Ultrasound has progressed rapidly in recent years in terms of the technology itself and how it has been applied to both clinical practice and research. Musculoskeletal ultrasound is now considered a major subspecialty of radiology, and manufacturers have been responsive in making the technology more versatile and user-friendly. It is considered a first-line investigative tool for many musculoskeletal diseases although the politics and structure of local health systems often dictate which modality should be applied and when. For example, in the United States, magnetic resonance imaging (MRI) and computed tomography (CT) have traditionally been preferred over ultrasound although ultrasound is now beginning to be accepted by insurance companies particularly with the realization that it may be more cost-effective.
Advances in Technology
Transducers
Modern transducer technology has contributed greatly to the use of ultrasound in rheumatology. Improved transducer design has enabled the use of higher frequencies for detailed superficial work ( Figs. 26-1 and 26-2 ). Other improvements include the use of broadband, compound, and harmonic imaging in addition to more sensitive Doppler.


Matrix array transducers, used for both two-dimensional (2D) and three-dimensional (3D) scanning, are likely to play an increasing role in ultrasound. They are composed of crystals that allow focusing in the near, middle, and far fields. This leads to versatile near-field and penetrating imaging with superior gray-scale tissue differentiation ( Fig. 26-3 ). They also enable, higher Doppler frequencies to be achieved with subsequent lower flow sensitivity. Currently, these transducers utilize relatively low frequencies, hence their predominant use in echocardiography rather than rheumatology, but this is likely to change with time.

Three-Dimensional Ultrasound
Three-dimensional ultrasound offers an interesting prospect for the volumetric assessment of tissues using gray-scale or Doppler imaging. In a clinical setting, this technology has been used most commonly for fetal assessment and cardiology, but its applications are steadily growing. In rheumatology, it serves mainly as a research tool and is not routinely available in most centers.
Instead of using a conventional image-freeze-print process as in conventional 2D ultrasound, 3D transducers acquire a block of tissue consisting of up to 25 specific slices. The advantages of this approach include increased speed of image acquisition and the potential for improved reliability and image quantification. Transducers are large and heavy ( Fig. 26-4 ) and somewhat cumbersome to use. They are held firmly in position while an internal mechanized drive scans a sector of tissue. The saved images can be viewed in coronal, transverse, sagittal, and axial planes.

One of the best features of 3D ultrasound is its ability to quantify regions of interest. However, currently there is no commercially available software to quantify a block of tissue for gray-scale or Doppler ultrasound. When the software becomes available, the use of 3D ultrasound is likely to grow in rheumatology. Until then, the additional cost of the technology and the reduction in image quality prohibits its widespread use.
Fusion Imaging
The concept of fusion imaging describes the simultaneous mapping of one type of image modality onto another, preacquired image modality. In this way, a live ultrasound image can be directly compared and mapped onto a preacquired 3D multiplanar reslice (MPR) CT or MRI volume dataset.
The technique can be used only on high-end machines, but several companies provide the technology. The hardware consists of an external box ( Fig. 26-5 ) that is suspended from a trolley device. This acts as the transmitter of the electromagnetic field. An adjustable arm allows positioning of the box as close to the region of interest as possible. The distance between the transmitter and the scanning region of interest should remain constant throughout the examination. The receivers or sensors are attached to the transducer by means of a clip-on attachment in the example shown ( Fig. 26-6 ).


There are theoretical advantages of fusion technology, but whether it provides added value in a clinical setting is uncertain. The technology was first developed for interventions such as guiding biopsy needles, but it can also be used for injections such as in the sacroiliac joints. From a research perspective, it is useful for the validation of bone or soft tissue lesions seen on ultrasound or with other techniques ( Fig. 26-7 ).

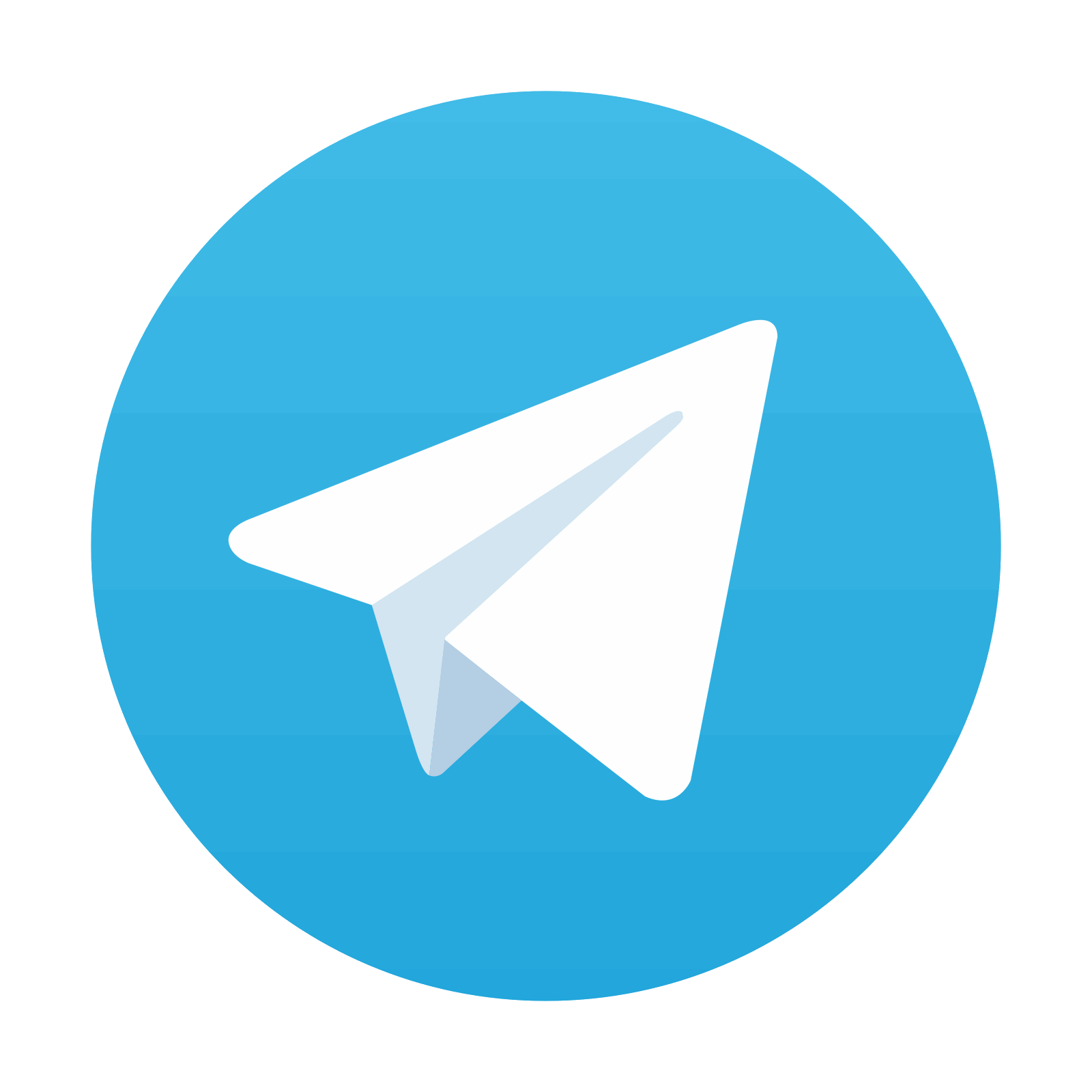
Stay updated, free articles. Join our Telegram channel

Full access? Get Clinical Tree
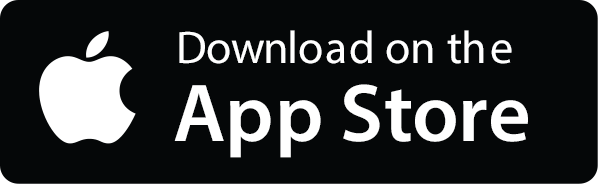
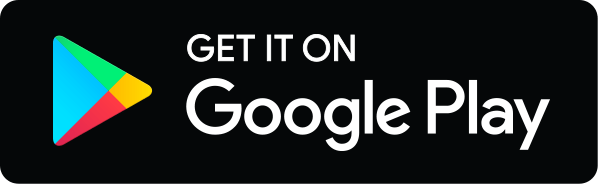
