The progressive perilunar instability model described by Mayfield is still used to predict the pattern of injury. Diagnosis of injury and clinical and radiographic findings depend on the pattern of injury. Open procedures are preferred for anatomic reduction after initial closed reduction is performed for acute injuries. A dorsal, volar, or combined dorsal/volar approach may be necessary and is often decided by surgeon preference. Loss of motion and diminished grip strength are common consequences despite appropriate treatment. Successful outcomes depend on time to treatment, open or closed nature of injury, extent of chondral damage, residual instability, and fracture union.
Key Points
- •
Perilunate injuries are rare; unfortunately, the consequences of improper recognition and inappropriate management can be debilitating.
- •
Injuries occur as a result of a high-energy load leading to carpal disruption and progressive ligamentous and bone injury.
- •
Loss of motion, diminished grip strength and post-traumatic arthrosis are common consequences despite appropriate treatment.
- •
Successful outcomes depend on time to treatment, open or closed nature of the injury, extent of chondral damage, residual instability, and fracture union.
Introduction
Perilunate injuries are rare; unfortunately, the consequences of improper recognition and inappropriate management can be debilitating. Injuries occur as a result of a high-energy load leading to carpal disruption and progressive ligamentous and bone injury. The most commonly described events include falls, sports-related injuries, and motorcycle and motor vehicle accidents. Mayfield and colleagues observed that cadaveric wrists loaded in hyperextension, ulnar deviation, and carpal supination reproduced the injury. Progressive failure of ligament and bone contributes to predictable patterns with multiple variants. Injuries are divided into perilunate dislocations and perilunate fracture dislocations . Deformity can sometimes be subtle, and spontaneous reduction has been reported. Prompt recognition is a challenge, and up to 25% of these injuries are missed on initial presentation. Early and accurate diagnosis is critical to prevent progressive pain and dysfunction. Treatment involves an attempt at closed reduction followed by surgical intervention to restore carpal alignment and stability. Delay is associated with poor outcomes, including posttraumatic arthritis, decreased motion and grip strength, median nerve impairment, disability, loss of wages, and even attritional rupture of flexor tendons. This article reviews carpal anatomy and kinematics, classification systems, treatment options, and expected outcomes.
Introduction
Perilunate injuries are rare; unfortunately, the consequences of improper recognition and inappropriate management can be debilitating. Injuries occur as a result of a high-energy load leading to carpal disruption and progressive ligamentous and bone injury. The most commonly described events include falls, sports-related injuries, and motorcycle and motor vehicle accidents. Mayfield and colleagues observed that cadaveric wrists loaded in hyperextension, ulnar deviation, and carpal supination reproduced the injury. Progressive failure of ligament and bone contributes to predictable patterns with multiple variants. Injuries are divided into perilunate dislocations and perilunate fracture dislocations . Deformity can sometimes be subtle, and spontaneous reduction has been reported. Prompt recognition is a challenge, and up to 25% of these injuries are missed on initial presentation. Early and accurate diagnosis is critical to prevent progressive pain and dysfunction. Treatment involves an attempt at closed reduction followed by surgical intervention to restore carpal alignment and stability. Delay is associated with poor outcomes, including posttraumatic arthritis, decreased motion and grip strength, median nerve impairment, disability, loss of wages, and even attritional rupture of flexor tendons. This article reviews carpal anatomy and kinematics, classification systems, treatment options, and expected outcomes.
Wrist biomechanics
The wrist is a diarthrodial joint comprised of 8 carpal bones arranged in 2 rows. The geometry of the carpal bones and their attachments allows motion in 2 primary planes: flexion-extension and radio-ulnar deviation. The flexion-extension arc is derived from roughly equal motion through the radiocarpal articulation and the midcarpal articulation. Radio-ulnar deviation is similarly distributed with the radiocarpal joint contributing 40% and the midcarpal joint contributing 60%.
There are several theories to explain carpal kinematics and function. Bryce described carpal bone motion based on radiographs of his own wrist. Since then, several tools have been used to evaluate wrist motion, including uniplanar radiographs, measurement of inserted wires into individual carpal bones, fluoroscopy, cineradiography, stereoscopic measurement, light emitting diodes, and 3-dimensional computed tomographic (CT) imaging.
In the early twentieth century, Navarro proposed that the carpus was arranged in 3 rigid, vertical columns. The central column (lunate and capitate) provided a base for axial loading. The medial column (triquetrum, pisiform, and hamate) created a pivot point around which the carpus could rotate. The lateral column (scaphoid, trapezium, and trapezoid) acted as a mobile linkage column. Taleisnik modified the 3-column concept in 1976 by including the trapezium and trapezoid in the central column while eliminating the pisiform from the medial column. In 1988, Weber introduced the longitudinal column theory of a force-bearing column and a control column. The force-bearing column bore weight and consisted of the radius, lunate, proximal two-thirds of the scaphoid, capitate, and trapezoid. The control column provided control during motion and was comprised of the ulna, ulnocarpal complex, triquetrum and hamate, and the fourth and fifth metacarpal bases. The column theories provided a model to account for axial load transmission from the hand to forearm; however, they failed to explain the coupled motion between the proximal and distal rows. Additionally, it did not account for the small but significant independent motion present between carpal bones.
The row theory was introduced in the 1980s and suggested that the proximal and distal rows behaved as separate functional units. The distal row was fixed to the metacarpal bases with very little intercarpal motion, creating a distal hand segment. The forearm comprised the proximal stable segment. The proximal carpal row was the intercalated segment, with freedom of motion caused by the bony configuration and ligamentous attachments. The scaphoid was thought to serve as a linkage and strut mechanism, which maintained the integrity of the intercalated segment under axial loading forces. To their credit, Linscheid and colleagues, years earlier, recognized that no muscles or tendons insert on the proximal carpal row and, as a result, proposed that the scaphoid, lunate, and triquetrum acted as an intercalated segment between the rigid distal carpal row and the radius and ulna. The interosseous ligaments between the bones and the articular geometry allowed them to move in unison during wrist motion.
Linscheid and Weber observed carpal motion in the radial-ulnar plane and formulated different theories on the mechanics involved. Linscheid noted that as the wrist radially deviates, the scaphoid and proximal row flex. He thought that scaphoid flexion occurred in response to the pressure exerted by the trapezium and trapezoid. Weber postulated that it was the helicoid geometry of the triquetrohamate articulation that forced the distal row to translate palmarly during radial deviation. Volar translation of the distal row placed a flexion moment on the proximal row causing it to rotate into flexion.
Different theories on kinematics have offered a greater understanding of the coordinated motion between rows and the tendencies for motion when the system breaks down. Gifford and colleagues took note of the flexion and extension arcs of motion at the wrist and the relative contributions from the radiolunate and lunocapitate articulations. They thought that each row rotated around a single axis of rotation located around its proximal articular surface. They observed the instability of the arrangement and thought that the scaphoid was part of both rows and functioned to link the radius and the distal carpus, offering stability to an otherwise unstable system. Lichtman and colleagues initially introduced the oval ring concept; they thought that the circular arrangement of ligaments provided a continuum of stability and allowed for reciprocal motion between the proximal and distal rows. The rows are linked by the scaphotrapezial joint (mobile radial link) and the triquetrohamate joint (rotatory ulnar link).
No one concept adequately describes the complexity of carpal motion, and most agree that carpal kinetics can be explained by a combination of theories. It is evident that in the intact wrist, a balanced synchrony exists between the carpal bones and between the proximal row and distal row.
Ligament anatomy and mechanics
Intrinsic ligaments and extrinsic ligaments of the wrist link and stabilize the carpal bones ( Fig. 1 ). Intrinsic ligaments, otherwise known as interosseous ligaments, connect carpal bones to one another. Extrinsic ligaments connect the carpal bones to the radius and ulna and tend to be stronger than their intrinsic counterparts. There are 2 intrinsic interosseous ligaments in the proximal row (scapholunate and lunotriquetral) and 3 within the distal row (deep capitolunate, deep trapeziocapitate, trapeziotrapezoid). Extrinsic ligaments are classified as either volar or dorsal. Dorsal extrinsic ligaments include the dorsal radiocarpal and dorsal intercarpal ligaments. There are 4 volar radiocarpal ligaments (radial collateral, radioscaphocapitate, long radiolunate, and short radiolunate), 4 volar ulnocarpal ligaments (ulnar collateral, ulnotriquetral, ulnolunate, ulnocapitate), and one volar deltoid intercalated ligament. Volar extrinsic ligaments are thicker and stronger than the 2 dorsal extrinsic ligaments. As noted earlier, tendons do not directly insert on the carpals (except the pisiform) but help to stabilize their motion when actively contracting. Wrist motion tends to be initiated by the distal carpal row and metacarpals, which move as a unit. During wrist flexion, the distal row flexes and ulnar deviates. As the distal row flexes, the proximal row flexes. Conversely, with wrist extension, the distal row extends and radially deviates. As the distal row extends, the proximal row extends. Radial deviation of the wrist causes the distal row to incline radially, extend, and supinate, which leads the proximal row to flex and translate in an ulnar direction. During ulnar deviation, the distal row inclines ulnarly, flexes, and pronates causing the proximal row to extend and translate in the radial direction.
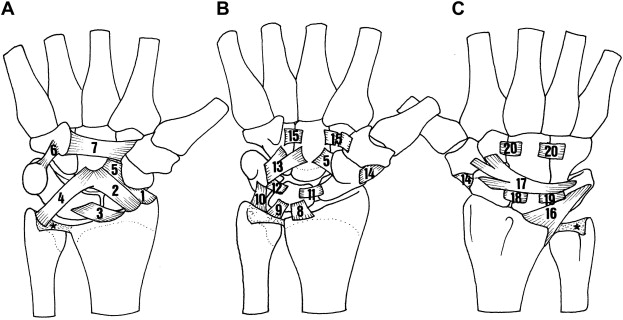
Carpal instability
Carpal instability is a broad term that is used to describe the carpal position that results following disruption to bone and/or wrist ligaments. Acute instability usually results from violent trauma, such as a fall or motor vehicle accident. Injury to an interosseous ligament can cause dissociation between carpal bones of a row. The most commonly injured structures are the scapholunate interosseous ligament between the scaphoid and lunate and the lunotriquetral interosseous ligament between the lunate and triquetrum. The thickest region of the scapholunate ligament is dorsal, whereas the lunotriquetral ligament is strongest volarly. Injury to one of these structures leads to altered carpal mechanics, called carpal instability dissociative . Disruption that alters motion between the proximal and distal carpal rows has been termed carpal instability nondissociative . A ligamentous disruption occurring within a row and between rows has been designated carpal instability complex .
Perilunate instability
Perilunate instability is a type of carpal instability complex. In this case, the carpal anatomy is disrupted in a predictable manner. Progressive ligament and bone dissociation depend on the degree of force imparted on the wrist. The defining feature of the perilunate injury is dissociation of the capitate from the lunate; 95% to 97% are dorsal perilunate dislocations (dorsal displacement of the capitate with respect to the longitudinal axis). Palmar dislocations are quite uncommon and in one series represented only 3% of perilunate injuries. Deformity can be subtle; spontaneous reduction has been reported, making missed injuries common.
Progressive perilunar instability was described by Mayfield. Cadaver wrists loaded in wrist extension, ulnar deviation, and intercarpal supination produced a characteristic sequence of carpus disruption around the lunate. Sequential disruption occurs in 4 stages ( Fig. 2 ). In stage 1, the scapholunate ligament is disrupted and the radioscapholunate ligament is torn. The force is then transmitted to the lunocapitate articulation where the capitate dislocates. Stage II is accompanied by injury to the radioscaphocapitate ligament, dorsal intercarpal ligament, and radial collateral ligament. In stage III, energy propagates into the lunotriquetral joint tearing the lunotriquetral ligament. A triquetral dislocation results; however, the lunate remains aligned with the radius. Finally, in stage IV, the lunate dislocates as a result of ulnotriquetral and dorsal radiocarpal tears. The lunate no longer remains within the lunate fossa and displaces volarly. The palmar ligaments, which usually remain intact, cause the lunate to rotate into the carpal tunnel.
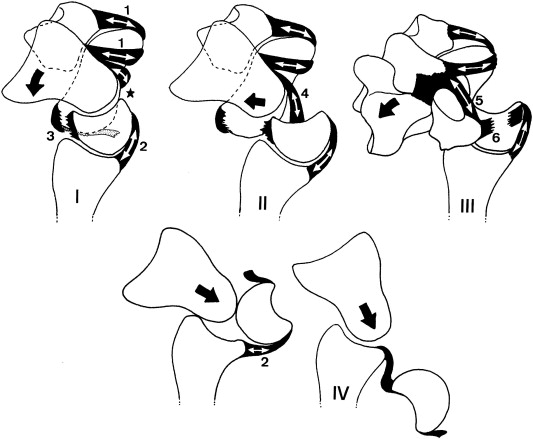
Herzberg further classified the final lunate position in 1993. Stage I described a perilunate dislocation where the lunate remains in its place under the radius. A lunate that was palmarly displaced from the lunate fossa (perilunate dislocation with lunate dislocation) was categorized as stage II. Stage II lunate dislocations were further organized by the degree of rotation involved. A lunate rotated 90° was termed stage IIa, whereas stage IIb identified a lunate that was 180° rotated.
Perilunate instability can occur through purely ligamentous means or in combination with fracture and are consequently subdivided into perilunate dislocations and perilunate fracture dislocations . Lesser arc injuries describe those that involve only ligamentous disruption. Greater arc injuries designate that a fracture of a bone or bones around the lunate has occurred. With greater arc injuries, the trans prefix is used to describe the fractured bones involved. Although Gilula originally described the normal arcs for radiographic interpretation in his classic article in 1979 ( Fig. 3 ), Johnson classified them into lesser arc and greater arc injuries. Recently, Bain and colleagues have modified Johnson’s classification to describe a third pattern of injury termed translunate arc , which occurs through the lunate ( Fig. 4 ).
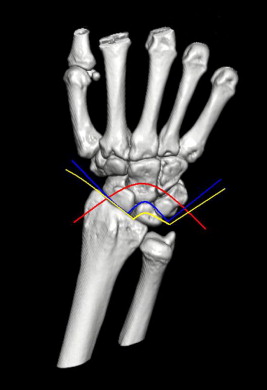
Common variants of perilunate injuries are trans-scaphoid or trans-styloid injuries. Uncommon variants include transtriquetral and transcapitate injuries. Scaphocapitate syndrome involves a particular dissipation of energy through the neck of the capitate. Other variants include scaphotrapezio-trapezoid dislocation that may replace the scapholunate dislocation or triquetrohamate dislocation that may replace the lunotriquetral dislocation within the perilunar instability model described by Mayfield.
A reverse mechanism has been described contrary to Mayfield’s radially initiated sequence of disruption. In a reverse Mayfield injury, reactive forces are directed toward the ulnar side of the wrist. Most often caused by a pronation-type injury, the pattern of sequential failure begins with the lunotriquetral ligament. The pathomechanics of ulnar-initiated perilunate instability has not been completely elucidated.
Diagnosis
Clinical and radiographic findings in perilunate injuries depend on the pattern of injury and multiple variations exist. Diagnosis requires a thorough history, complete physical examination, and appropriate imaging. Patients frequently describe a high-energy injury and present with a swollen, painful wrist. The carpus is most commonly dislocated dorsally, and physical examination reveals a prominent, palpable capitate. In acute injuries, soft tissue swelling may obscure bone landmarks. If the lunate is dislocated, it can encroach on the carpal tunnel and cause symptoms consistent with median nerve neuropathy. The incidence of acute carpal tunnel syndrome with perilunate injuries ranges from 16% to 46%. Standard orthogonal views should be scrutinized for incongruity of arcs, intercarpal distance abnormalities, and disruption of angular associations between carpal bones ( Fig. 5 ). Normal balance is assessed by the collinear alignment of the radius, lunate, capitate, and third metacarpal. Deviated values for the scapholunate angle (30–60°), radiolunate angle (<15°), and carpal height ratio (>0.5) provide objective evidence for injury. The posteroanterior (PA) view will demonstrate a loss of carpal arcs. The scaphoid and lunate will fall into a flexed position and a cortical ring sign directs the clinician to consider a scapholunate ligament and/or lunotriquetral injury. The lunate will seem triangular and it will be displaced relative to the extent of energy imparted. Loss of articular alignment, overlap of carpal bones, and fractures may also be present. The lateral image will demonstrate alteration of the collinear alignment of the radius, lunate, and capitate. The capitate is commonly displaced in a dorsal direction, and the lunate is displaced volarly. The lunate may be rotated 90° or 180°, resembling a spilled teacup. A CT scan can delineate subtle fracture lines that may be present.
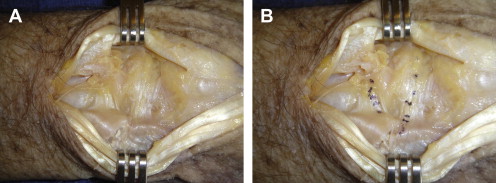
Treatment
The treatment of perilunate injuries has evolved over the years and includes closed reduction and immobilization, closed reduction and percutaneous fixation, external fixation combined with or without internal fixation, open reduction and fixation with Kirschner wires (k wires) or screws, lunate excision, proximal row carpectomy, and 4-corner fusion.
Initial closed reduction maneuvers will relieve pressure on the median nerve and prevent further cartilage damage ( Fig. 6 ). Traction for 10 to 15 minutes and analgesia may facilitate reduction. Closed reduction by itself is rarely a definitive treatment because the restoration of anatomy is critical to optimize the healing of ligaments and fractured bone. Adkison and Chapman observed that 59% of the perilunate injuries that were anatomically realigned with closed reduction lost their reduction over time. It is not surprising, then, that closed reduction techniques combined with percutaneous pinning are not always successful in maintaining carpal alignment.
