lower incidence of metatarsal stress fractures and foot overuse injuries (metatarsalgia and arch or heel pain) in those using basketball shoes but no difference in overall overuse injuries. Additionally, no differences in the incidence of tibial or femoral stress fractures were identified [11]. The same group also evaluated the effect of a cushioning shoe insert in a prospective study of military recruits treated with or without shock-absorbing orthotic worn in military boots [12]. There was a significant decrease in femoral stress fractures with the use of orthotics but this was not seen in metatarsal or tibial stress fractures. No differences were found in time to onset or location of stress fractures. With this data, Milgrom et al. randomized 404 recruits to three groups: group 1 was given custom semirigid orthotics with army boot, group 2 was given soft orthotics with army boot, and group 3 received only army boots [13]. All military boots used in this study had soles designed like basketball shoes. After 14 weeks of basic training, the femoral and tibial stress fracture rate was 15.7 % for those in semirigid group, 10.7 % for soft orthotic group, and 27 % for the control group. When comparing the patients who received any orthotic (semirigid or soft) to those without, the authors found a significant decrease in total stress fracture rate with orthotic use. No differences in stress fracture rate were identified between the two types of orthotics. However, the analysis was limited by dropout rate of nearly 50 %, as many recruits preferred their own custom orthotics that they were using before the military. The authors recommended the use of orthotic inserts in the military population for prophylaxis of stress fractures. These findings have been corroborated in other clinical series of military recruits, with either orthotic use or modified shoe type reducing stress fracture incidence [14–17].
In addition to footwear characteristics, training surface has also been thought to play a role in the development of stress fractures. Current studies, though, have not demonstrated an association between type of training surface and incidence of stress fractures. Only limited data exists to associate running on concrete surfaces with lower extremity injuries [18]. Evidence is poor due to methodology and sampling bias, but some investigators recommend considering minimizing time on hard, uneven surfaces based upon intuitive reasoning [18–22].
Swissa et al. evaluated the role of pretraining in reducing the risk of stress fractures in military recruits. Their hypothesis was that recruits with increased levels of impact activity prior to basic training had decreased stress fractures. The authors prospectively evaluated 295 recruits and found 78 % were involved in some sort of sport activity prior to basic activity. No correlation was found between those who participated in pretraining sport activity and incidence of stress fractures, averaging 31 % for trained and untrained groups [23].
Intrinsic Variables
Individual anatomic variables have also been investigated as possible risk factors for stress fractures and may serve as potential targets for prevention. Milgrom et al. prospectively evaluated the role of midfoot position and stress fractures and found increased prevalence of femoral and tibial stress fractures in military recruits with pes cavus. However, metatarsal stress fractures were more frequently seen in those with pes planus [14]. The authors suggest that a high arch is a more rigid position and has decreased ability to absorb shock, as opposed to a more flexible low-arched foot [19]. Other studies have confirmed the theory that rigid pes cavus is more common in patients with femoral stress fractures. Additionally, excessive foot pronation position was more commonly seen in patients with stress fractures of tibia and fibula, thought to be related to increased tibial torsion during the support phase of running [24].
Leg length discrepancy has also been associated with increased risk of stress fractures [22]. Surveys of distance runners revealed leg length discrepancies are a major contributing factor to running injuries [22]. The longer leg was associated more frequently with tibial, metatarsal, and femur fractures. Incidence of stress fractures has been shown to increase with increasing magnitude of leg length discrepancy [19, 25]. Bennell et al. prospectively evaluated 53 female athletes and found leg length discrepancy of more than 0.5 cm was seen more frequently in those who sustained stress fractures (70 % vs. 36 %). Fractures occurred with similar frequency in the longer and shorter limb [26]. The authors also noted athletes who had decreased calf muscle mass had higher rates of stress fractures, suggesting calf muscles strength may protect bone from repetitive excessive force.
Treatment Modalities
Activity Modification
Often the first step in the management of stress fractures includes adjusting an athlete’s activity below a threshold that the body’s reparative process can keep up with. Patient reported pain is useful as a proxy for “too much” activity. However, the maintenance of cardiovascular fitness is crucial for an athlete and may be preserved through aerobic activities that limit weight bearing and impact, including cycling (with or with an immobilizing device), upper extremity cycling (ergometry) in the presence of a lower extremity stress fracture, pool exercises, or weight unloading training in harnesses or decreased gravity treadmills (Fig. 2.2). Some reports found distance runners have improved stamina after cross-training in a pool as opposed to other low-impact modalities [27]. It may take up to 6–8 weeks for healing of stress fractures to occur [28].
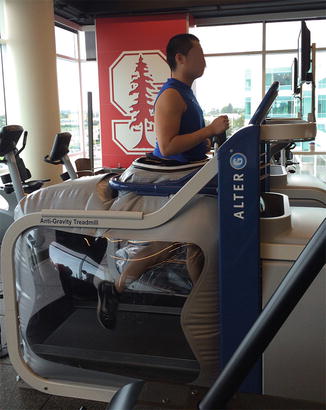
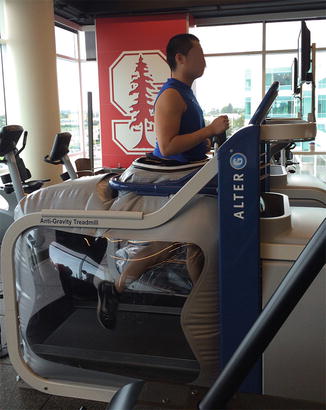
Fig. 2.2
Gravity-reduction treadmill
Matheson et al. reported on a series of 320 patients with stress fractures identified by radiographs and bone scan [24]. The athletes were initially treated with nonsteroidal anti-inflammatories (NSAIDs), symptom control, and activity modification. Athletes were allowed normal weight bearing during day-to-day activities with the avoidance of pain-provoking maneuvers. Cycling, swimming, and running in water were allowed as low-impact alternatives. After athletes were pain free for 10–14 days, gradual reintroduction of sport was allowed. No patients treated in the series progressed to complete fracture and the majority of athletes were able to return to activity with excellent results. The authors concluded the effectiveness of conservative treatment for the treatment of most stress fractures.
External bracing of the lower extremity has also been evaluated as a treatment adjunct for the management of stress fractures. Dale et al. described the contribution of pneumatic braces on fractures in a canine model [29]. Animals were treated with tibial osteotomy and external fixator placement and allowed unrestricted weight bearing for 48 days to simulate partial healing. External fixators were then removed and the subjects were divided into groups treated with either standard cast or pneumatic brace. There was significantly greater torque to failure, energy absorbed, and angle of rotation for those treated with pressurized brace. Histologic studies also demonstrated increased periosteal bone formation and greater bone density at fracture site in treatment group as compared to standard group. Human studies have similarly found a positive benefit to the use of such braces. Swensen et al. randomized 18 athletes with tibial shaft stress fracture to examine recovery after use of pneumatic leg brace. The control group was treated with rest and allowed return to activity once had they had three consecutive pain-free days. The treatment group had pneumatic leg brace applied and followed the same return to play guidelines. Athletes receiving the brace demonstrated earlier return to light activity and accelerated return to full activity (21 vs. 77 days) [30]. The authors proposed that compressing the soft tissue may increase intravascular hydrostatic pressure and produce downstream effects that stimulate osteoblast bone formation.
A recent Cochrane review evaluated the effect of pneumatic braces for stress fractures and showed a significant reduction in time before returning to full activity (Fig. 2.3) [31]. When evaluating trials in aggregate, the mean acceleration of healing measured approximately 33 days when the braces where used.
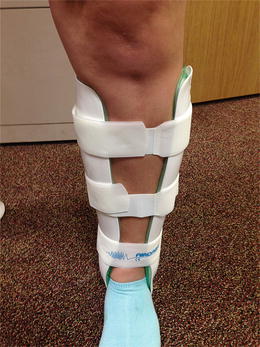
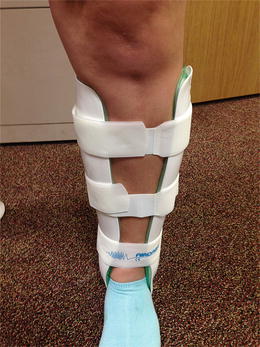
Fig. 2.3
Pneumatic compression ankle brace
Pharmacologic Therapies
A variety of pharmacologics have been used in order to both treat and prevent stress fractures [2]. Much of the literature stems originally from the treatment of acute fractures with further studies that have been focused on stress fractures and its applicability to the athletic population [32].
Vitamins
Calcium and vitamin D have long been understood as essential vitamins in the maintenance of bone health. Calcium is a significant component in the inorganic substrate of bone. Vitamin D is obtained from diet, sunlight, or supplements and facilitates intestinal absorption of calcium [33]. Vitamin D is metabolized into 1,25-dihydroxyvitamin D, which serves as an active metabolite to enhance intestinal mucosal calcium absorption via active calcium transporters in the mucosal epithelium [34]. In the elderly, oral vitamin D has been shown to reduce risks of insufficiency fractures in randomized trials [35]. In the setting of stress fractures, the addition of oral calcium and vitamin D are advocated if laboratory values are low [3]. Recommended daily allowances are 1,000 mg of calcium and 800–1,000 IU of vitamin D. However, some authors recommended higher doses for athletes with stress fractures, up to 1,500 mg calcium and 3,000 IU of vitamin D [3, 34].
Nieves et al. followed 125 female distance runners between 18 and 26 years of age and found stress fracture incidence was reduced with increased baseline intake of dietary calcium and dairy products. The effective fracture risk decreased by 62 % with each additional cup of skim milk per day. Women who consumed less than 800 mg of calcium per day had six times the stress fracture rate than those who consumed more than 1,500 mg per day [36, 37]. Lappe et al. randomized 5,201 female Navy recruits to either treatment with 2,000 mg calcium and 800 IU vitamin D or placebo. A total of 309 stress fractures were diagnosed (5.9 %) over an 8-week period. Recruits who were treated with supplementation had a 20 % lower incidence of stress fractures (6.6 % vs. 5.3 % in intent-to-treat analysis, 8.6 % vs. 6.8 % in as-treated analysis). The authors stated that if the entire population of 14,416 women who entered basic training in the 2-year recruitment period were treated with vitamin supplementation, 187 stress fractures would have been prevented [37, 38].
NSAIDs
NSAIDs are commonly used medications to treat musculoskeletal disease. Their therapeutic effects are achieved via inhibition of cyclooxygenases (COX), enzymes that participate in the pro-inflammatory response. NSAIDs ultimately reduce the production of prostaglandins, whose downstream effects include pain, swelling, and other inflammatory symptoms manifested through histamine and bradykinin release [39, 40]. During the cascade of fracture healing, COX enzymes are active at injury sites to produce prostaglandins. These chemicals are released by both local osteoblasts and osteoclasts to serve as potent stimulators of bone turnover. Bone formation and resorption, as well as local angiogenesis, support cell metabolic activity along the pathways of endochondral and intramembranous ossification [41]. There is concern, though, that use of NSAIDs may inhibit fracture healing and increase nonunion rates.
Wheeler et al. reviewed the interactions of NSAIDs on bone healing. Multiple animal studies have shown NSAIDs (including ibuprofen and indomethacin) may retard the rate of fracture healing, decrease fracture callus strength, and may lead to increased nonunion rates [42]. Other theories surmise NSAIDs may inhibit differentiation of mesenchymal stem cells into chondrocytes and osteoblasts [42]. Kidd et al. evaluated the effect of NSAIDs on stress fractures in an animal model. Stress fractures were created via cyclic loading of rat ulnas. The animals were then treated with ibuprofen 30 mg/kg/day or control and examined at 2, 4, and 6 weeks. Use of ibuprofen decreased both resorption and bone formation after 6 weeks when measured via histomorphometry [43].
Despite the detrimental consequences of NSAIDs on fracture healing in animal studies, human studies have not clearly demonstrated the same effects. The majority of clinical studies are retrospective with assorted results, finding either small association or no difference with NSAID use [42]. Only two randomized studies exist evaluating the role of NSAIDs in fracture healing. Adolphson et al. randomized 42 patients with distal radius fractures to either treatment with piroxicam 20 mg/day for 8 weeks or placebo. All patients were treated nonoperatively with dorsal plaster splints for 4 weeks. At final 8-week follow-up, no difference in bone-mineral density, rate of fracture healing, radiographic findings, or functional recovery was found between the two groups. Patients receiving piroxicam did demonstrate decreased pain scores in the early follow-up paper. However, six patients, all in the piroxicam group, had loss of reduction and required external fixation for stability and were excluded from final analysis [44]. Burd et al. evaluated the role of NSAIDs in 282 patients who had open reduction and internal fixation of an acetabular fracture [45]. Patients at risk of heterotopic ossification were randomized to receive either radiation or indomethacin (25 mg three times a day for 6 weeks) for prophylaxis. The authors found that patients who received indomethacin had increased rates of nonunion for associated long bone fracture (26 % vs. 7 %). However, the two groups were randomized according to their acetabular surgery and differed in injury severity scores and rates of open fracture. Concerns with randomization protocol, data collection outcomes, and methodological deficiencies exist in current clinical studies and limit the ability to draw conclusions regarding therapeutic effect of NSAIDs on fracture healing. Currently, there are no randomized studies of NSAIDs in the healing of stress fractures, and extrapolation of results from acute traumatic fractures may not be fully applicable given differing clinical scenarios. Further large, prospective studies with careful attention to methodology are required to better define the role of NSAIDs and eventually guide physician decision making.
Bisphosphonates
Bisphosphonates have been used to treat many different bone diseases caused by excessive osteoclast activity. Its mechanism of action is through suppression of bone resorption through suppression of osteoclast activity, accomplished via inhibiting farnesyl pyrophosphate synthase. Isoprenylation of GTP-ases is interrupted to interfere with the attachment of osteoclasts to the bone surface and promotion of osteoclast cell apoptosis [46]. Significant evidence for bisphosphonates has been published in the literature regarding its ability to prevent of postmenopausal fragility fractures. Large randomized trials demonstrate decreased incidence of new vertebral compression fractures and hip fractures in elderly females prescribed bisphosphonate therapy [46–49].
The role of bisphosphonates in bony healing in the setting of acute fractures has been investigated as well. Adolphson et al. performed a randomized, double-blinded study in postmenopausal females with distal radius fractures. Patients either received 500 mg of clodronate twice a day for 8 weeks or placebo. At 2-month follow-up, those treated with clodronate had a greater ratio of bone-mineral density at the fracture site when compared to contralateral side (53 % vs. 33 %). At 12-month follow-up, effective increased bone density at the fracture site was still maintained. There were no differences in pain or clinical function scores at 3-month follow-up [50].
Despite these findings, convincing clinical improvements for the use of bisphosphonates in stress fractures have not yet been clearly borne out in the literature. Stewart et al. treated five collegiate female athletes 18–24 years old with tibial stress fractures with pamidronate, a second generation bisphosphonate [51]. Patients received intravenous infusions of pamidronate, comprised of five doses of 60–90 mg administered on a weekly basis. Four of five patients were able to achieve primary outcomes of ability to continue training without pain. All five athletes reported improvement in pain after the first dose. No improvement in outcome was found between the 60- and 90-mg dosing, with 90 mg having a higher rate of nausea as an unintended side effect. Though results are encouraging, further prospective studies with comparison groups are needed.
Milgrom et al. randomized 324 military recruits to treatment with risedronate or placebo in order to evaluate stress fracture prophylaxis. Patients in the treatment arm were given 30-mg oral dose of risedronate for 10 days as a loading dose during the first 2 weeks of basic training. They then continued 30 mg by mouth every week for the next 12 weeks. The authors found no differences in stress fracture incidence between groups using either intention-to-treat analysis or an as-treated comparison. Total incidence of stress fractures was 14.5 % in the risedronate group and 13.2 % in the placebo group. No significant differences in side effects were found between the two groups [52].
Use of bisphosphonate treatment is not without risk. Recent evidence associates long-term bisphosphonate use with atypical femur fractures. These typically occur in the subtrochanteric or diaphyseal femur after low-energy trauma, thought to be due abnormal bone remodeling due to osteoclast inhibition, resulting in accumulation of microfractures [53]. Bisphosphonates have been shown to decrease bone toughness in animal studies, resulting in increased brittleness and decreased ability to absorb energy prior to fracture [54, 55]. Canine studies have also shown that bisphosphonates, by suppressing the body’s natural bone remodeling process, leads to the accumulation of microdamages in bone [55–58]. Caution should especially be noted with use of bisphosphonates in young women of child-bearing age. Animal studies have shown bisphosphonates to cross the placenta and decrease fetal weight, affect bone growth, and may be associated with increased neonatal deaths. As bisphosphonates are stored in bone and slowly released, they may have circulating half-life of over ten years [59]. Risks for nonunion must be carefully weighed against future teratogenic complications; many authors suggest withholding bisphosphonate treatment in young females.
Forteo (Teriparatide)
Teriparatide is a synthetic parathyroid hormone (PTH) approved in 2002 for treatment of osteoporosis. PTH functions to maintain calcium and phosphate homeostasis via increasing tubular resorption in the kidneys, increasing renal production 1,25-dihydroxyvitamin D, and by mobilizing calcium from bone stores [60]. Though originally understood to conserve calcium in the body, PTH has more recently been discovered to have anabolic effects for bone and have receptors located on osteoblasts. Animal studies demonstrated administration of PTH in a continuous infusion resulted in decreased body weight, hypercalcemia, and peritrabecular marrow fibrosis and resorption. However, administration in a pulsatile manner increased anabolic effects and ultimately results in osteoblast proliferation and bone formation [61]. Other animal studies demonstrate PTH role in the activation of resting osteoblasts and stimulating osteoprogenitor cells [32].
Teriparatide was initially approved after randomized trials in osteoporotic women revealed it increased bone marrow density by up to 13 % and decreased risk of vertebral and nonvertebral fractures by at least 50 % to 72 % [62]. Neer et al. published a randomized, blinded trial treating 1,637 postmenopausal women with low-dose PTH (20 mcg administered subcutaneously daily), high-dose PTH (40 mcg administered subcutaneously daily), or placebo. Patients in both treatment groups had decreased rates of new vertebral fractures, nonvertebral fragility fractures, and increased bone marrow density [62]. The medication was well tolerated by patients with only minor side effects of nausea and headache.
The use of synthetic PTH has been evaluated for the treatment of acute fractures as well. Komatsubara et al. evaluated femoral osteotomies performed in a rat model and treated subjects with various dosing regimens of PTH or control [63]. Treatment was administered via subcutaneous injections three times a week for 3 weeks. The authors found treatment at 30 mcg/kg administered both before and after osteotomy increased the mechanical strength of callus through faster remodeling of woven bone to lamellar bone, increased cortical shell formation, and stronger ultimate load to failure at 12-week follow-up. Accelerated appearance of the cortical shell was likely the main contributor of increased mechanical strength. The same group also investigated PTH in a monkey model treated with femoral osteotomy and stabilized with plate fixation [64]. Subjects were randomized to control, low-dose or high-dose PTH via twice a week subcutaneous injection for 3 weeks. At 6-month follow-up, the authors found acceleration of the body’s natural healing process in a dose-dependent manner. Increased ultimate stress and elastic modulus was seen for the high-dose treatment group. The authors also noted decreased callus area and increased callus mineralization, resulting in stronger intrinsic material properties such as ultimate stress, elastic modulus, and toughness. No differences were found in ultimate load, stiffness, load to failure. Andreassen et al. found PTH increased callus formation, strength, mineralization, and mechanical strength in rat tibia fracture model [65, 66]. Other animal studies have demonstrated similar findings of accelerated rate of normal fracture healing, resulting in increased callus strength at comparable time points [67].
Peichl et al. performed one of the first human clinical trials evaluating the effect of PTH in acute fractures and demonstrated accelerated fracture healing [68]. Sixty-five postmenopausal female patients who sustained unilateral fractures of the pubic bone or ischial rami with T-score less than −2.5 were included. Patients were randomized to treatment with either placebo or daily injections of 100 mcg of PTH. All patients received calcium and vitamin D supplementation. Patients were evaluated with CT scans every fourth week for evaluation of bony bridging. The authors found significantly accelerated healing with earlier bony bridging in the treatment group (7.8 vs. 12.6 weeks). After 8 weeks from injury, 100 % of fractures in the treatment groups had healed, as opposed to 9.1 % in control group. Patients allocated to the treatment group also demonstrated improved clinical scores as assessed by VAS pain scores and timed “up and go” test (22.9 vs. 54.3 s). No laboratory abnormalities were noted with treatment. Aspenberg et al. randomized postmenopausal women with dorsally angulated distal radius fractures treated with closed reduction and immobilization to either 8 weeks of daily injections of placebo or teriparatide at 20 or 40 mcg. The authors found shorter time to radiographic healing in the low-dose group but no differences in the high-dose group. There were no differences in fracture displacement or clinical scoring outcomes [69]. The majority of clinical studies evaluating the effect of teriparatide are performed in elderly, osteoporotic populations. No studies are yet published describing the use of PTH in the treatment of stress fractures; it is not yet known whether these effects can be extrapolated to a younger, more active patient population. However, the senior author has been using teriparatide in the management of stress fractures in high-level athletes with anecdotal results of earlier return to play.
Adjunctive Therapies
Low-Intensity Pulsed Ultrasound
Ultrasound functions as propagating pressure waves arising via vibration from a source material, which transfers mechanical pressure to recipient tissue. Though the exact mechanism of action has not been fully elucidated, many studies suggest these waves accelerate the endochondral ossification process through afferent mechanoreceptors and alter cell signaling. Potential changes in blood flow patterns may occur as well [70]. The clinically effective ultrasound signal approved by the United States Food and Drug Administration consists of a 1.5-MHz ultrasound wave pulsed at 20 % duty cycle at an intensity of 30 mW/cm2 [71].
Wolff’s law dictates that bone remodels according to mechanical forces within its environment. Animal studies have demonstrated the effect of ultrasound waves as an external, mechanical stimulus to bone growth. Yang et al. used a rat femur-fracture model and found greater maximum torque and torsional stiffness at 3 weeks after injury in subjects treated with pulsed ultrasound [72]. Biochemical analysis demonstrated increased expression of genes associated with cartilage formation, but no differences in cell number or mineral content. The authors suggested this may due to accelerated chondrogenesis and endochondral ossification. Similar findings have been seen in other animal studies, where groups receiving ultrasound therapy demonstrated increased torque to failure and stiffness [73].
Kristiansen et al. performed a prospective multicenter trial that randomized 60 patients with dorsally angulated distal radius fractures treated nonoperatively with either an ultrasound device or placebo for 20 min per day for 10 weeks [70]. The authors found faster time to union by 37 days and accelerated radiographic signs of healing, as well as decreased loss of reduction in patients receiving ultrasound device. Though no adverse reactions or side effects were reported with use of the device, no relevant clinical outcomes were reported. Heckman et al. reported accelerated healing in tibial diaphyseal fracture with the use of a pulsed ultrasound device in a prospective blinded multicenter trial [74]. Sixty-seven patients with tibial shaft fractures were randomized to cast treatment with or without external ultrasound stimulator. Treatment was started within 1 week of injury and used for 20 min per day for 20 weeks or until the fracture healed; placebo groups were given a non-functioning machine. The authors found significant decrease in time to clinical healing (86 vs. 114 days), decreased time in cast (94 vs. 120 days) and decrease in time to overall (clinical plus radiographic) healing (86 vs. 154 days). Patients reported very high compliance with the device. Low-intensity ultrasound also accelerated healing response in smokers and reduced the incidence of delayed unions [75, 76].
Rue et al. randomized 26 midshipmen with tibial stress fractures in a double-blind study to pulsed ultrasound or placebo device. Patients received 20-min daily treatments until they were asymptomatic or signs of healing were noted on plain radiographs. The authors found no differences in total number of treatments needed, days needed to return to duty, or total days of symptoms [77]. The average treatment duration was 24–26 sessions.
A systematic review analyzed 13 randomized trials studying the use of low-intensity pulsed ultrasound in fractures. Only five studies assessed clinically relevant outcomes, with one trial demonstrating a positive effect. Two studies with the best quality evidence demonstrated no difference in functional outcome. Overall there was low-to-moderate quality evidence with conflicting results and most trials only reporting proxy outcomes for clinical benefit [78, 79]. A recent Cochrane review demonstrated no significant difference in time to union as assessed by clinical outcomes. Subgroup analysis reported possible acceleration of nonoperatively treated fractures but overall insufficient data to recommend routine use [80]. The apparent consensus from most meta-analyses concludes that pulsed ultrasound may speed union rates as assessed by radiographs. However, improvements in patient-reported scores or clinical effect have not yet been convincingly reported in the literature [78, 80]. Pulsed ultrasound may have a role in accelerating healing in acute fractures and may be considered decreasing nonunion rate in at risk population, such as smokers and the elderly. Few risks or complications have been associated with its use [76]. The results are promising but need larger trials with more relevant outcomes are needed. At this point no clinically significant effect for low-intensity pulsed ultrasound has been established for stress fractures.
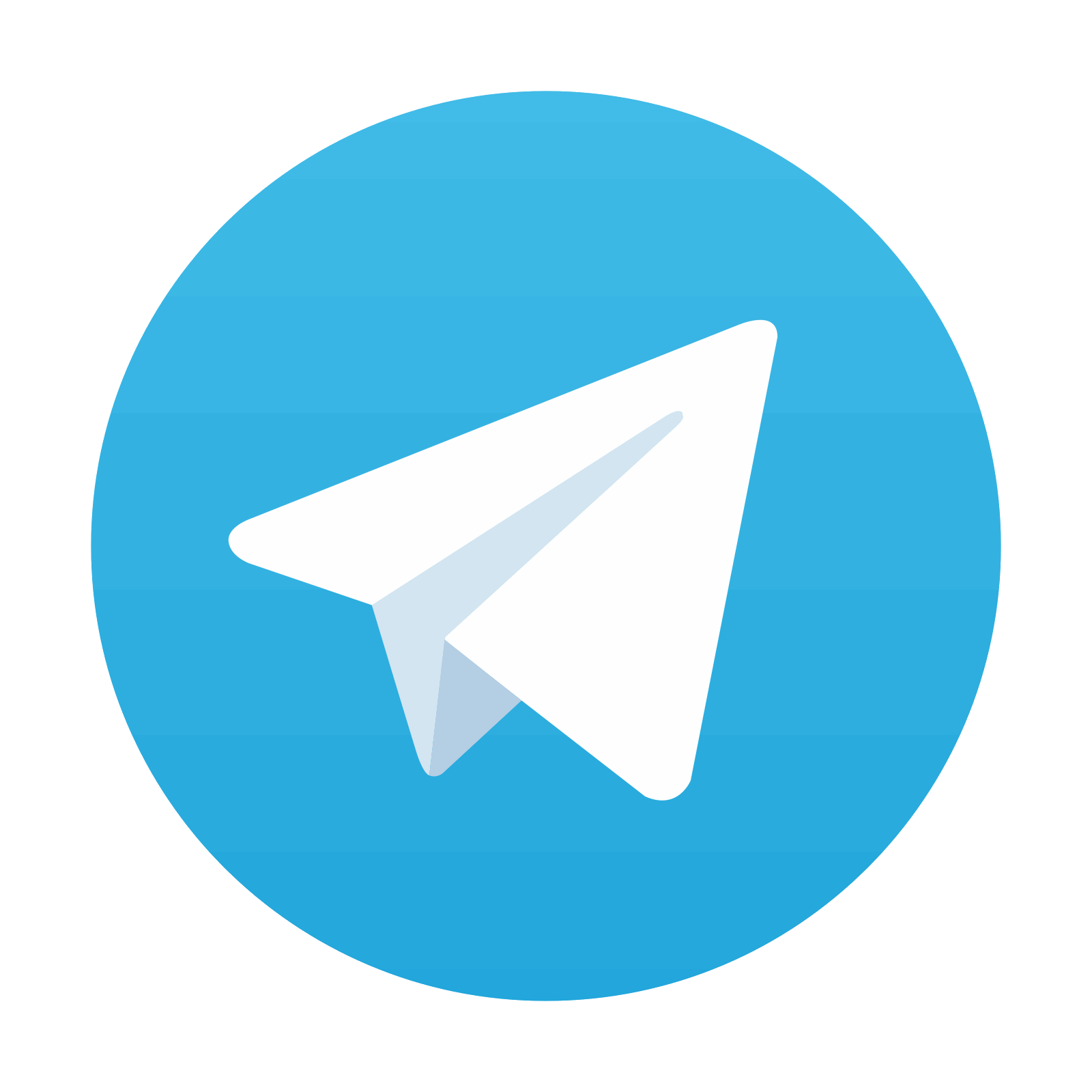
Stay updated, free articles. Join our Telegram channel

Full access? Get Clinical Tree
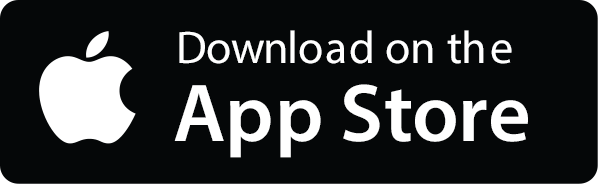
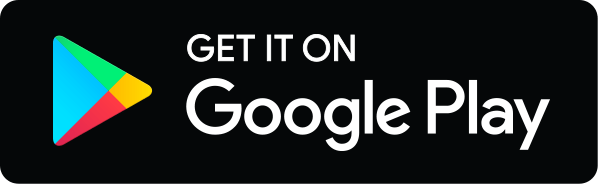