General Considerations of Allergies in Childhood
Hugh A. Sampson
Peyton A. Eggleston
The prevalence of allergic disorders such as asthma, atopic dermatitis, and allergic rhinitis has doubled in the past 25 years. Ironically, during this same time, a virtual explosion has occurred in our knowledge of the immunologic and biochemical mechanisms responsible for allergic disorders. Data support the pathogenic role of allergy in many cases of asthma, allergic rhinitis, atopic dermatitis, urticaria and angioedema, adverse food reactions, drug and biologic agent reactions, and stinging-insect hypersensitivity. Information is available to approach the diagnosis and treatment of these disorders in a rational medical fashion.
Allergy may be defined as any untoward physiologic event caused by an immunologically mediated reaction. This definition has several components that restrict its scope. First, a demonstrable event or disease must occur that is both symptomatic and pathologic, and this event must be related to an antigen or environmental factor that could include airborne pollens, ingested foods, contactants such as latex, industrial chemicals, or parenterally administered drugs. In addition, the disease must have a demonstrable immunologic mechanism and must occur as a result of this immune mechanism. Atopy, a subtype of allergy comprising a constellation of chronic diseases provoked by IgE-mediated mechanisms, has a strong genetic predisposition. Coca and Cooke initially coined the term atopy in 1923, and later suggested that atopy was made up of asthma, allergic rhinitis, and atopic eczema (or atopic dermatitis). However, not all asthma, rhinitis, and eczema are IgE mediated, which results in considerable semantic confusion.
CLASSIFICATION OF ALLERGIC DISEASES
In 1963, Gell and Coombs classified allergic (hypersensitivity) reactions into four types. The first three are antibody mediated and are distinguished by the different type of antigen and antibody class involved. Type IV reactions can be subdivided into three types depending on the class of T cell involved, Th1, Th2, or cytotoxic lymphocyte (CTL). Although somewhat simplistic, this classification is helpful and is referred to frequently. This chapter focuses primarily on type I, IgE-mediated hypersensitivity reactions.
Type I (Anaphylactic Reactions)
Type I reactions are mediated by antigen-specific IgE antibodies. This class of antibody binds to the surface of mast cells, circulating basophilic granulocytes, and certain antigen-presenting cells (e.g., macrophages, dendritic cells, Langerhans cells). The exposure of IgE-coated mast cells and basophils to antigen results in rapid cell activation and the release of a variety of pharmacologically potent mediators and cytokines. The interaction of these mediators with blood vessels, bronchi, or mucus-secreting glands causes disease. Examples of this type of allergy include anaphylactic reactions to insect stings, food-induced urticaria, or allergic rhinitis. The generation of cytokines leads to the more chronic inflammation seen in allergen-induced asthma, allergic rhinitis, and atopic dermatitis.
Type II (Cytotoxic Reactions)
In type II reactions, antibodies of the IgG or IgM class are formed to cell-surface antigens that may be environmental antigens adsorbed to the surface of cells or to self-antigens (as in autoimmune disease). Antibodies bind to the cell and activate complement, which can damage or destroy the cell via the membrane attack complex. Common clinical examples of this type of allergic reaction include drug-induced leukopenia, hemolytic anemia, and thrombocytopenia.
Type III (Arthus or Immune Complex Reactions)
In type III reactions, as in type II responses, IgG and IgM antibodies to an environmental antigen are produced. In this type of reaction, however, the antigen does not bind to cells but circulates in a soluble form. The antigen–antibody complexes that are formed may be small, intermediate, or large. Small complexes may remain harmlessly in the circulation, whereas large complexes are cleared rapidly by the reticuloendothelial system. Intermediate-size complexes, however, may be deposited in vessel walls and tissues. Vascular damage is then initiated by activation of complement, granulocytes, platelets and, probably, basophils. The most common example of this reaction is classic serum sickness.
Type IV (Cell-Mediated Reactions)
Type IV reactions involve antigen-presenting cells (APCs, e.g., monocytes, macrophages, dendritic cells) and one of three types of T lymphocytes with specific receptors for an antigen. After primary exposure, T cells respond to subsequent exposure by proliferation and differentiation into cells capable of causing cytolysis (CTLs), or by recruiting other inflammatory cells; for example, Th1 cells attract and activate macrophages, and Th2 cells attract and activate eosinophils. Classic examples of these reactions are graft-versus-host reactions, contact dermatitis from poison ivy or other chemicals, and forms of chronic asthma and atopic dermatitis.
PATHOGENESIS OF IGE-MEDIATED DISORDERS
By definition, atopic disease is caused by type I, IgE-mediated reactions, although studies indicate that the classic mast cell–bound IgE allergen reaction is not the only means by which mast cells and basophils participate in inflammation. IgE is produced primarily by plasma cells in lymphoid tissues lining the respiratory and gastrointestinal tracts, and it constitutes 0.001% of circulating immunoglobulins in normal individuals. This immunoglobulin class does not activate complement, nor does it cross the placenta. It has the unique property of binding to high-affinity receptors (FcεRI) on the surface of mast cells, basophilic granulocytes and antigen-presenting cells, and to low-affinity receptors (FcεRII) on lymphocytes, monocytes and macrophages, eosinophils, and platelets. The IgE molecule is bound by the Fc-terminal end, so the antigen-specific N-terminal end Fab is exposed and confers antigen specificity to the mast cell or basophil (sensitizes); 40,000 to 90,000 IgE molecules may bind to the cell membrane of a mast cell or basophil. On antigen-presenting cells, the IgE molecule serves as a highly efficient receptor for focusing allergen-specific T-cell responses, especially of the Th2 type.
All mammalian species make IgE antibody, and much of the information regarding its production and regulation is derived from work with rodent models. IgE-producing plasma cells are found in all lymphoid organs, but they are in the highest concentration in the lymphoid tissue of the respiratory tract (tonsils and adenoids) and gut (Peyer’s patches and lamina propria). IgE-bearing B cells are present in the human fetus by the eleventh week of gestation, but IgE production in utero is negligible.
As with other immunoglobulin classes, surface IgM-bearing, virgin B cells differentiate into surface IgE-bearing memory B cells under the influence of regulatory CD4+ helper T lymphocytes (Th). Studies have shown the presence of three types of CD4+ cells based on the profile of cytokines they generate: Th1 cells that promote cell-mediated reactions by secreting interleukin-2 (IL-2), interferon-gamma, and granulocyte-macrophage colony-stimulating factor (GM-CSF); Th2 cells that promote immunoglobulin synthesis, especially IgE synthesis, by secreting IL-4, IL-5, IL-6, IL-10, IL-13, and GM-CSF; and Th3 cells that appear to regulate the function of Th1 and Th2 cells by secreting IL-10 and transforming growth factor alpha (TGF-α). B cells differentiate and mature into IgE-secreting plasma cells in the presence of allergen, APCs, and the appropriate antigen-presenting cell- and T cell–derived cytokines. The nature of the antigen is an important component of the IgE antibody response. Certain antigens, such as penicillin, ovomucoid, ragweed antigen E, and parasitic proteins, stimulate the production of more IgE than IgG antibodies. In general, these proteins are glycoproteins in the 10,000- to 60,000-dalton molecular weight range. Immunization with low doses of these antigens favors IgE production. Antigens presented at mucosal surfaces initiate IgE production in most individuals, but the response normally is turned off rapidly by specific regulatory or suppressor T lymphocytes. Procedures that eliminate T-suppressor cells, such as irradiation or cyclophosphamide,
promote the indefinite production of high-titer IgE antibodies in animal models.
promote the indefinite production of high-titer IgE antibodies in animal models.
The modulation of IgE antibody is genetically controlled, although the development of antigen-specific IgE immune responses occur only after appropriate environmental exposure. Analogous to individual humans, certain inbred strains of mice preferentially produce IgE antibodies when immunized, whereas others produce IgG. Control appears to reside primarily with a T-helper lymphocyte population that is regulated by IgE-enhancing or IgE-inhibitory factors (cytokines) produced by APCs and other Th2 or Th3 lymphocytes, respectively. The growing body of data available concerning human IgE regulation is compatible with information derived from rodent models. Data from family and twin studies suggest that elevated serum IgE concentrations (more than 100 IU/mL) are inherited as a simple recessive trait. Complex diseases, such as asthma and allergic rhinitis, are multifactorial in that they are the result of interactions between major and minor genes and involve nongenetic interactions for their expression. Atopic diseases have a strong familial tendency. In a study of college-aged persons, the prevalence of atopic disease was 15% when no first-degree relative had one of the diseases, 33% when one first-degree relative was affected, and 68% when two or more first-degree relatives were atopic. No clear pattern of inheritance has been found, suggesting that atopy is a complex genetic trait (diabetes is another example of a complex trait). Indeed, studies have identified associated areas on several chromosomes, including 11q13 (IgE receptor) and 5q31 (IL-4, beta-adrenergic receptor). A number of alleles for the beta-adrenergic receptor have been found, including one that rapidly becomes unresponsive during continued beta-adrenergic use. Exposure to several environmental factors such as cigarette smoke, air pollutants, and allergens (mites, foods, pollens, cockroaches, and molds) have been associated with an increased risk of atopic disease.
In addition, specific IgE antibody responses more frequently are associated with specific (human leukocyte antigens) HLA specificities. For example, the development of IgE antibody to the Ra5 antigen of ragweed frequently is associated with HLA-Dw2, whereas individuals with a response to rye grass pollen have a frequency of HLA-B8 three times higher than expected.
Elevated serum IgE concentrations are not restricted to allergic disorders. Many disease states are associated with elevated levels of IgE. With atopic disorders, the IgE concentration generally is elevated in only 60% to 70% of patients and correlates roughly with disease severity. Individuals with no detectable serum or cell-bound IgE are apparently healthy, which suggests that IgE is not essential in maintaining good health. Serum containing IgE antibody can transfer sensitivity, but not particular atopic disorders, to normal individuals. The first human example was reported in 1919, when a patient with pernicious anemia was transfused with blood from a donor who was allergic to horses. The patient subsequently developed wheezing for the first time while driving home behind horses. More recently, recipients of liver transplants from food-allergic donors have experienced food allergic reactions after ingesting the food to which the donor was sensitive. Before the advent of radioimmunoassays, circulating IgE antibody was detected by its ability to sensitize normal skin (Prausnitz-Küstner reaction); the technique is still useful in animal experiments (passive cutaneous anaphylaxis). Passive sensitization occurs when IgE molecules bind with high avidity on tissue mast cells and blood basophils. In allergic individuals, a similar process occurs when mast cells and basophils are sensitized by the IgE molecules produced endogenously by plasma cells and released into the circulation.
TABLE 419.1. PHARMACOLOGICALLY ACTIVE MEDIATORS AND CYTOKINES FROM MAST CELLS AND BASOPHILS | |
---|---|
|
Sensitization confers on the mast cell or basophil the ability to respond to an allergen. Once sensitization occurs, allergen exposure causes rapid (in seconds) changes in mast cell phospholipid and calcium metabolism, which results in an energy-dependent secretion of numerous pharmacologically active mediators, listed in Table 419.1. The release of these mediators results in an immediate response (within 15 to 30 minutes), which may include vasodilation, increased vascular permeability, and smooth-muscle constriction and mucus secretion in the respiratory and gastrointestinal tracts. In addition, thromboxanes and at least two proteins with chemotactic activity (IL-5 and GM-CSF) are secreted during the immediate phase, which may contribute to the infiltration of inflammatory cells. In addition to the immediate response, mast-cell activation may result in what is termed a late-phase reaction (LPR). As shown in Figure 419.1, the injection of an allergen causes an immediate wheal-and-flare reaction within 10 to 20 minutes. During the next 2 to 4 hours, the site may remain somewhat erythematous and edematous, but generally is not symptomatic. After 6 to 8 hours, the test site becomes pruritic (sometimes tender), warm, and more edematous and erythematous. This LPR may last 12 to 48 hours, although discoloration caused by extravasation of erythrocytes in severe reactions may persist for days. Histologically, lesions of LPRs show edema and perivascular infiltration of eosinophils and neutrophils. After 48 hours, mononuclear cells predominate in the cellular infiltrate, and the histology appears similar to the classic type IV, cell-mediated response. Studies have demonstrated that infiltrating lymphocytes are allergen-specific CD4+ Th2 cells (as opposed to CD4+ Th1 cells in type IV responses), which would promote further IgE synthesis and upregulation of IgE receptors on many cell types and would attract inflammatory cells. In addition to cytokines secreted by lymphocytes, it has been shown that tissue macrophages, mast cells, and eosinophils secrete a variety of
cytokines (interleukins) that promote the IgE allergen-driven inflammatory response. In the nose, the LPR causes persistent nasal obstruction and hypersecretion. In the lung, LPR is associated with a persistent airflow obstruction that responds only partially to bronchodilator therapy. Airway bronchial hyperresponsiveness (propensity of the airways to obstruct secondary to nonimmunologic stimuli) may be measured by the concentration of histamine or methacholine required to cause significant airflow obstruction. This hyperresponsiveness may remain increased for weeks after initiation of the LPR (Fig. 419.2). Histologic examination of the lung in animal models and bronchial biopsies in man demonstrate an infiltrate similar to that seen in the LPR in human skin.
cytokines (interleukins) that promote the IgE allergen-driven inflammatory response. In the nose, the LPR causes persistent nasal obstruction and hypersecretion. In the lung, LPR is associated with a persistent airflow obstruction that responds only partially to bronchodilator therapy. Airway bronchial hyperresponsiveness (propensity of the airways to obstruct secondary to nonimmunologic stimuli) may be measured by the concentration of histamine or methacholine required to cause significant airflow obstruction. This hyperresponsiveness may remain increased for weeks after initiation of the LPR (Fig. 419.2). Histologic examination of the lung in animal models and bronchial biopsies in man demonstrate an infiltrate similar to that seen in the LPR in human skin.
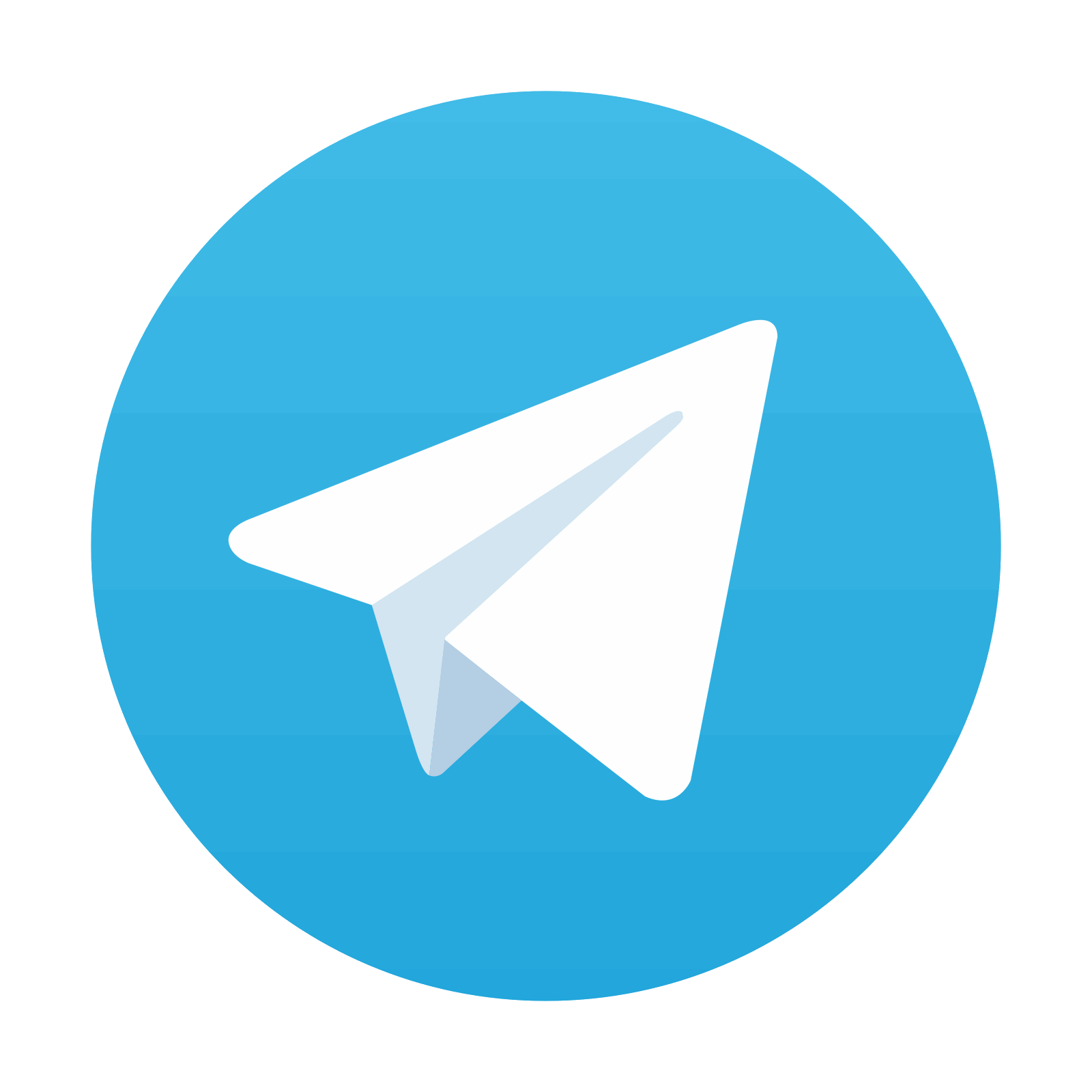
Stay updated, free articles. Join our Telegram channel

Full access? Get Clinical Tree
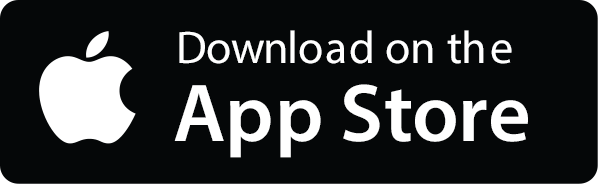
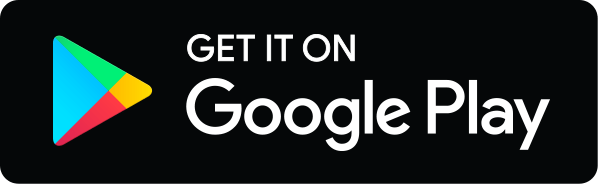