Recent studies employing functional neuroimaging provide new insights into the elusive construct of fatigue. Studies have been conducted primarily in persons with multiple sclerosis (MS) and chronic fatigue syndrome (CFS). These studies outline the key role of the basal ganglia and frontal lobes in understanding the neural mechanisms associated with fatigue. The lack of a relationship between self-reported fatigue and objectively measured fatigue is outlined, and new functional imaging paradigms may lead to significant advances in relating cognitive fatigue to functional cerebral activity.
Fatigue is one of the most common symptoms observed in numerous medical populations. It has been conceptualized as both a symptom and a syndrome. As a symptom, fatigue has many causes ranging from medical conditions (eg, infections, cancer, thyroid abnormalities, coronary heart disease), insults to the brain (eg, traumatic brain injury [TBI], MS, Parkinson disease [PD], stroke), psychiatric disorders (eg, depression, somatization), medications (eg, antihistamines), and even unhealthy lifestyles. Little is known about the mechanisms that cause fatigue in these conditions. As a syndrome or disease, fatigue has been recognized as the major part of a group of “unexplained” illnesses, such as CFS, neurasthenia, Da Costa syndrome, and so on, in which there is little understanding of its causes.
Fatigue is not always seen as a pathologic feature of a medical condition, as it is also a common problem affecting otherwise healthy individuals. It has been observed as a common problem affecting up to 40% of the population based on community-based samples. It is often one of the primary complaints in visits to both primary care physicians and hospitals and can have a significant negative effect on quality of life.
Fatigue is not a unitary concept in terms of both its definition and its measurement. Although the multidimensional nature of fatigue has been recognized for more than 100 years, definitions of fatigue have largely been tied to its subjective nature. For instance, the Multiple Sclerosis Council for Clinical Practice Guidelines defines fatigue as “a subjective lack of physical and/or mental energy that is perceived by the individual or caregiver to interfere with usual and desired activities.” However, others have conceptualized fatigue through direct behavioral observation, typically viewed as a performance decrement over time (ie, objective performance). Given these 2 most common conceptualizations of fatigue (feeling state vs performance decrement), the most common finding observed in more than 100 years of inquiry is that these 2 metrics of fatigue do not correlate (see DeLuca ). In addition, fatigue has also been divided into other components such as central and peripheral fatigue or as cognitive and physical (or motor) fatigue.
The measurement of fatigue only adds to this confusion. A remarkable number of fatigue questionnaires have been developed over the years to assess subjective fatigue. Such inventories range in length from single-item scales (eg, Borg ), to multidimensional scales, which claim to assess various dimensions of fatigue (eg, physical vs mental). Most of the items on these scales have a good deal of item similarity and correlate highly with each other, whereas some items claim to be specific to a target audience. One of the major problems, however, with most of the so-called fatigue questionnaires is the construct contamination that mars the validity of such scales. For instance, fatigue inventories often include items on sleepiness and cognition, both of which may not be associated with fatigue (see DeLuca for a review).
In addition to the nebulous qualities of its definition and measurement, the pathophysiology of fatigue remains elusive. A number of pathophysiological mechanisms have been proposed, including immune system dysregulation, impaired nerve conduction, neuroendocrine and neurotransmitter dysregulation, and energy depletion. In addition, a great deal of research has focused on damage to the central nervous system (CNS) as the major contributing factor to fatigue. In particular, research using neuroimaging has begun to provide evidence that several distinct areas of the CNS may be specifically involved in fatigue.
While traditional magnetic resonance imaging (MRI) provides images of brain structure, functional imaging techniques such as functional MRI (fMRI) and positron emission tomography (PET) are techniques that assess functional changes in the brain. fMRI primarily uses the blood oxygen level-dependent (BOLD) contrast as an index of neuronal activity. PET measures glucose metabolism with the aid of radioactive tracers.
The major thrust of this article is on what we are beginning to learn about fatigue from studies using functional neuroimaging; though, for completeness, we begin with a brief review of the structural imaging studies.
A search of the functional imaging literature on fatigue yielded studies almost exclusively with 2 populations: MS and CFS ( Fig. 1 ). Therefore, the primary focus of this article is to review the existing neuroimaging research on fatigue in MS and CFS.
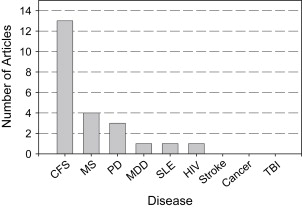
Fatigue in MS
Fatigue is one of the most common symptoms reported by patients with MS. It frequently presents as the primary symptom at the time of diagnosis, and patients often report fatigue as the most troubling feature of the disease. Fatigue has been shown to be significantly associated with reduced activities of daily living, and it negatively affects employment and social relationships in persons with MS. Fatigue in MS tends to be associated more with affect state than with both measures of disease activity (eg, EDSS) and other neurologic metrics (eg, neuroimaging).
Fatigue in MS Using Structural MRI Studies
A number of studies have been conducted during the past 10 years examining the relationship between self-reported measures of fatigue and structural damage in MS using MRI. Most of these studies focused on using self-report of fatigue in groups of fatigued and nonfatigued MS participants and measured cerebral abnormalities, usually T2- and T1-weighted total lesion load in discrete cerebral areas and/or cerebral atrophy. The earlier studies conducted found no significant relationship between these indices of pathology and self-reported fatigue. To illustrate, Mainero and colleagues associated increased fatigue with disruption of the blood–brain barrier, which would allow cytokines to infiltrate the CNS. They hypothesized that the presence of gadolinium-enhancing (Gd+) lesions on MRI scans would be indicative of this blood–brain barrier disruption. Yet no relationship was found between the number and volume of Gd-enhancing lesions and fatigue severity at any time point studied.
Although most studies using MRI have not found a relationship between structural indices and fatigue, a few studies have reported positive results. For example, Marrie and colleagues (2005) used a longitudinal design to examine the relationship between brain atrophy changes and subjective fatigue during 8 years. Although no relationship between pathology and fatigue was observed after 2 years, a significant change was observed in fatigue effect during the 8-year period. This relationship was maintained after adjusting for disability, mood, and the subjects’ baseline MRI.
More advanced MRI techniques, such as diffusion tensor imaging (DTI) and magnetization transfer, have also failed to show a significant relationship with self-reported fatigue in persons with MS.
Functional Neuroimaging of Fatigue in MS
The most common functional imaging techniques to study fatigue in MS are PET and fMRI. Roelcke and colleagues used 18F-fluorodeoxyglucose PET (FDG-PET) to measure cerebral glucose metabolism in 47 participants with MS. Compared with MS subjects without fatigue, the fatigued MS group showed significant hypometabolism in the putamen, prefrontal and premotor cortex, and the right supplementary motor area. The Fatigue Severity Scale (FSS) scores were negatively correlated with glucose uptake in the right prefrontal cortex (PFC). Hypometabolism in the fatigued MS group extended from the rostral putamen toward the lateral head of the caudate nucleus. In contrast, the fatigued MS group showed elevated glucose metabolism in the anterior cingulate and the cerebellar vermis. The authors concluded that dysfunctional cerebral activity in both the basal ganglia and prefrontal regions is responsible for fatigue in persons with MS.
The primary technique employed to examine functional cerebral activity of fatigue in persons with MS is fMRI. Fillipi and colleagues divided their MS sample into fatigued and nonfatigued groups based on the FSS and measured BOLD activation during performance of a motor task. They reported that the MS group with fatigue had significantly less functional cerebral activation in several regions involved in motor planning and execution compared with that in the MS group without fatigue. These regions included the ipsilateral precuneus, ipsilateral cerebellar hemispheres, contralateral middle frontal gyrus, and contralateral thalamus. A negative correlation between the FSS score and cerebral activation in the contralateral thalamus was also observed. The thalamus is known to be a major relay station between motor and prefrontal regions and basal ganglia. Filipi and colleagues hypothesized that these findings suggested a disruption of cortical–subcortical circuits associated with functional activity between the thalamus, basal ganglia, and motor and prefrontal regions of the frontal lobes. Although most regions of the brain showed hypoactivity, the fatigued MS group did show increased activation in the anterior cingulate relative to that in the nonfatigued MS group. The authors suggested that anterior cingulate recruitment in the fatigued group may represent a “compensatory mechanism” because of the increased effort required to perform the task. These authors also conducted an analysis of structural MRI lesions and found no significant differences in lesion load quantity between the fatigued and nonfatigued MS groups. Taken together, such data suggest that functional cerebral changes in the brain may be more sensitive than structural imaging in identifying the cerebral underpinnings associated with fatigue in persons with MS.
All of the studies reviewed thus far have used subjective report as the measure of fatigue in fMRI studies. A more recent approach is to assess fatigue objectively, by inducing cognitive fatigue during fMRI acquisition and examining the brain regions associated with such fatigue. Successful attempts at objective measurement of cognitive fatigue have focused on decreased behavioral performance in tasks requiring sustained mental effort. This approach is similar to that typically thought of in the motor fatigue literature, where fatigue has been defined as a failure to maintain a required force or output of power during sustained or repeated muscle contraction. There is indeed considerable support for such an objective assessment of cognitive fatigue in clinical populations (see DeLuca, for a review). Two recent studies used this design of objectively measuring cognitive fatigue during fMRI acquisition in persons with MS.
DeLuca and colleagues attempted to induce cognitive fatigue in persons with MS and in healthy controls (HCs) by administering 4 trials of a sustained attention task ie, modified Symbol Digit Modalities Task or (mSDMT) during fMRI acquisition. It was hypothesized that participants with MS would show a greater increase in cerebral activity while performing the mSDMT across time compared with that in HCs. Importantly, based on the various studies in HCs, DeLuca and colleagues hypothesized observing a decrease in cerebral activation to repeated administration of the mSDMT tasks over time in healthy individuals (eg, Petersen and colleagues; Raichle and colleagues; Koch and colleagues ). That is, DeLuca and colleagues operationally defined “cognitive fatigue” as an increase in cerebral activation across the 4 trials of a processing-speed task in individuals with MS, relative to that in the control group, which would exhibit a decrease in brain activity across time. DeLuca and colleagues found no group differences in performance accuracy across the 4 trials of the cognitive task. The MS group showed increased cerebral activation in key areas of the brain compared with that in the healthy group, which showed decreased activation ( Fig. 2 ) . These regions included the precuneus, superior parietal lobe (BA 7), medial/orbital frontal gyrus, inferior parietal lobe, and the caudate in the basal ganglia. One limitation of the DeLuca and colleagues study was that subjective fatigue was not assessed, so it was unclear how these results would change if differing levels of subjective fatigue were taken into account.
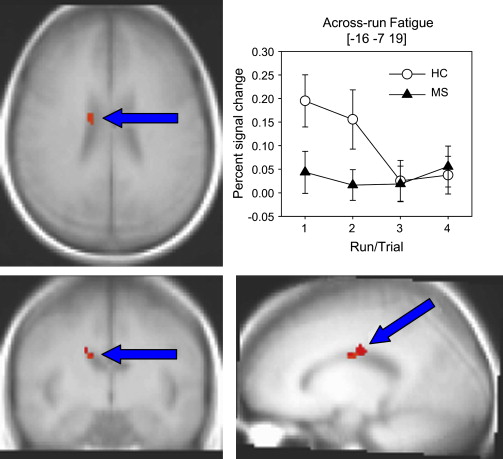
What was particularly important about the DeLuca and colleagues study was that it was specifically designed to test the current model of brain mechanisms responsible for central fatigue proposed by Chaudhuri and Behan. Chaudhuri and Behan hypothesize that “central fatigue” is a function of the nonmotor function of the basal ganglia. These authors suggest that “alterations in the normal flow of sequential activation within the basal ganglia system affecting the neural integrator and the cortical feedback by the associated loop of the striato-thalamo-cortical fibers is a possible mechanism of central fatigue …” (p40). The findings of the DeLuca and colleagues study of altered cerebral activation within the basal ganglia and frontal lobes during cognitive fatigue lend direct support to the Chaudhuri and Behan model.
A second study was recently published using objective behavioral performance to measure fatigue in persons with MS using fMRI. Tartaglia and colleagues hypothesized that induced cognitive fatigue could alter cerebral activation patterns associated with motor performance. Specifically, they hypothesized that fatigue experienced by individuals with MS may be related to “greater demands being placed on diminishing functional neural circuits, resulting in reduced capacity to respond to new demands.” Tartaglia and colleagues examined BOLD activation during an experiment that had 3 stages. First, subjects performed a motor task (stage 1); then they performed a challenging cognitive task (the paced auditory serial addition test PASAT; stage 2); finally, they performed the motor task again (stage 3). The HC group showed no significant difference in cerebral activation during the motor task when the motor task in stage 1 was compared with the same task in stage 3 (ie, pre- vs post-PASAT). The MS group, however, showed increased activity in multiple regions after performance of the PASAT (that is, when the motor task in stage 3 was compared with the same task in stage 1). These included the bilateral cingulate gyrus, the post-central gyrus, and the right PFC. In fact, although the HCs showed decreased activation in the motor task after PASAT performance (ie, stage 3 vs stage 1), in the MS group the activation in all recruited areas was increased. The authors concluded that induced cognitive fatigue could alter brain activation in an unrelated motor task, resulting in increased cerebral activity in persons with MS. One limitation of the study is the absence of a nonfatigued MS group, making it difficult to determine whether these findings were truly due to fatigue or to some other disease-related process.
In summary, studies using structural MRI have yielded mixed results at best in identifying the underlying cerebral structures associated with fatigue in MS. Functional imaging, particularly fMRI, has been much more successful. Although most studies have used subjective report as the primary index of fatigue, a few have measured fatigue using objective performance during the imaging session.
Chronic fatigue syndrome
CFS is a heterogeneous illness characterized primarily by severe and debilitating fatigue as well as infectious, rheumatologic, and neuropsychiatric symptoms. A number of structural and functional imaging studies have been conducted on individuals with CFS. Given that fatigue is the defining characteristic of CFS, one could expect that the neuroimaging studies conducted would reveal much about fatigue itself. However, it should be recognized that some of the differences in brain structure and function between individuals with CFS and HCs might not be related to fatigue but to differences in subject characteristics (eg, psychopathology). Thus, one should be cautious in attributing group differences in functional imaging to effects of fatigue based on simple comparisons between individuals with CFS and HCs. Only studies that measure fatigue and use this measurement in the imaging data can be used to make inferences about brain mechanisms involved in fatigue in CFS. Unfortunately, only a handful of recent studies have employed this approach in CFS. Most of the imaging studies have simply compared brain structure or function in patients with CFS to those without CFS and assumed that the differences are related to fatigue.
Structural Neuroimaging in CFS
The early studies in CFS focused primarily on structural imaging. Although several early studies showed an increased number of white matter hyperintensities on MRI in CFS versus HCs (eg, Buchwald and colleagues; Natelson and colleagues ), several other studies did not (eg, Schwartz and colleagues; Cope and colleagues ). Lange and colleagues found significantly more subcortical white matter hyperintensities in a group of CFS subjects without psychiatric illness (concurrent or historic) compared with those in the CFS group with concurrent psychiatric illness. A similar pattern of results was reported by Greco and colleagues.
Cook and colleagues divided individuals with CFS into those with cerebral abnormalities and those without and found that CFS participants with cerebral abnormalities reported more impairments in physical functioning on the short-form 36-item health survey than those of CFS subjects without cerebral abnormalities. Measuring cerebral volume using MRI, Lange and colleagues found that CFS participants had larger lateral ventricle volumes compared with those of HCs. Okada and colleagues reported significantly reduced gray matter volume in prefrontal areas, bilaterally in their CFS group. Gray matter volume in the right PFC was correlated with the severity of self-reported fatigue, suggesting a prominent role of the PFC in the phenomenology of fatigue. De Lange and colleagues have reported that this reduction in gray matter volume can be partially reversed by cognitive-behavioral therapy.
Functional Neuroimaging in CFS
Using single photon emission computerized tomography (SPECT), Schwartz and colleagues found increased perfusion in the lateral frontal cortex and lateral and medial temporal cortex in their CFS group. Increased perfusion using SPECT in CFS has also been observed in the right thalamus and basal ganglia (pallidum and putamen). Costa and colleagues, however, reported decreases in perfusion in the brainstem of their CFS sample using SPECT. Although these studies showing systemic differences in blood flow are of interest, their role in fatigue is unclear. In contrast, Fischler and colleagues examined whether there was a relationship between cerebral perfusion using SPECT and physical or mental fatigue in persons with CFS. No relationship between fatigue and cerebral perfusion was found.
A few studies in CFS using PET have been published. Using 18-FDG-PET, CFS subjects showed decreases in cerebral metabolism in the frontal lobes. Siessmeier and colleagues reported abnormalities in the anterior cingulate gyrus/mesial frontal cortex and orbital frontal cortex in about half of their CFS sample. However, no significant relationships were found between reductions in glucose metabolism and fatigue in CFS.
Another approach has been to use a challenging task that one might expect would induce fatigue and to investigate the pattern of activity during performance of this task in individuals with CFS relative to that in HCs. This approach has shown CFS to be associated with more widespread and diffuse regional cerebral blood flow in frontal, temporal, and thalamic regions relative to that in controls using SPECT. Using fMRI, Lange and colleagues used a challenging cognitive task and found that persons with CFS showed more diffuse and bilateral activity than that of HCs. Although HCs showed left-lateralized activity in frontal and parietal regions, individuals with CFS showed bilateral activity in frontal and parietal regions (see also Lange and colleagues ). Other work has underscored the importance of the right prefrontal areas, showing that as the difficulty of a working memory task increased, these areas are recruited. All of these studies that used challenging tasks as the experimental paradigm show group differences between individuals with CFS and controls. However, it is unclear whether these differences have anything to do with fatigue itself, as no specific relationship with measures of fatigue was made.
More recent studies in CFS have begun to explicitly include measures of fatigue in the analysis of functional imaging data. Cook and colleagues demonstrated a relationship between subjective fatigue and functional brain activity using fMRI while performing a cognitive task (modified PASAT) in persons with CFS. They found that subjective reports of fatigue after task performance correlated positively with brain activity in several areas including the cerebellum (vermis), the cingulate region (middle and posterior), inferior frontal gyrus, superior temporal gyrus, parietal regions, and the hippocampus. Tanaka and colleagues used a similar approach but looked at brain activity associated both with a difficult, fatiguing, visual search task and activity associated with a completely extraneous, task-irrelevant change in auditory stimulation using fMRI. The functional activity associated with the search task did not differ significantly between the CFS and control groups nor did it correlate with subjective ratings of fatigue. However, the CFS group showed significantly less cerebral activity than that of the HC group during the task-irrelevant change in auditory stimulation, and the activity was correlated with subjective ratings of fatigue. The authors suggested that CFS subjects may have less processing “capacity” and, therefore, have less ability to process task-irrelevant stimuli than that of HCs.
A novel approach to the study of fatigue in persons with CFS was adopted by Caseras and colleagues, who analyzed the BOLD response recorded while persons with CFS were watching either a fatigue-inducing scenario (video clip) or an anxiety-inducing scenario. Following each scenario, subjects rated their feelings of fatigue and anxiety. Results showed that the fatiguing clips induced greater feelings of fatigue than the anxiety-clips in both the CFS and HC groups ( P <.001) and that the CFS group was more fatigued than the control group ( P <.001). Moreover, when watching the fatiguing clips, the CFS group showed increased activity in the cerebellum and occipito-parietal regions bilaterally extending toward the cingulate gyrus, the left hippocampal gyrus, and the left caudate nucleus. For the HC group, increased activity was seen in the right dorsolateral and bilateral medial PFC (extending toward the anterior cingulate), right insula, and right caudate nucleus when watching the fatiguing clips than the anxiety-inducing clips. Importantly, fatigue was associated with activity in the basal ganglia (caudate) for both the CFS and HC groups. In addition, the CFS group showed more activation in the posterior regions and those associated with emotion (cingulate), whereas the HC group showed more activity in the frontal regions associated with executive control. Lastly, the regions activated in the CFS group in the Caseras and colleagues study showed broad overlap with those reported by Cook and colleagues, even though the 2 studies used widely different paradigms and analyses.
In summary, although there is evidence for structural changes in the brain for persons with CFS, including white matter damage and gray matter volume, it is unclear how these changes are associated with fatigue per se. Functional neuroimaging studies appear to offer greater promise in understanding the cerebral mechanisms of fatigue in CFS, although caution is needed in interpreting functional imaging studies that just display group difference, as these characteristics may not be directly related to fatigue. When fatigue is specifically assessed and related to functional brain activity, areas that appear to be significantly associated with fatigue include the basal ganglia, the cingulate, the cerebellum, and the parietal cortex. Interestingly, some of these structures, particularly the basal ganglia, support the Chaudhuri and Behan, model of fatigue. It is likely that further investigation of this set of brain regions may prove fruitful in our understanding of fatigue in CFS.
Functional neuroimaging in other clinical populations
As illustrated in Fig. 1 , there are only about a handful of studies examining the association between subjectively reported fatigue and neurocognitive measures in several other clinical populations, including systemic lupus erythematosus (SLE), major depressive disorder (MDD), Parkinson disease (PD), and TBI. Overall, these studies have shown mixed results. For example, when a correlation was sought between fatigue (using the FSS) and cerebral blood flow (CBF) in SLE (using SPECT), no brain region showed such a correlation. This was found despite the fact that subjects with SLE showed reliable disturbances in CBF compared with those of controls. No relationship between subjective fatigue and FDG-PET abnormalities was observed in a small sample of persons who were human immunodeficiency virus (HIV) positive. However, in MDD, fatigue was found to covary with measures of functional brain activity in 1 study. Brody and colleagues examined the association between change in depressive symptoms and change in regional brain metabolism using PET from before to after treatment. Decreases in fatigue from pre- to post-treatment correlated with decreases in ventral PFC activity (metabolism) bilaterally. That is, as fatigue lessened (improved), there was less activity in ventral PFC. This is a promising finding but must be interpreted with caution, since a fairly liberal activation threshold was used ( P <.05, not corrected for multiple comparisons).
In PD, 2 studies have looked at the availability of dopamine in the basal ganglia, using SPECT. Schifitto and colleagues compared fatigued PD subjects to nonfatigued patients and found that though fatigued patients had significantly more neurologic impairment (as measured by each subset of the Unified Parkinson Disease Rating Scale and Hoehn-Yayr scale), there was no difference in their measure of striatal dopamine transporter density, [ 123 I]-β-CIT SPECT in the striatum, caudate, or putamen. Weintraub and colleagues found a trend for a correlation ( P = .11) between their measure of fatigue in PD subjects (the profile of mood states) and the distribution of striatal dopamine transporter in the left anterior putamen. Although this association was clearly not significant, the cohort of PD patients in this study were not reliably more fatigued than the HC group, suggesting that perhaps more robust effects would be found with more fatigued participants. Finally, Abe and colleagues investigated the correlation between cerebral perfusion, using SPECT, and fatigue (using the FSS) in PD subjects. These researchers found a significant correlation between FSS and perfusion in the frontal lobes such that more fatigue (higher FSS scores) was associated with less frontal perfusion.
Using a similar paradigm to that employed by DeLuca and colleagues in their fMRI study of fatigue in MS, Kohl and colleagues measured cognitive fatigue objectively during fMRI in persons with TBI. Briefly, a cognitive task requiring sustained attention (ie, SDMT) was administered 3 times for blocks (or “runs”) lasting approximately 5 minutes during fMRI acquisition for both TBI and HC subjects. Based on the logic of the DeLuca and colleagues study in MS (see also above), Kohl and colleagues hypothesized that TBI participants would show an increase in cerebral activity on the mSDMT in areas of the brain hypothesized to be associated with fatigue, whereas HCs would show decreased activation. That is, Kohl and colleagues operationally defined “cognitive fatigue” in 2 ways: an increase in cerebral activation from the first half to the second half of the 3 runs of the processing-speed task in individuals with TBI compared with that in HCs (within-run fatigue) and an increase in cerebral activation across the 3 runs of the processing-speed task (across-run fatigue). Behaviorally, no significant difference in performance accuracy was observed between the TBI and HC group, although the TBI was significantly slower in performing the task. However, in the fMRI data, effects consistent with within-run fatigue were found in the middle frontal gyrus (BA 10), the superior parietal lobe (BA 7), and the basal ganglia (the putamen). Effects consistent with across-run fatigue were also seen and were confined to the anterior cingulate (BA 32). These data were interpreted as supporting the Chaudhuri and Behan model of central fatigue.
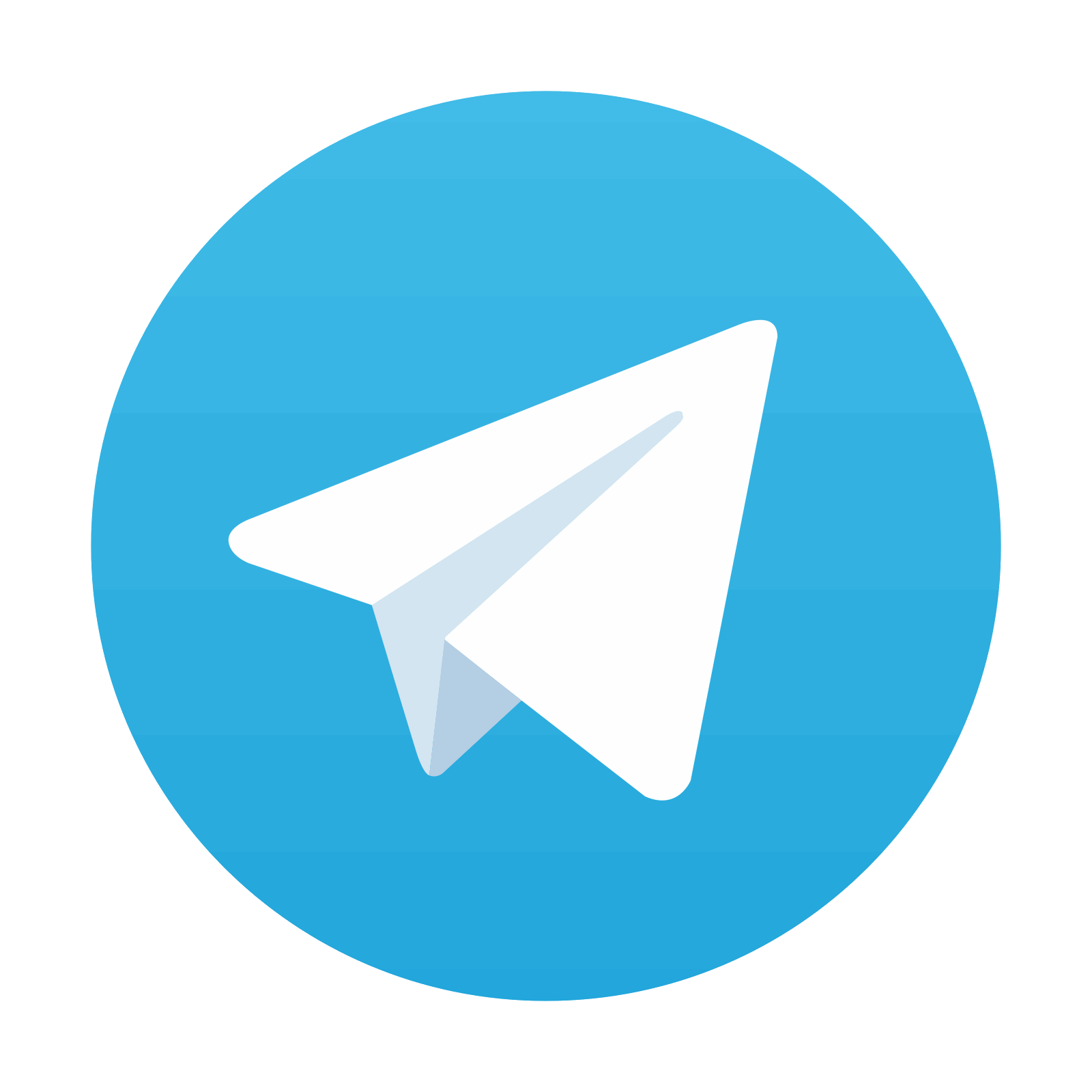
Stay updated, free articles. Join our Telegram channel

Full access? Get Clinical Tree
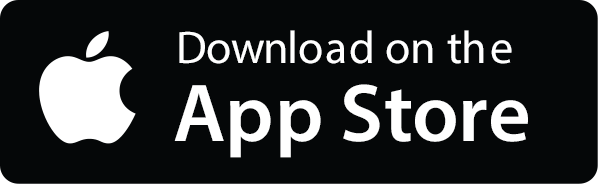
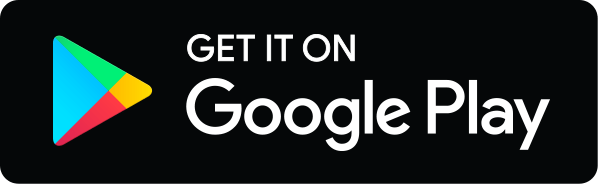