CHAPTER 2 Functions of the autonomic nervous system
current concepts
Introduction
The maintenance of physiological parameters such as concentrations of ions, blood glucose, arterial blood gases, body core temperature, etc. in a narrow range is called homeostasis (Cannon 1929, 1939). This process of maintaining the stability of the internal milieu during changes in the body and in the environment requires systems that have a large range of activity, such as the cardiovascular system, the thermoregulatory system, the metabolic system (involving the gastrointestinal tract and endocrine systems releasing insulin, glucagon, leptin and thyroxin) or the immune system. The autonomic and endocrine homeostatic regulations are represented in the brain (i.e., in the hypothalamus, brain stem, and spinal cord) and under the control of the telencephalon. They are integrated at all central neural levels with the somatomotor and sensory representations of the body, leading to tight coordination of autonomic and somatomotor regulation. Thus the brain contains autonomic ‘sensorimotor programs’ for the regulation of the internal environment of the body and sends efferent commands to the peripheral target tissues through the autonomic and endocrine routes.
The model in Fig. 2.1 outlines the role of the autonomic nervous system in the generation of behavior. Behavior is defined as purposeful motor action of the body in the environment. It is generated by coordinated activation of the three divisions of the motor system: the somatic, the autonomic, and the neuroendocrine motor systems (Swanson 2000, 2008). The somatomotor system moves the body in the environment. The autonomic and neuroendocrine motor systems prepare and adjust the internal milieu and body, enabling the body to move. Both are also important to protect the body against real and potential short- and long-term injuries occurring continuously from outside as well as from within the body.
Based on this concept I will discuss (1) the principles of the organization and functioning of the autonomic nervous system in the periphery and in the CNS (Jänig 2006, Jänig & McLachlan 2002) and (2) the role of the sympathetic nervous system in body protection (including pain, hyperalgesia, and infla-mmation)(Jänig & Levine 2006, Jänig 2009a).
Organization of peripheral autonomic pathways
Definition of the autonomic nervous system and visceral afferent neurons
Langley (1921) originally proposed the generic term ‘autonomic nervous system’ to describe the innervation of virtually all tissues and organs except striated muscle fibers and the brain. Langley’s division of the autonomic nervous system into the sympathetic, parasympathetic, and enteric nervous systems is now universally applied. The definition of the sympathetic and parasympathetic nervous systems is primarily anatomical (the thoracolumbar system or sympathetic system; the craniosacral or parasympathetic system). The enteric nervous system is intrinsic to the wall of the gastrointestinal tract and consists of interconnecting plexuses along its length (Furness 2006, Jänig 2006).
The peripheral sympathetic and parasympathetic pathways consist of two populations of neurons. The cell bodies of the postganglionic neurons are grouped in autonomic ganglia. Their axons are unmyelinated and project from these ganglia to the target organs. The cell bodies of the preganglionic neurons lie in the spinal cord and brain stem. They send axons from the CNS into the ganglia and form synapses on the dendrites and cell bodies of the postganglionic neurons. Their axons are myelinated as well as unmyelinated. The peripheral sympathetic and parasympathetic pathways are synaptically linked to distinct central circuits in the spinal cord, brain stem, and hypothalamus; the principle of organization of these circuits will be discussed below (Fig. 2.2).
In the definition of the terms sympathetic and parasympathetic, afferent neurons are not included. About 85% of the axons in the vagus nerves and up to 50% of those in the (spinal) splanchnic nerves are afferent and are called vagal or spinal visceral afferents, respectively. They come from sensory receptors in the internal organs and have their cell bodies in the ganglia of the ninth and tenth nerves, and in the dorsal root ganglia of the spinal segments corresponding to the autonomic outflow. To label thoracolumbar and sacral afferents ‘sympathetic’ or ‘parasympathetic,’ respectively, is misleading. This does not conflict with the idea that visceral afferents are integral components of most autonomic reflexes and regulations (Jänig & Koltzenburg 1993, Cervero 1994, Undem & Weinreich 2005, Jänig 2005, 2006). In fact, visceral afferent neurons, together with small-diameter (Aδ, C) afferent neurons innervating almost all somatic tissues, monitor the mechanical, thermal, chemical, and metabolic states of the body tissues. These afferent neurons are also involved in homeostatic regulations and regulation of body protection (see pp. 39–43 [Sympathetic nervous system and body protection]). Their activation leads to interoceptive body sensations that include pain, heat, sensual touch, itch, various visceral sensations, respiratory sensations, and sensations of deep somatic tissues (e.g., during exercise). Thus, these body sensations belong to interoception ‘as the sense of the physiological conditions of the entire body’ and have a distinct primary cortical representation in the insular cortex (Craig 2002, 2003a, b,c). This concept of interoception extends the concept of Sherrington (1900), who limited it to the viscera (Jänig 2009a).
Functional characteristics and anatomy of peripheral autonomic pathways
Many peripheral autonomic neurons exhibit spontaneous activity and/or can be activated or inhibited reflexly by appropriate physiological stimuli. This has been shown (1) in anesthetized animals (mainly cats and rats) for neurons of the lumbar sympathetic outflow to skeletal muscle, skin, and pelvic viscera (Jänig 1996, Jänig & McLachlan 1987), and for neurons of the thoracic sympathetic outflow to the head and neck; (2) in awake humans for the sympathetic outflow to skeletal muscles and skin (Jänig 2006, Jänig & Häbler 2003, Wallin 2002); and (3) in animals for some parasympathetic systems innervating inner eye muscles, heart, trachea and bronchi, upper gastrointestinal tract, urinary bladder, and colon. The reflexes observed correspond to the effector responses which are induced by changes in activity of these autonomic neurons. The reflex patterns elicited by physiological stimulation of various afferent input systems represent physiological ‘fingerprints’ (markers) for each autonomic pathway.
Reflex Patterns in Sympathetic Neurons: Animal Experiments
Figures 2.3 and 2.4 illustrate examples of reflex responses in sympathetic neurons regulating blood vessels in skeletal muscle (muscle vasoconstrictor neurons, MVC), skin (cutaneous vasoconstrictor neurons, CVC), possibly the nasopharyngeal mucosa (‘inspiratory’ neurons, INS) and sweat glands (sudomotor neurons, SM) in the anesthetized cat. The overall results obtained on sympathetic neurons are:
More than 10 different functional groups of post- and preganglionic sympathetic neurons have been identified in the lumbar sympathetic outflow to skin, skeletal muscle, and viscera, and in the thoracic sympathetic outflow to the head or neck of the cat (see Fig. 2.5 for the lumbar sympathetic outflow to skin, skeletal muscle, and pelvic organs). The same types of reflex patterns have been observed in both pre- and postganglionic neurons. Most neurons in several of these pathways (e.g., the vasoconstrictor, sudomotor, and motility-regulating pathways) have ongoing activity, whereas in four investigated pathways (e.g., the pilomotor and vasodilator pathways) the neurons are normally silent. Other functionally distinct groups of sympathetic neurons which have not been studied so far are likely to innervate other autonomic target cells (e.g., the kidney [blood vessels, juxtaglomerular cells], the urogenital tract [urinary bladder, vas deferens, erectile tissue, glandular tissue], the hindgut [non-vascular smooth musculature or glandular tissue via the enteric nervous system], the spleen [immune tissue], the heart, the brown adipose tissue [Morrison 1999, 2001], etc.).
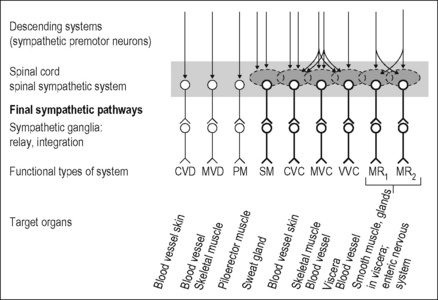
Figure 2.5 Lumbar sympathetic systems supplying skeletal muscle and skin of the hindlimb and pelvic organs (including distal colon) in the cat. The preganglionic neurons of these systems are largely located in the lumbar segments L1 to rostral half L5 (the cat has seven lumbar segments) and project in the lumbar sympathetic trunk distal to paravertebral ganglion L5 or in the lumbar splanchnic nerves and the hypogastric nerves. The first group projects (with a few exceptions related to pelvic organs) to postganglionic neurons innervating somatic tissues, and the second group to postganglionic neurons innervating pelvic organs or colon. The second group includes other sympathetic pathways associated with the internal sexual organs, and probably other target cells in the pelvic organs which are not shown in the figure. Preganglionic neurons are associated with spinal circuits (shaded areas) and are under multiple supraspinal control via sympathetic premotor neurons (see Fig. 2.12). Sympathetic neurons with spontaneous activity in bold. CVC, cutaneous vasoconstrictor; CVD, cutaneous vasodilator; MR, motility-regulating; MVC, muscle vasoconstrictor; MVD, muscle vasodilator; PM, pilomotor; SM, sudomotor; VVC, visceral vasoconstrictor.
Modified from Jänig (2006).
Reflex patterns in sympathetic neurons: studies in humans
Activity in bundles with few or single discriminated sympathetic postganglionic axons in human skin or muscle nerves can be recorded in the awake subject with tungsten electrodes inserted manually into the nerve. Using this microneurographic recording technique, it has clearly been shown that muscle vasoconstrictor, cutaneous vasoconstrictor, and sudomotor neurons have the same distinct activity patterns as in the cat or rat, and that there is also evidence for the existence of sympathetic vasodilator neurons supplying skin or skeletal muscle in humans (Jänig & Häbler 2003, Wallin 2002). However, in humans we have no recordings from sympathetic neurons innervating viscera or head, and no recordings from preganglionic neurons (Jänig 2006).
Reflex patterns in parasympathetic neurons
Several systematic studies have been carried out on the functional properties of the parasympathetic pre- and postganglionic neurons involved in the regulation of pelvic organs, gastrointestinal tract, heart, airways, salivary glands, and inner eye muscles. There are good reasons to assume that the principle of organization into functionally discrete pathways is the same as in the sympathetic nervous system, the main difference being that some targets of the sympathetic system are widely distributed throughout the body (e.g., blood vessels, sweat glands, erector pili muscles, fat tissue), whereas the targets of parasympathetic pathways are more restricted (for details and references see Jänig 2006).
Functional implications of the neurophysiological studies of autonomic neurons in vivo
These neurophysiologic studies in animals and humans argue that the peripheral autonomic nervous system consists of many separate pathways. This separation is supported by a substantial amount of histochemical evidence demonstrating that, depending on their function, peripheral autonomic neurons may contain neuropeptides or combinations of neuropeptides in addition to the classic transmitters noradrenaline and acetylcholine. The co-localized peptides in autonomic neurons are to a certain degree correlated with the function of the autonomic neurons, although this correlation is not straightforward and absolute; although there are species differences; and although the function of almost all peptides in these neurons is unknown (Gibbins 1995, 2004).
Signal transmission in peripheral autonomic pathways
Autonomic ganglia
In peripheral autonomic ganglia preganglionic axons diverge to and converge on postganglionic neurons. Both divergence and convergence occur within, but not between, functionally distinct autonomic pathways. A major function of peripheral autonomic ganglia is to distribute the centrally generated signals by connecting each preganglionic neuron with several postganglionic neurons. In this way a small number of preganglionic neurons connect with a large number of postganglionic neurons. The extent of divergence varies significantly, the ratio of pre- to postganglionic axons being, in pathways such as in the ciliary ganglion to the iris and ciliary body, as low as 1:4, and in others, such as in the superior cervical ganglion with many vasoconstrictor neurons, as high as 1:150. A limited divergence and much divergence are not characteristics of the parasympathetic or sympathetic systems, respectively. Probably, by analogy with somatic motor units, limited divergence is common in pathways to small targets with discrete functions (e.g., in autonomic pathways to the inner muscles of the eye, or the parasympathetic cardiomotor pathway to cardiac pacemaker cells), whereas widespread divergence is a feature of pathways to anatomically extensive effectors that act more or less simultaneously (e.g., of cutaneous, muscle, or visceral vasoconstrictor pathways) (McLachlan 1995, Jänig 2006).
Sympathetic paravertebral ganglia
Most postganglionic neurons in sympathetic paravertebral ganglia (in the sympathetic chains) innervate autonomic targets in somatic tissues; some innervate viscera (e.g., the heart) or targets of the head (e.g., the intracranial blood vessels, salivary and nasopharyngeal glands, intraocular smooth muscles). Within these ganglia, ganglionic neurons have uniform properties. Each convergent cholinergic preganglionic axon produces excitatory postsynaptic potentials by activating cholinergic nicotinic receptor channels. The amplitude of the potentials ranges from a few millivolts (subthreshold weak synaptic inputs) to 10–40 mV (suprathreshold strong synaptic inputs). In most cases, one or a few strong preganglionic synaptic inputs are always suprathreshold, such as the endplate potential at the skeletal neuromuscular junction. They have a high safety factor and always initiate an action potential. Thus, these ganglion cells relay the incoming CNS-derived signals in only a few of their converging preganglionic inputs (McLachlan et al. 1997, 1998). The function of weak preganglionic synaptic inputs generating small subthreshold potentials in paravertebral ganglia is not clear (Fig. 2.6A).
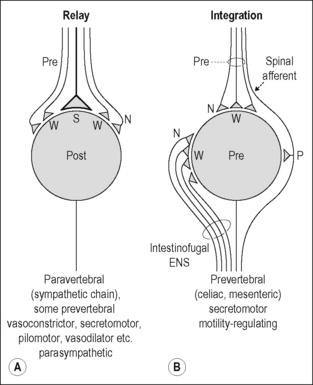
Figure 2.6 Signal transmission in peripheral autonomic pathways: Transmission through autonomic ganglia. A. Relay functions of autonomic ganglion cells. Postganglionic neuron with one or a few strong (S; suprathreshold) preganglionic synaptic inputs (generating postsynaptic potentials of 10–30 mV) and several weak (W; subthreshold) synaptic inputs (generating synaptic potentials of a few mV). This connectivity occurs in almost all neurons in paravertebral sympathetic ganglia, in some neurons of prevertebral ganglia, and in parasympathetic ganglia. These connections function mainly to transmit the activity from pre- to postganglionic neurons (McLachlan et al. 1997, 1998). B. Relay and integrative functions of autonomic ganglion cells in prevertebral ganglia. Postganglionic neuron with weak synaptic inputs from both preganglionic neurons and interneurons of the enteric nervous system (ENS; intestinofugal neurons), and also from collaterals of spinal visceral afferents. The first two are nicotinic cholinergic (N). The afferent collaterals are peptidergic and use substance P (SP) as transmitter. These postganglionic neurons innervate neurons of the enteric nervous system and possibly other target organs in the viscera (but not blood vessels). They fire only after integration of several subthreshold cholinergic synaptic inputs and/or a slow afferent-induced depolarization.
Modified from Jänig & McLachlan (2002).
Sympathetic prevertebral ganglia
Neurons in prevertebral (sympathetic) ganglia innervate viscera (gastrointestinal tract, pelvic organs, kidney, and spleen). These postganglionic neurons do not have uniform properties. Three broad groups differ electrophysiologically (by the K+ channels that control excitability), morphologically (by their size and dendritic branching), and neurochemically (by their neuropeptide content). Two groups, e.g., paravertebral neurons, have suprathreshold synaptic connections with one or a few preganglionic axons which determine the firing pattern of these neurons, one of them having vasoconstrictor function and the other being involved in regulation of motility or secretion. The mode of synaptic transmission in the third group is different. These neurons receive weak preganglionic synaptic inputs and many weak cholinergic nicotinic inputs from intestinofugal neurons of the enteric nervous system, which can be activated by mechanical stimulation of the gastrointestinal tract. Summation of synaptic potentials from peripheral and preganglionic inputs is necessary to initiate their discharge. These postganglionic neurons do not have vasoconstrictor function, but are involved in regulation of motility or secretion. Some of them, possibly the secretomotor neurons, also receive synaptic input from collaterals of visceral primary afferent neurons (which have their cell bodies in the dorsal root ganglia) and depolarize slowly when these inputs are activated at relatively high frequency. The slow responses are generated by the neuropeptide substance P released from the afferent collaterals. This third group of prevertebral neurons therefore depends on temporal and spatial integration of incoming synaptic signals and may establish peripheral (extraspinal) reflexes (Jänig & McLachlan 1992, 2002, Jänig 2006) (Fig. 2.6B).
Parasympathetic ganglia
The structure of many parasympathetic ganglion cells, with few dendrites, is simpler than that of sympathetic neurons. The preganglionic input is correspondingly simple, often consisting of a single suprathreshold input. However, in addition to postganglionic neurons, some parasympathetic ganglia in the body trunk (e.g., cardiac ganglia) contain neurons that behave as primary afferent neurons or interneurons. These ganglia may have the potential for reflex activity independent of the CNS, like the enteric nervous system (McLachlan 1995, Jänig 2006).
The pelvic or hypogastric plexuses contain the neurons that innervate the pelvic organs. Some of these ganglion cells are noradrenergic and are innervated by lumbar sympathetic preganglionic axons; others are cholinergic and receive sacral parasympathetic inputs. A small proportion of pelvic neurons receive synaptic connections from both sympathetic preganglionic neurons projecting through the hypogastric nerves and parasympathetic preganglionic neurons projecting through the pelvic nerves (Keast 1995).
Transmission of signals at the autonomic neuroeffector junctions
In peripheral tissues the transmission of activity in autonomic nerve terminals onto autonomic effector cells is complex and depends on the release of several different compounds and on the presence and distribution of the receptors in the effector membranes for these compounds. Anatomical investigations of neuroeffector junctions at arterioles, veins, pacemaker cells of the heart, cells of the vas deferens, the iris dilator myoepithelium, and the longitudinal muscle of the gastrointestinal tract have demonstrated that varicosities of autonomic nerve fibers which are not surrounded by Schwann cells form close synaptic contacts with the effector cells (Hirst et al. 1992, 1996). These structures are the morphological substrate for the transmission of the centrally generated signals by the postganglionic neurons to the effector cells (Fig. 2.7). The close synaptic contacts occur on <1% of the surface of effector cells. Neuroeffector transmission on arterioles, small arteries, the heart, the vas deferens, the iris dilator myoepithelium, and the longitudinal musculature of the gastrointestinal tract has been studied in in-vitro preparations using neurophysiological and other methods. These investigations show that signal transmission from postganglionic axons to autonomic effector cells occurs via close neuroeffector junctions and postjunctional receptors, but not – or only to a limited degree (as far as vascular target cells are concerned) – via extrajunctional receptors. This specific method of neuroeffector transmission is the basis upon which signals generated in specific central circuits (see below) are channeled to the appropriate autonomic target cells.
Chemical transmission at these neuroeffector junctions is based on the release of one of the ‘conventional’ transmitters, acetylcholine or noradrenaline. Most sympathetic postganglionic axons release noradre-naline, but some release acetylcholine (e.g., sudomotor and muscle vasodilator axons in some species). Most (but not all) nerve-mediated effects can be antagonized by blockade of adrenoceptors or muscarinic acetylcholine receptors. All parasympathetic neurons are cholinergic, i.e., they release acetylcholine on stimulation. However, not all effects of stimulating parasympathetic nerves are blocked by muscarinic antagonists. This clearly implies that other transmitters and/or other receptors may be involved. It is now clear that several chemical substances, often contained within individual autonomic neurons, can be released during excitation and can have multiple actions on effector tissues (Furness et al. 1989, Morris & Gibbins 1992). The compounds which may be involved are nitric oxide (NO), adenosine triphosphate (ATP), or a neuropeptide (e.g. vasoactive intestinal peptide [VIP], neuropeptide Y [NPY], galanin [GAL] and other peptides). Immunohistochemistry has revealed the presence of many peptides, although only a few of these have been demonstrated to modify the function after release from nerve terminals in vivo (e.g., NPY or VIP) (Gibbins 1995, 2004, Gibbins et al. 2003).
The response of autonomic target tissues to nerve-released noradrenaline or acetylcholine or other compounds usually only follows repetitive activation of many axons. High-frequency stimuli, particularly in bursts, may produce effector responses owing to the concomitant release of a neuropeptide. Alternatively, when the effects of nerve activity are not completely blocked by an adrenoceptor or muscarinic antagonist at a concentration that entirely abolishes the response to exogenous transmitter, it may not necessarily be the case that a transmitter other than acetylcholine or noradrenaline is involved (as the subjunctional receptors may be different from the extrajunctional ones; and as the concentration of transmitter released into the junctional cleft may reach millimolar concentrations). Although the effects of exogenously applied substances, which have putative transmitter function, on cellular functions are known for many tissues, the consequences of activation of postjunctional receptors by neurally released transmitters have rarely been investigated. When they have, the mechanisms of neuroeffector transmission have been found to be diverse, involving a range of cellular events (Jänig & McLachlan 2002). One important concept that has emerged is that the cellular mechanisms utilized by an endogenously released transmitter are often not the same as when this transmitter substance or its analogue is applied exogenously. The endogenously released transmitter acts primarily or exclusively on the subjunctional receptors, whereas the exogenously applied transmitter acts on the extrasynaptically located receptors (Fig. 2.7, Hirst et al. 1996).
Conclusions
The experimental studies of the peripheral autonomic systems show that (Fig. 2.8):
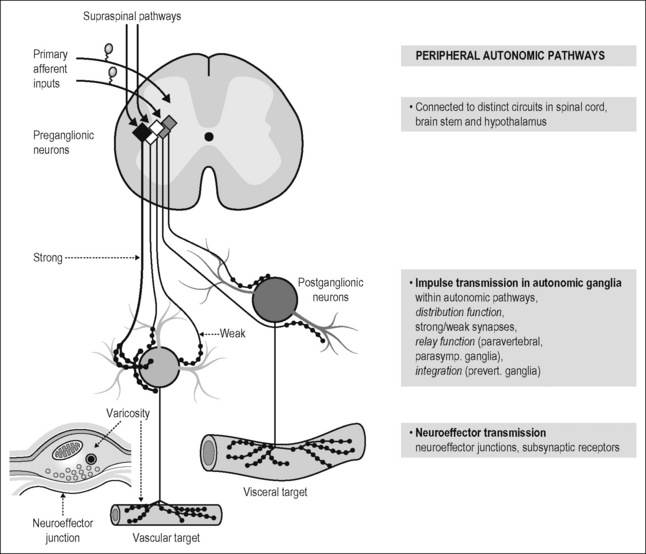
Figure 2.8 Organization of spinal autonomic systems into functional pathways. Separate functional pathways extend from the spinal cord to the effector organs. Preganglionic neurons located in the intermediate zone of the thoracolumbar spinal and sacral cord integrate signals descending from the brain stem and hypothalamus and segmentally from primary afferent fibers. This integration involves several classes of autonomic interneurons in the spinal cord. A similar organization exists for parasympathetic pathways originating from the brain stem. The preganglionic neurons project to peripheral ganglia and converge onto postganglionic neurons. Some preganglionic inputs to postganglionic neurons are always suprathreshold (or strong; see Fig. 2.6A). Others are subthreshold (weak). Subthreshold (weak) postganglionic potentials must either summate to generate an action potential (in some postganglionic neurons of prevertebral ganglia) or their functions are unknown (see Figs 2.6A, B). The postganglionic axons form multiple neuroeffector junctions with their target cells. Many varicosities of the terminal axons which contain the synaptic vesicles with the transmitter(s) form close contacts with the target cells (neuroeffector junctions; see Fig. 2.7). For details of this concept see Jänig (2006) and Jänig & McLachlan (2002).
Modified from Jänig & McLachlan (1992).
Central organization of autonomic systems
The central representation of the autonomic functions is organized in the spinal cord, brain stem, hypothalamus, and telencephalon. The reflex discharge patterns by which peripheral autonomic neurons are functionally characterized (see Figs 2.3 and 2.4) reflect this central organization. The concept of hierarchical organization of autonomic functions in the CNS is not absolute and must not be taken too literally. However, it helps to understand the structural and physiological basis of the regulation of peripheral autonomic pathways by the brain.
Spinal cord
Segmental organization of the spinal afferent and sympathetic innervation
The basis of the spatial organization of the viscerosomatic and somatovisceral reflexes, including referred pain in skin and deep somatic tissues, is the segmental (spinal) organization of the afferent and sympathetic innervation of the three body domains. This is particularly visible at the trunk of the body. Functional morphological studies on rats using tracers such as horseradish peroxidase applied either to a dorsal cutaneous nerve or an internal intercostal nerve have shown that about 90% of all sympathetic postganglionic neurons that project to the skin of the trunk through the dorsal or ventral ramus of a spinal nerve have their cell bodies in the paravertebral ganglia of the corresponding segment and of the adjacent caudal segment, the remaining postganglionic cell bodies being located in the two rostral paravertebral ganglia. For example, the cell bodies of the postganglionic neurons innervating dermatome C and projecting through segmental nerve C in Figure 2.9 are located in paravertebral ganglia 3 and 4 (90%) and 1 and 2 (10%). The cell bodies of the primary afferent neurons that innervate dermatome C and project through the segmental spinal nerve C are located in spinal ganglion 3 (Baron et al. 1995). All preganglionic neurons of a spinal segment project through the ventral root and the corresponding white ramus of this segment. In the spinal cord the preganglionic neurons are arranged in rostrocaudal columns and in clusters in the intermediate zone. There is some topical organization with respect to the type of target (Baron et al. 1985, Jänig 2006). Preganglionic axons preferentially form synapses with postganglionic neurons that innervate effector cells in the corresponding dermatome, myotomes, or sclerotome. The degree of branching of individual preganglionic axons is unknown. However, individual preganglionic neurons do not seem to project up and down into the sympathetic chain, and do not project into functionally different nerves (e.g., both into a splanchnic nerve innervating viscera and into the sympathetic chain to ganglia projecting to somatic tissues).
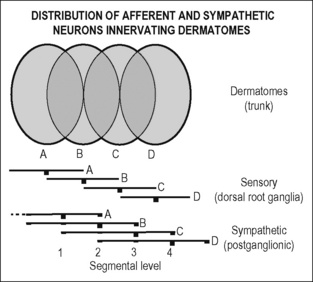
Figure 2.9 Segmental organization of postganglionic sympathetic neurons and primary afferent neurons to the skin of the trunk. A. Dorsal root ganglia (sensory) and paravertebral ganglia (sympathetic) are indicated by vertebral bars. The height of the bars indicates the relative numbers of neurons that project through the corresponding segmental nerve to the dermatome. Neighboring dermatomes overlap. Cell bodies of all afferent neurons are located in one dorsal root ganglion (e.g., for dermatome C in dorsal root ganglion 3). The cell bodies of the postganglionic neurons are located to 90% in the corresponding or the next caudal paravertebral (sympathetic) ganglion (e.g., for dermatome C in ganglions 3 and 4), and the remaining postganglionic neurons are located in the next two rostral paravertebral ganglia (for dermatome C in 1 and 2). This means that most postganglionic neurons in one paravertebral ganglion project in two overlapping dermatomes (e.g. 2 in B and A). According to data using the tracer horseradish peroxidase to locate cell bodies obtained in the rat from Baron et al. (1995).
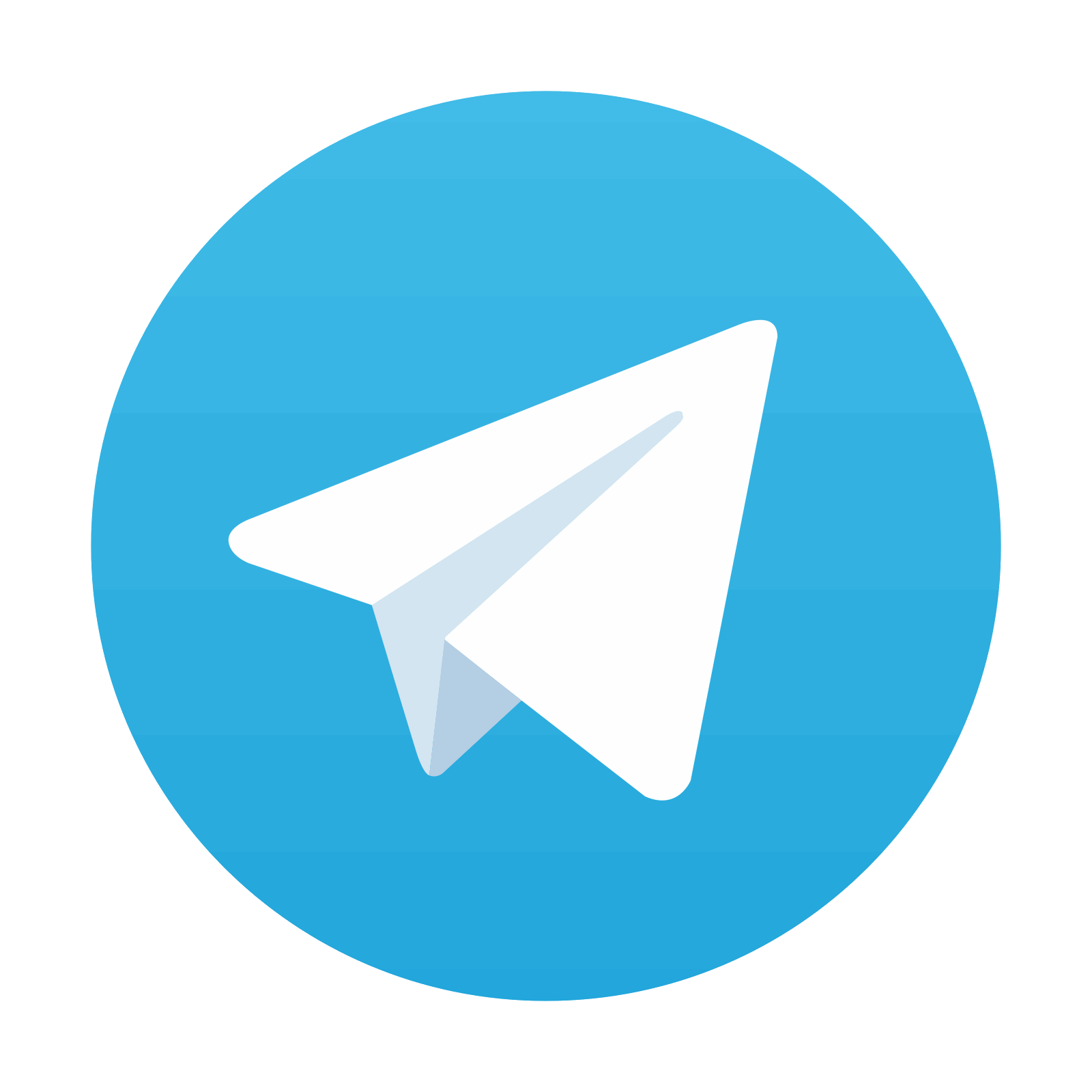
Stay updated, free articles. Join our Telegram channel

Full access? Get Clinical Tree
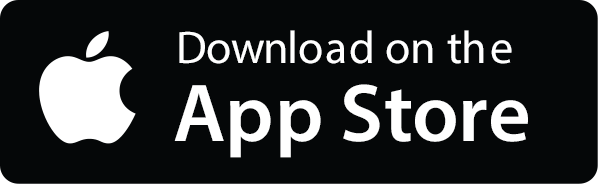
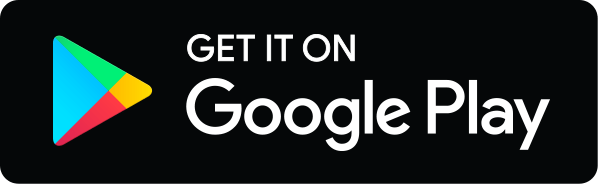