Fig. 10.1
The core is a three-dimensional structure resembling a cube or sphere. Viewing it as a cube, with each side contributing a function, while overlapping with one another (like in a sphere), helps in understanding its function and purpose
The muscles and tissues of the core are arranged in layers, each with a different function. These are the inner, middle, and outer layers of the core. The outer layer is made up of the power muscles, such as the rectus abdominis. The middle layer is made up of the abdominal and back-stabilizing muscles. And finally, the inner most layer is comprised of the intersegmental muscles and proprioceptive structures, such as the multifidi and nerves [4] (Fig. 10.2).


Fig. 10.2
The core is arranged spherically with a complex arrangement of tissues . The three layers that make it up are the outer, middle, and inner. Each one contributes a specific function to the core’s action. The outer layer functions by producing muscular power. The middle layer uses its muscles and tissues to stabilize the core. The inner layer functions in providing neural feedback to the body
Looking at the core as a cube simplifies its complexity. All the described borders are connected to one another and overlap. They all function together as a unit in body mechanics to allow transition of forces and stability (Fig. 10.2).
The anterior core consists of the rectus abdominis, the external and internal obliques, the transversus abdominis and their aponeurosis (Table 10.1 and Fig. 10.3a). These muscles connect the ribcage and the pelvis. The rectus abdominis is the most superficial muscle and originates on the pubis of the pelvis, inserting onto the cartilage of the xiphoid, fifth, sixth, and seventh ribs. It flexes the lumbar spine, straightens the pelvis, maintains intra-abdominal pressure, and assists in expiration. The external oblique originates on the surfaces of ribs 5 to 12 and inserts on the iliac crest and anterior rectus sheath. When it contracts unilaterally, it causes bending of the trunk to the ipsilateral side of contraction and rotation to contralateral side. Bilateral activation of the external obliques leads to flexion of the trunk, straightening of the pelvis and increases intra-abdominal pressure. The internal oblique lies between the external oblique and the transversus abdominis. It increases intra-abdominal pressure similar to the external oblique. It bends and rotates the trunk to the ipsilateral side. It originates from the thoracolumbar fascia, iliac crest, and anterior superior iliac spine and inserts on the lower borders of ribs 10 to 12, the linea alba, and rectus sheath. The deepest muscle is the transversus abdominis. It originates on the posterior–lateral portions of the deep thoracolumbar fascia, anterior iliac spine, and iliac crest. It inserts on the inner surface of the 7th to 12th ribs, the rectus sheath, and the linea alba. It bends the trunk unilaterally to the same side of contraction, and rotates to the opposite side. With bilateral contraction, it assists in flexion of the trunk and lumbar spine, increases intra-abdominal pressure, and assists in expiration. It is noteworthy that all the origins and insertions have connections to the hip, pelvis, and lumbar spine [2, 3].

Table 10.1
The different muscle layers for the anterior, posterior core, lateral borders, floor, and ceiling
Anterior core muscles | Lateral core muscles |
Rectus abdominis | Gluteus maximus |
External oblique | Gluteus minimus |
Internal oblique | Gluteus medius |
Transversus abdominis | External oblique |
Posterior core muscles | Internal oblique |
Erector spinae group | Transversus abdominis |
Iliopsoas | Ceiling core muscle |
Quadratus lumborum | Abdominal diaphragm |
Multifidi | Floor core muscle |
Thoracolumbar fascia | Pelvic diaphragm/ pelvic floor muscles |

Fig. 10.3
(a) The anterior abdomen and core muscles. (b) The posterior aspect of the torso and muscles
The posterior portion of the core consists of the erector spinae, iliopsoas, quadratus lumborum, multifidi muscles, and the thoracolumbar fascia. The erector spinae originates at the sacrum, iliac crest, lumbar spinous processes, and lumbar transverse processes (Table 10.1 and Fig. 10.3b) and inserts onto the 2nd to 12th ribs and thoracic vertebral transverse processes. During bilateral contraction, the erector spinae causes the lumbar spine to extend; unilateral contraction leads to lateral bending to the ipsilateral side. The iliopsoas originates on the T12-L5 vertebral bodies, L1-L5 transverse processes and intervertebral disks, as well as the iliac fossa. It inserts on the lesser trochanter of the femur. The iliopsoas causes hip flexion, internal rotation at the hip and tilts the pelvis anteriorly, exaggerating the lumbar lordosis. Quadratus lumborum originates from the iliac crest and inserts on the 12th rib and L1-L4 vertebrae. It has three poles: superior, longitudinal, and inferior. The inferior pole is functional in the core musculature, while the superior and middle poles function during respiration. When the quadratus lumborum contracts unilaterally, it causes the trunk to bend to the ipsilateral side; when it contracts bilaterally, it provides the ability to bear down and increase intra-abdominal pressure [2]. The multifidi originate on the transverse processes and insert superiorly on the spinous process. They function in segmental motion of the lumbar vertebrae and help extend the spine with bilateral contraction. With unilateral contraction, they cause flexion, ipsilateral side-bending, and contralateral rotation of the spine [2, 3, 5, 6].
The lateral borders of the core are composed of the gluteal muscles as well as the lateral portions of the previously discussed anterior core muscles; the obliques, thoracolumbar fascia, and transverse abdominis. The gluteal muscles consist of the gluteus maximus, medius, and minimus (Table 10.1 and Fig. 10.3). The gluteus maximus originates from the dorsal surface of the sacrum and posterior iliac crest, and inserts on the iliotibial band and gluteal tuberosity of the femur. Its primary motion is hip extension with lateral rotation. The gluteus maximus extends the trunk from a flexed position. The gluteus medius and minimus both originate from the ilium and insert on the greater trochanter of the femur. Their primary motion is hip abduction. The gluteal muscle group as a whole has an important role in dynamic stabilization of the hip, pelvis, and trunk during motion [2, 3, 5].
The thoracolumbar fascia acts as a girdle around the abdomen, and consists of three layers (Fig. 10.3). All three layers—anterior, middle, and posterior—function to maintain stability of the spine and trunk while preventing unwanted motion during movement. The anterior and middle portions are intimately connected to the iliopsoas, quadratus lumborum, and intrinsic back muscles such as the multifidi. The posterior layer is the most superficial layer with connections to the transversus abdominis and latissimus dorsi, connecting the abdomen and the upper limb. The various attachments pulling at the thoracodorsal fascia are instrumental in providing tension, removing slack, and providing stability (Fig. 10.4) [2–5].


Fig. 10.4
The thoracolumbar fascia is a crucial component of the core. It is connected to many muscles and, when they contract, it is pulled tight like a girdle and provides stability. The transverse abdominis muscle is one of the major players in pulling the thoracolumbar fascia tight during contraction. The arrows indicate the manner in which the fascia is pulled during a contraction
The abdominal diaphragm serves as the roof of the core and increases the intra-abdominal pressure by descending. It provides stability during exercise through coordinated breathing.
The pelvic floor acts as the base of the core and aids in increasing intra-abdominal pressure by contracting and ascending. The pelvic floor and diaphragm primarily work in co-contraction with the trunk muscles (Table 10.1 and Fig. 10.5). When a contraction occurs, an increase in abdominal pressure occurs, which, in turn, causes the lumbar spine to stabilize, reducing unwanted movement [2, 3, 5–8].


Fig. 10.5
The pelvic floor and diaphragm are major components in the core and help in providing and maintaining an intra-abdominal pressure during movements. The floor of the core is made up of the pelvic diaphragm muscles. The roof of the core is made up of the diaphragm
10.4 Mechanism of the Core and the Kinetic Chain
To understand the core and its function, the body should be viewed as having an inherent connection among all the different layers of muscles, fascia, and other connective tissues. Patterns of muscle contraction allow for coordinated movement [9]. The motion of an extremity in three-dimensional space occurs freely at the distal portions of the extremity. The proximal limb girdle is anchored to the trunk, while the trunk is anchored to the spine and stabilized by the core. This coupling of musculature of the limbs and trunk allows the body to function as a unit. Thus the motion of one body part influences movement of ipsi- and contralateral distal or proximal portions of the body. These motions are generated from contractile forces within the muscles, which then disperse energy distally and proximally, allowing for motion in a three-dimensional plane. The spine, hip, pelvis, and trunk are the areas of transition of this energy, force, and motion (Fig. 10.6). Therefore, when a force is generated in the lower extremity, the core transmits the force to the trunk. Studies have shown that the generation of forces in the extremities is preceded by unconscious contraction of the core muscles [9, 10]. The core therefore both transmits forces from the extremities proximally and stabilizes the trunk for motion of the limb distally. This coupling of functions makes the core the center of the kinetic chain.


Fig. 10.6
The kinetic chain theory can often be applied to sports that involve explosive motions . In this example of kicking a soccer ball, the power to kick the ball will often develop in the upper extremities, and transmit downward to the leg during the follow-through. The core contracts prior to all these motions, and stabilizes the body to allow for a fluid transition of the upper body momentum to the lower body. When the core is functional and intact, the maximal and coordinated force from these sources can be achieved
The kinetic chain theory, also known as the link theory , describes the connection of musculoskeletal motion in the extremities and trunk. The definition of the kinetic chain, as described in the literature, is the significant interrelationship of muscle activation and translation of forces within the musculoskeletal system [11].
An example illustrating this principle is in the case of a pitcher throwing a fastball. The starting point of the force is the lower extremity; the end point is the upper limb. When the lower limb contracts in the early part of a pitcher’s motion, power is generated in the lower limbs. Prior to start of the pitching stride, the abdominal muscles and back muscles contract, to provide stability. As the pitcher steps forward through his stride, the force is transferred from the lower limbs through the stabilized core, and upwards to the upper extremity where the follow-through is made and the ball is thrown (Fig. 10.7). In an ideal kinetic chain system, no vulnerable points exist where forces can be lost because of instability or weakness. When an interruption in the chain occurs, motion and forces are improperly and inadequately transmitted, and compensation is attempted by other muscle groups. For example, generating the same amount of power to throw a fastball in an athlete with a weak core will lead to straining of the compensatory muscles. As a result, a weak core places a person at greater risk of injury, misalignment, and submaximal performance [4, 9, 10, 12, 13].


Fig. 10.7
In the case of a baseball pitcher, the kinetic chain functions similarly to that of a soccer player; however, the forces develop in the lower extremities initially, and then transmit upward to the upper extremities. The core once again contracts, stabilizes, and allows for an efficient throw
To understand this, it is important to see how patterns of motion are interrupted in patients with extremity pain or low-back pain. These injuries can result in altered neuromuscular control of the core and limbs. Athletes with lower extremity instability (i.e., ankle or knee) have a delayed firing pattern of the gluteus medius (which functions in hip abduction and posterior-lateral trunk stability) and the anterior abdominal muscles [13, 14]. A dysfunction of the neuromuscular control of the core interrupts the kinetic chain. The effect of breakdown in one part of the chain results in abnormal and delayed muscle firing patterns, poor neuronal feedback, and poor proprioception. This, in turn, causes increased instability in the spine and other joints [4, 9, 10, 14, 15].
The core is not only responsible for generating or transmitting force; it functions to provide stability to the spine and other joints . Joints require stabilizing forces from muscles and proprioceptive feedback from neurons for optimal function. The early development of arthritis or joint dysfunction often results from misalignment, unwanted motion, and high shearing stress. A strong core with sufficient endurance and optimal neuromuscular control patterns provides spinal stability [9, 16]. The diaphragm, pelvic floor, internal oblique, external oblique, and transversus abdominis increase intra-abdominal pressure when contracting. This creates tension in the spine and thoracolumbar fascia, reducing laxity in the spine and imparting stability to the lumbar spine [4, 7, 8, 17, 18]. This is analogous to inflating a tire. As air is added to the tire, tension in the walls increases, preventing the tire from sagging, and allowing it to roll firmly and efficiently.
The transversus abdominis and multifidi have been shown to contract prior to movement of a limb. This is thought to provide immediate stability to the core prior to limb movement, occurring through a neuromuscular control feedback mechanism [6, 11]. In cases of injury or in presence of low-back pain, the multifidi and transversus abdominis are inhibited. During muscle contraction of the lower extremity, the transversus abdominis is normally the first to contract. This prevents unwanted trunk movement, and allows the fluid transition of energy/force through the kinetic chain, as described previously. With low-back pain, a delayed activation of the transversus abdominis contraction is noted on electromyography evaluation, relative to limb activation [19, 20].
Pelvic motion is controlled by the paraspinals and anterior abdominal musculature as well as the pelvic attachments of the hip rotators, adductors and abductors and knee flexors and extensors. Thus weakness of the core can create breakdown in pelvic control, leading to breakdown in lower extremity control. Pelvic tilt is important in achieving normal range of motion of the lower extremity and providing an optimal center of balance. The hip rotator muscles are particularly important in dynamic single-leg stance activity such as running, jumping, and throwing [9–11, 21].
Summarily, a strong, balanced, and controlled core is essential in providing stability and proper alignment of the spine, hip, pelvis, and sacrum. Lack of core stability interrupts the physiologic contraction patterns of the kinetic chain as a result of compensatory muscle firing due to alterations in neuronal feedback [1].
For a more comprehensive discussion of the kinetic chain, refer to Chap. 3 of this text.
10.5 Core Dysfunction in Clinical Scenarios
As illustrated thus far, core strength is integral to the dynamic movements of everyday activities and even more so in athletic endeavors. In cases where distal extremity dysfunction develops, there is a greater risk for developing proximal injury due to compensatory mechanisms. A core strengthening program may prevent injury by improving dynamic control of the core and stabilization of motion of the limbs through different planes [7, 22].
Core and hip extensors , primarily the gluteus maximus and medius, play a major role in stabilizing the pelvis during trunk rotation, or when the center of gravity is grossly shifted. The hip abductors function in midstance to stabilize the pelvis, preventing downward inclination (Trendelenburg sign) during single leg stance. When hip abduction weakness occurs, there is an increase in lateral trunk stabilizer firing. Asymmetry in hip muscle strength, flexibility, or endurance may produce delayed firing in the hip extensors and abductors, theorized to predispose to lower extremity injury and low-back pain. This can be attributed to the interruption of neuronal firing patterns, which in turn results in poor muscular control [10]. Hip abductor strength in females athletes has been studied utilizing the Star Excursion Balance Test [23–25] which associates neuromuscular control and decreased lower extremity injury risk.
In cases of injury, such as anterior cruciate ligament (ACL ) dysfunction or ankle instability, the firing patterns of the hip and core muscle are disrupted [14, 15, 26]. In response to an ACL injury or knee instability, the hip flexors and knee extensors display altered contraction patterns, in an effort to reduce stress on the ACL and knee joint. A pattern of increased firing of the hip muscles on the ipsilateral side of an ankle injury has been shown [14]. With a chronic injury or without neuromuscular rehabilitation, this results in poor firing patterns on the ipsilateral side of the injury causing a chronic disruption in the kinetic chain [15].
Patients with low-back pain have been shown to develop an abnormal pattern of muscle contraction [20]. The multifidi and transverse abdominis have been shown to function abnormally when low-back pain is present. During muscle contraction with anterior loading, the transverse abdominis is activated first, preventing unwanted trunk movement and thus allowing the transition of energy and forces in a normal pattern of the kinetic chain. However, patients with low-back pain have demonstrated delayed activation of the transverse abdominis relative to the limbs [6–8, 12, 27].
Core strengthening for low-back pain requires focus on the muscles associated with twisting motions and repetitive flexion/extension motions. People with poor musculature control of the trunk and hips are often at greater risk for developing low-back pain due to abnormal or unstable transferences of forces when motion is occurring. Correction of abnormal neuromuscular control with core stability exercises can improve function [6, 27, 28]. Focused core stability exercise appears to be more effective in decreasing pain and improving general function in the short term versus general exercise in chronic low back pain [29] Several studies have shown significant improvement in low back pain in athletes after core stability exercise, however, further research is needed for high quality support [30].
10.6 Assessing the Core Musculature
The comprehensive evaluation of an athletes’ core stability is important and should include 5 components: range of motion, strength, endurance, motor control, and function [31]. There are both static and dynamic measures of assessing the core musculature. It is important to note that athletic performance is dynamic, intermittent, and oscillates in intensity and energy requirements. Therefore a static core muscle test may not represent a comprehensive assessment of the core in a healthy athlete [32]. Currently, there are no standardized means of dynamically assessing the core [32].
No published consensus definition or measurement criteria for core stability exist. In fact, there are currently at least 35 published tests developed to assess core stability. As a group, it has been determined that the core endurance tests have the highest intra-rater reliability [31].
10.6.1 Neutral Spine Position
Neutral spine position should be maintained during examination (Fig. 10.8). Neutral spine position represents normal alignment of the spine, with all three physiological curvatures in place. With regard to core stability, neutral spine position should also describe the point of pain-free positioning, where maximal power can be obtained and balance can be preserved. Maintenance of the neutral spine position is important in day-to-day function and is the position in which training should occur. Neutral spine positioning allows for muscle training and development without increasing load, shear, and damage on the spine and other joints. The eventual goal of core stability training is to improve strength, endurance, balance, and the neuronal output of the trunk and limbs while maintaining a neutral spine position [4].


Fig. 10.8
Maintenance of neutral spine positioning during core assessment is essential. (a) kyphotic posture; (b) neutral spine posture; (c) lordotic posture
10.6.2 Flexibility
The examiner should begin by assessing the patient’s posture and gait. Flexibility assessment should include examination of both the active and passive range of motion of the trunk, hips, and pelvic girdle, all the while assessing for laxity and stability of joints (Table 10.2; Fig. 10.9). It is important to compare side-to-side for asymmetries. Examination may begin by observing and palpating the spine to assess for anatomical alignment or deformity. The spine should be evaluated in the neutral spine position, which is the position of minimal loading on the spinal joints. Range of motion of the lumbar spine should be assessed in the functional planes of flexion, extension, side bending, and rotation (Figs. 10.10 and 10.11). Hip extension, flexion, internal and external rotation should be evaluated (Fig. 10.12). The major components under scrutiny throughout the evaluation are loss of range of motion or clinical instability, which is defined as the loss of the ability to maintain normal anatomical positions [16].
Table 10.2
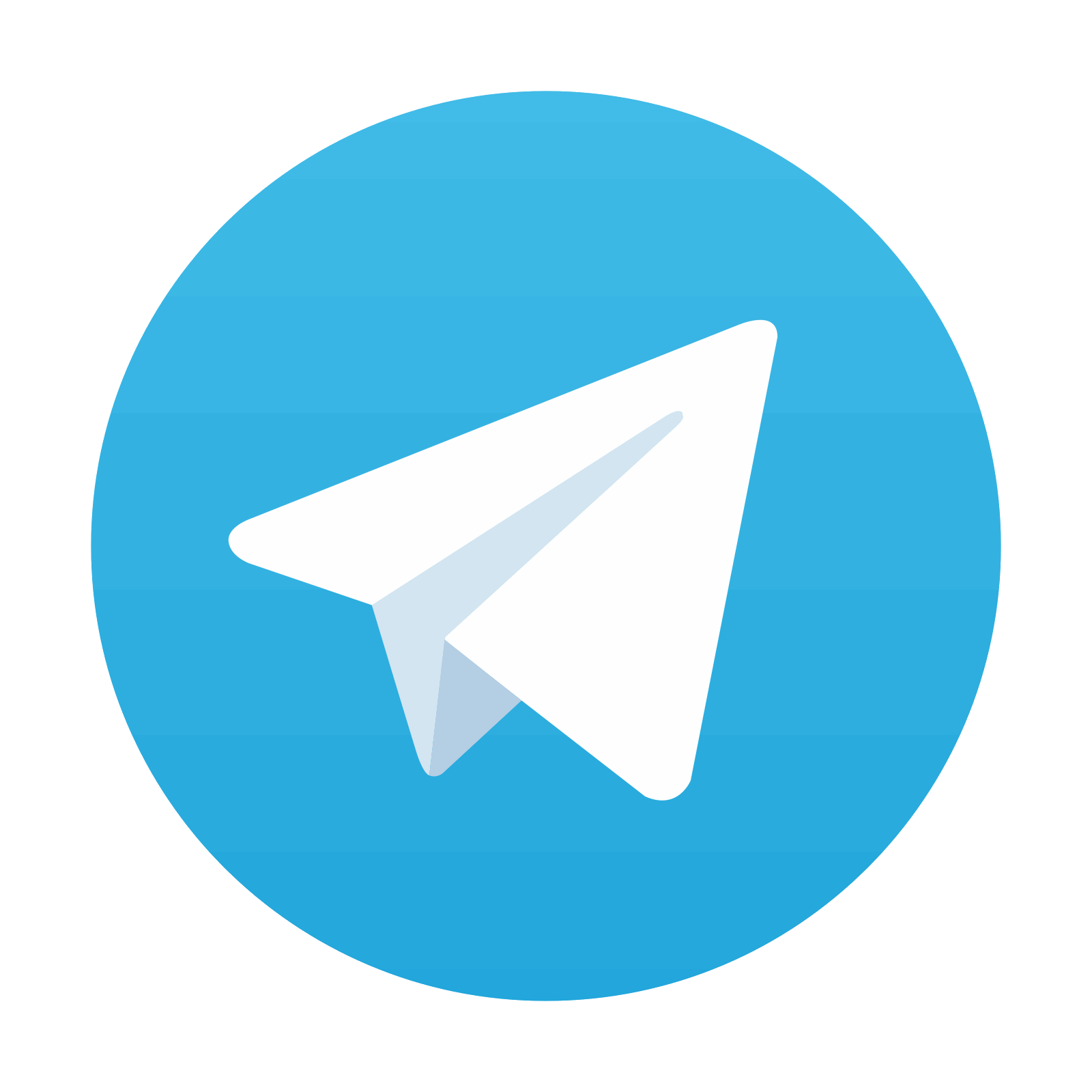
Screening tests for stability and flexibility
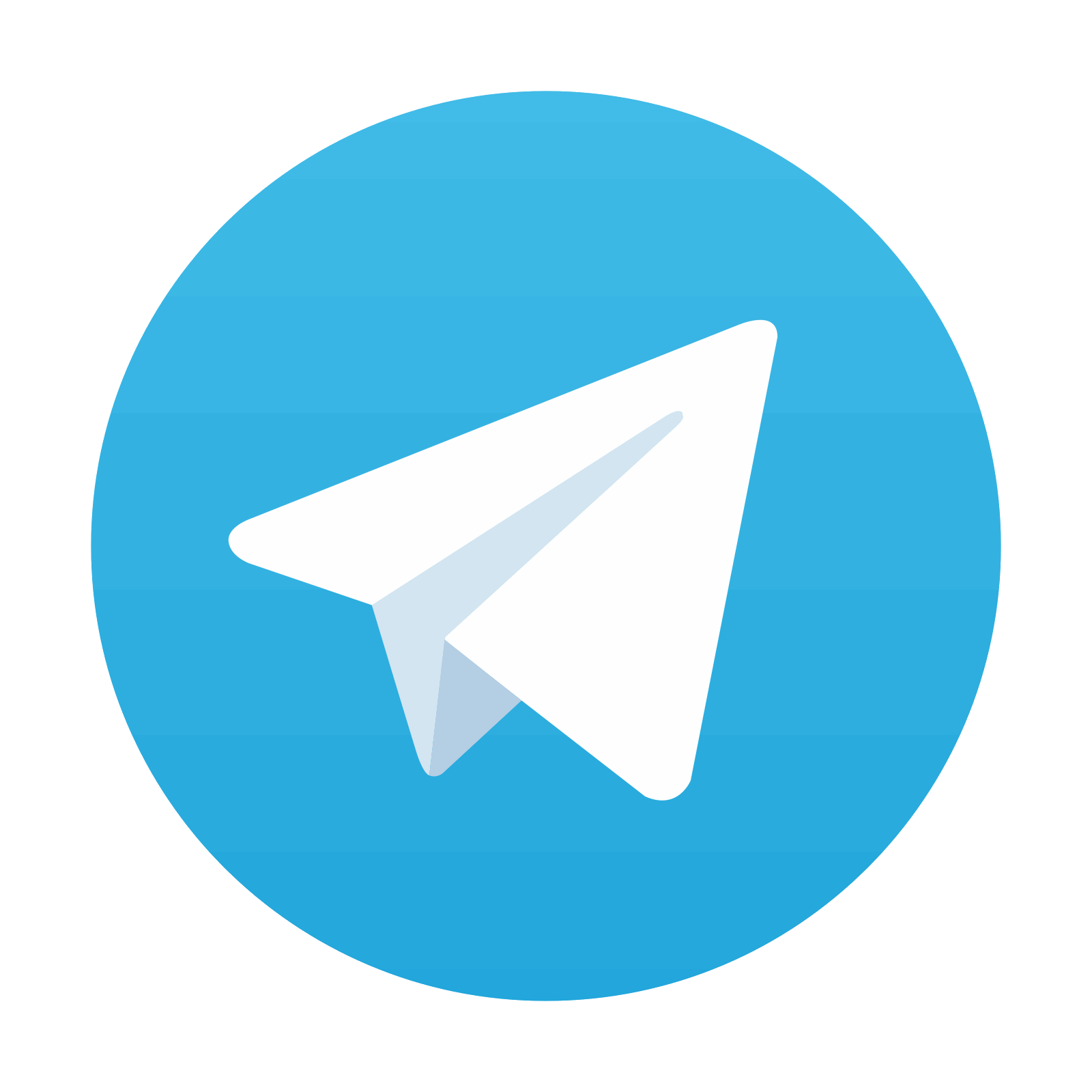
Stay updated, free articles. Join our Telegram channel

Full access? Get Clinical Tree
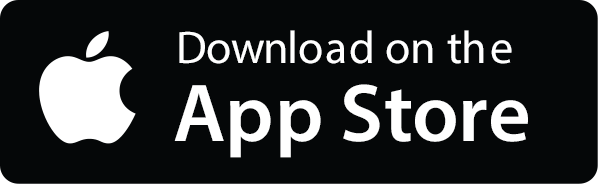
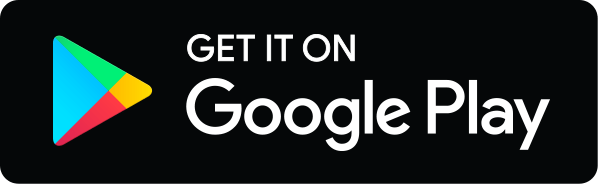
