Fig. 8.1
A demineralized bone graft, Xpanse®, which expands when it is rehydrated. By expanding to create direct contact with the prepared host bone surface, it can assist osteogenic cells to travel into the graft
Beginning in the late 1990s a large number of other demineralized bone materials became available from tissue banking organizations, orthopaedic manufacturers, and alliances between the two. In an attempt to provide surgically-relevant handling characteristics using materials that were still outside of the prior patents, a host of carrier materials were introduced for the first time: carboxymethyl cellulose, hyaluronic acid, lipid-based carriers, reverse-phase polymers, among others –though all of these are not equivalent in their biological effects and may contribute to differences in performance. Around this time also, Osteotech developed and assessed various demineralized bone formulations that utilized fiber-based demineralized bone. It was shown that fibers offer distinct advantages in osteoconductivity for the formulation [30].
In the present time, there are a host of demineralized bone-based formulated grafting materials, each with its own group of claimed benefits, and each with significant differences in processing history, carrier materials, methods of achieving safety, and osteoinductive performance.
Demineralized Bone Safety
The safety profile for allograft bone is largely, but not completely, contributed by the process that it undergoes. Early development efforts on demineralized bone products [31–33] included viral, bacteriological and other safety considerations, and led to standardized techniques for demineralized bone processing and viral inactivation treatments. Tissues typically undergo processes that include cutting and shaping steps; milling; treatment with sterile water, alcohol, detergents and acid; freeze drying and packaging. The process is designed in such a way as to kill and remove bacteria, mold, fungi, virus and other potential adventitious agents, in accordance with government regulation and industry standards [34].
Demineralized bone comes from human cadaveric donors, who have made the choice, confirmed by a living family member, to donate tissue for the therapeutic use of an unrelated recipient. As with any human sourced-biologic product, a primary concern is the prevention of disease transmission from implanted tissues. This objective encompasses (1) Screening the donor population to exclude those with high risk; (2) Maintaining an aseptic processing environment that assures tissue sterility, as confirmed by bacteriologic testing; (3) Demonstrating process capabilities that inactivate virus, and (4) Prion considerations.
Screening and Initial Process
Initially, the donated human allograft bone tissue is screened [35] to exclude tissues that contain disease agents, and to exclude those donors that have lifestyle risks or a medical history that suggests risk of disease transmission. Donor selection (Fig. 8.2) starts with review of the medical history for the donor and an interview with a family member. Tissue is then procured aseptically, and stored in the frozen state. At the same time, the tissues are swabbed for bacterial contamination, and blood and tissue samples are obtained The samples go for detailed analysis and assessment for antibodies, antigens and NAT testing for HIV, HTLV, Hepatitis and Syphilis, in CLIA-certified testing laboratories, and using FDA-approved test kits. If an autopsy was performed, its results are obtained. Once the results become available, they are reviewed by the Medical Director (a board-certified physician with additional tissue banking credentials), and suitability is determined. There are many diseases and conditions which disqualify tissues from processing, including a host of diseases that could be transmitted by transplantation, evidence (e.g. recent tattoos) of an activity or lifestyle that increases risk, and conditions (e.g. Alzheimer’s disease) that are sometimes mistaken for diseases that are communicable. Careful screening can dramatically reduced the potential for disease transmission – for HIV the risk is reduced to less than 1 in 1.6 million by the screening process [35].


Fig. 8.2
Process for donor selection and suitability. At the first stage, a procurement decision is made, based upon the donor’s medical history and an interview with a family member. Once tissue is procured, additional testing is performed, including serology. Prior to processing, all of the medical history, interview, and test results are reviewed and suitability is determined
These tissues undergo initial processing steps to remove soft tissues, and also to mill the bone into fibers or to grind it into particles. These particles or fibers then go through a series of solvent, detergent, and antibiotic treatments to remove remaining cells, and to assure viral inactivation [32, 33]. Hydrochloric acid, the same acid used by osteoclasts during the resorption phase of remodeling, is used to remove the mineral while retaining the protein elements. The result is processed demineralized bone, in a particulate form (fibers or powder, Fig. 8.3).


Fig. 8.3
Preparation of bone prior to making the formulated demineralized bone graft. Bone can be ground into particles, or it can be milled to provide rolled shavings, or fibers – which are exclusive to the Grafton® demineralized bone forms. Once prepared into this form, the particles or fibers continue on to be demineralized by treatment with hydrochloric acid and then are combined with the excipient to make the formulated demineralized bone matrix product
The processed demineralized bone is then combined with an excipient or carrier material to make the formulated demineralized bone product. As part of the regulatory filings required by governmental regulatory agencies, such excipients must demonstrate biocompatibility, usually including tests to demonstrate that the formulated demineralized bone matrix or its carrier is not cytotoxic, a sensitizer, an irritant, genotoxic, a pyrogen, or a hemolytic agent. They are also typically tested by implantation for acute systemic toxicity and subacute toxicity. Commonly, these are demonstrated by the appropriate tests of the ISO10993 [36].
Aseptic Processing
Aseptic processing is a system that is designed to process tissue without contaminating it. It differs from terminally sterilized processes in that the intermediate steps must be much more exacting to maintain sterility through the process, and to avoid the need to apply irradiation or other damaging treatments in the terminal step. In aseptic processing, there are two primary sources of contamination to avoid: contamination from the processing room environment, and contamination of the tissues of one donor by the tissues of another donor. These imperatives are achieved by careful processing controls (such as a rigid requirement that only the tissues of a single donor be allowed in a processing area at any given time), and bacteriological testing at multiple stages of the process. Aseptically processed tissue is prepared in a cleanroom environment. Cleanrooms are classified according to the number of particles that they allow, with smaller number classifications indicating a cleaner environment [37]. Aseptically processed tissues are typically processed in a very tightly controlled cleanrooms (e.g. class 100), and then only released after their sterility has been proven by bacteriological testing. Relatively few companies have chosen this approach, since it is more costly and labor intensive than a process with a less well-controlled environment that ends in terminal sterilization. However aseptic processing has the substantial advantage of eliminating protein damage in the graft, which is produced by the terminal sterilization step. The water and air that will come into contact with the tissues are filtered and purified. Electrical systems have fail-safe conditions, including backup generators in case of power failure. Workers involved in processing the tissue must be clad in specialized cleanroom gowns and must periodically demonstrate their ability to gown and function in the processing area without contaminating tissues. Validated cleaning procedures are used to clean each processing area between batches. Each batch represents the tissue of only one donor, with full traceability through the process and back to the procurement stage. All of this infrastructure relies extensively upon repeated and vigilant bacteriological testing, as the key to maintaining a sterile environment and a product that passes sterility testing at the end of the process.
Viral Inactivation
There is evidence that even “minimal processing”, including marrow evacuation and lyophilization (which are always part of the process for demineralized bone), can cause a dramatic reduction to risks of viral transmission [38–40]. Demineralized bone undergoes these process steps and a number of others which each have the ability to kill virus. Taken together, they have the potential to achieve “viral inactivation”. To call demineralized bone “virally inactivated”, it is necessary to first estimate the maximum viral burden that could possibly be carried by the tissues, and then to calculate the capability of the process, established though validation testing, to kill or remove the virus. If the process capability provided by all process steps exceeds the maximal viral burden of the tissues by a sufficient magnitude (usually at least 3 logs, or 1000 times the theoretical maximum virus burden), then the process is a “viral inactivation process.”
The first demonstration by a commercial manufacturer showing full viral inactivation in demineralized bone was the D-Min® process used in Grafton®, summarized in a 1995 publication [32], and was preceded by a research study demonstrating the removal of a virus from tissue that were known to be infected with HIV [31]. For HIV, the commercial process was shown to be capable of reducing virus by more than 2.8 billion times [32], or more than 400,000 times the highest estimated viral concentration of a tissue, should it be infected before processing. The process was likewise shown to be capable of killing more virus than could exist in the tissue for other clinically relevant virus types in addition to HIV, including Hepatitis B, Hepatitis C, CMV, and Poliovirus, and demonstrated to be effective at viral removal in tissues from systemically infected animals [41]. Since this time other manufacturers of formulated demineralized bone products have also developed viral inactivation methods, which differ dramatically in the mechanisms of inactivation, as well as the amount of damage caused to the tissue proteins by the process.
Demineralized human allograft bone is safer than is screened whole blood for any of the viruses where risks have been evaluated in both tissues [32, 33, 42, 43]. Currently, acellular blood components such as pooled plasma protein fractions receive viral inactivation treatment, but whole, or cell-containing blood fractions do not [44]. By contrast, demineralized bone is screened, and additionally it is also virally inactivated by the process. Using HIV as an example, the combination of these effects argues that demineralized bone is about 2.2 billion times safer than the screened blood that surgeons use every day, without concern [32, 35, 42].
TSE Agents/Prions
Prions [45], the causative agent for transmissible spongiform encephalopathies including Creutzfeldt-Jakob disease and Bovine Spongiform Encephalopathy, are a special issue for tissue processing. They are difficult to kill, resisting steam sterilization and other traditional methods of sterilization. Since prions are proteins, many of the processing steps that could be used to inactivate prions would also have the undesirable consequence of damaging the collagen, BMP’s and other proteins that make demineralized bone function well in bone healing. However the potential for infectivity is not the same among all tissues of the body.
The infectious prion proteins (PrP) have been isolated in some (but not all) tissues, including muscle [46] and blood [47] in animal models of the infection. Different tissues have different levels of risk based upon the potential infectivity [48, 49]. According to the World Health Organization, neural tissues, pituitary and dura mater have the highest level of potential infectivity [48]. Blood is a tissue of intermediate infectivity which is categorized as a “Peripheral tissue{s} that have tested positive for infectivity and/or PrP in at least one form of TSE” [48]. By contrast, bone and tendon tissues are listed among those tissues with “no detected infectivity or PrP” by the World Health Organization.
Thus, the tissues being processed into demineralized bone have no known possibility of containing prions. Different processors take different approaches to establishing safety, and while no risk appears to be present, Osteotech has taken the additional steps of including screening steps that will exclude donors with any unexplained neurologic disorders, and will not collect tissues from sites near neural or other high infectivity tissues (e.g. vertebral bodies are not collected, due to their proximity to the spinal cord and nerve roots).
Composition
Many schoolchildren first become aware of demineralization by performing an experiment using a chicken bone, soaked for a time in a jar of vinegar, and then tying a knot in the bone after a sufficient portion of the inorganic mineral has been removed by the acid. The result is to transform a mineral reinforced structural composite material into a collagen-based connective tissue with exposed non-collagenous proteins. If one takes this child’s experiment, and modifies it by (1) Using human allograft tissue and hydrochloric acid, (2) separating the bone into sub-millimeter sized elements before treatment, (3) treating the tissue with detergents, antibiotics and alcohols to kill any potential pathogens and to remove cells, (4) processing, reacting and packaging in a cleanroom environment, and (5) encompassing the process with process validations, microbiological testing and a quality control system then the resulting processed demineralized bone matrix is the starting material for a formulated demineralized bone product.
Why Demineralize the Bone?
If demineralized bone is placed in a site without osteoblastic bone forming cells, but with access to the mesenchymal cells of surrounding tissues (as in a muscle bed) it will gradually transform into living mineralized cellular bone. This is ectopic bone formation. Why should demineralized bone produce such a dramatic response in tissues that do not have a direct mineralized tissue function? Early researchers compared the endochondral formation in ectopically implanted demineralized bone matrix to the events in embryological tissue formation [6], with the implication that the healing is tied in to remnant developmental pathways. This embryological context became the source of the concept of “induction” in association with demineralized bone matrix.
A more recent understanding comes from the osteoclastic events of bone remodeling that occur every day in the skeleton. The acid treatment used to prepare demineralized bone is actually a biomimetic process, since the osteoclasts that prepare the resorption site for new bone in remodeling also use hydrochloric acid to achieve demineralization of the interior surface of the resorption pit [50, 51], just as tissue processors do when they prepare demineralized bone. Thus, when allograft bone is demineralized in a processing facility, the entire surface of each bone element is treated with acid in the same manner as the interior surface of a resorption pit – a surface that the body recognizes as a location that requires a new layer of bone to refill. When demineralized bone is implanted, it provides many surfaces that are prepared, as osteoclasts would have prepared them, to receive osteogenic cells and to encourage bone formation.
The proteins that are responsible for the osteoinductive character of the demineralized bone are acid-stable, and can actually be damaged by neutral or basic conditions. In the early 1970s, Urist and his co-workers demonstrated that bone contains natural autolytic enzymes, which can degrade inductive proteins [25]. These autolytic enzymes have different activity, depending upon the temperature and the pH of the storage environment [25, 52, 53]. Urist showed that BMP’s are preserved at acidic [25] pH (pH < 5.5) and degraded by autolysis at neutral pH (pH ~ 7.4). This is critical not only at the time of processing, but also during the period that the product is being stored prior to surgical use. Thus a demineralized bone formulation that contains a carrier or storage media maintaining it at neutral or basic pH, can cause the degradation of the proteins through autolysis [53], and thereby provide a demineralized bone that has lower inductive activity by the time that the package is opened in a surgical setting.
Basis of Healing
Acting Upon Cells to Influence Healing
Demineralized bone uses the natural growth factors and bone morphogenetic proteins (TGF-β, IGF, FGF, PDGF, and BMP’s, among others [18]) that exist within the bone matrix to signal nearby cells and to influence them to become bone –forming cells and to make new bone. Even though they are present in mineralized bone, it takes the act of demineralization to expose them, making signaling possible [3]. These cell-signaling molecules inside demineralized bone have been successfully used in a host of applications for repair or modification of skeletal tissues – in craniomaxillofacial, dental, spinal, trauma, joint arthroplasty, joint revision, and podiatry, among others. The reason for this widespread clinical use is the potential to positively influence the healing process through passive scaffolding (osteoconductive) and active signaling (osteoinductive) effects.
Osteoinduction: Signaling/Recruitment/Differentiation
As a biomaterial for bone healing, demineralized bone matrix is unusual, in that it is not only osteoconductive, it is also osteoinductive. The term “osteoinductive” is widely used and extensively misused in the clinical and basic science literature, Einhorn [54] has provided a lucid definition which is in line with the original intent of Urist:
The ability to form bone at an ectopic site, where no osteogenic cells were present at the time of implantation, is a hallmark of osteoinduction [2, 29, 55]. Even though cancellous iliac crest graft does promote bone formation in a bony site due to the osteogenic cells that it contains, it is not osteoinductive. It will resorb, rather than forming new bone when placed into an ectopic site [56, 57].
Osteoinduction is a process that supports the mitogenesis of undifferentiated perivascular mesenchymal cells, leading to the formation of osteoprogenitor cells with the capacity to form new bone. Unlike osteoconductive materials, osteoinductive substances lead to the formation of bone at extraskeletal sites…..
Similarly, pathological calcification in implanted tissue has been confused with osteoinduction [58–60]. Inert calcium phosphate materials [61, 62], have been advocated by some in the biomaterials community as “osteoinductive.” This is a misuse of the term, however. These inorganic materials do not cause cells to differentiate or to divide – there is no active signaling. The behavior of these materials is analogous to that of natural mineral in pathological calcium deposits in the aorta and aortic valves [63–65] – rather than delivering a signaling substance such as bone morphogenetic protein, these materials co-opt molecules from the surrounding environment, in amounts too small to offer a clinical benefit.
True osteoinduction is an active, protein-based signaling of cells, using the BMP’s and associated growth factors that Marshall Urist identified in demineralized bone. In a rat muscle site, the implant follows an endochondral ossification pathway [7], by initially forming cartilage and then later replacing this scaffold with bone and marrow [7]. When explanted after 4 weeks, the demineralized bone which was originally implanted into a muscle with no existing osteoprogenitor cells, has been transformed to a nodule of hard mineralized bone. Histologically (Fig. 8.4), it shows evidence of active new bone formation, osteoblasts, osteoclasts, embedded osteocytes, and marrow elements. If the activity of the demineralized bone matrix has been maintained by careful processing to avoid deteriorating the embedded proteins, then the nodule should be filled with newly formed bone and marrow over more than 75 % of the nodule’s volume [55].


Fig. 8.4
Image of new bone formation in a rodent muscle site induced by demineralized bone. OS: regions of new osteoid created by a line of osteoblasts (circles). DBM: remnant fragments of demineralized bone. Bar = 100 μm
Measurement of Osteoinductivity
Implantation studies and characterization of osteoinductivity are performed in living animals. Early work on osteoinductivity used a variety of animals and a variety of ectopic sites. Urist used the rectus abdominus, quadriceps or erector spinae of rabbits, rats, mice, and guinea pigs [2]. Others used subcutaneous sites for evaluation [66, 67]. However, since vascularity and availability of mesenchymal cells will vary with the species of animal and the location of the implant, it is to be expected that osteoinduction results will not be comparable among them. Some have advocated using an in vitro test based upon either measured BMP content of the graft [68], or alternatively, on expression of osteoinductive markers [69] such as alkaline phosphatase, rather than in vivo testing in an animal model. However such tests have proven inconsistent their relationship to in vivo assessments – alkaline phosphatase expression in cell culture is not a reliable quantitative measure of osteoinductivity [70]. Likewise, the ELISA technique typically used to measure BMP content of the tissue is unreliable, due to variations in the ability to extract the BMP’s and the fact that ELISA measures both active and inactive forms of BMP. This limitation was well-summarized by Bae et al [71]:
The major disadvantage of ELISA is that its detection is based on an antibody that binds to only a small region of the complete protein structure. Therefore, it is possible that the protein detected by ELISA is just an inactive fragmented portion of the complete molecule. Even if the protein is indeed the complete molecule and not just a fragment, the protein may still not have any biologic activity (i.e., a dead protein).
Clearly the proper interpretation of ELISA results is critical, and conversely the mis-interpretation – by equating all ELISA signal as functional inductive protein – is a major and common source of error. At this time, it is widely accepted that the only reliable quantitative measurement of osteoinductivity is a well characterized version of Urist’s in vivo models in a muscle site. Edwards et al. [55], working at Osteotech, characterized and validated the model using an athymic rat and a hindlimb intermuscular in an athymic (rnu/rnu) rat. In his earliest work, Urist used euthymic animals [2, 29], but later as he worked with human demineralized bone, he began to use athymic rats and mice [72, 73]. Since athymic animals have no thymus gland, they allow the implantation of tissue from another species (human), without a cross-species incompatibility response.
Taking this lead from Urist, Edwards and colleagues utilized athymic rats, and a semi-quantitative scoring system based upon the proportion of newly formed bone and marrow within an implant, to quantify the amount of osteoinductivity present in demineralized bone [55]. They used a site between the semimembranous and adductor brevis muscles of the hindlimb. The implants remained in the site for 28 days, and then were removed and prepared for histological evaluation. Using a 0–4 scoring system in a controlled rnu/rnu strain of rats, Edwards and coworkers demonstrated selectivity (response only to active demineralized bone matrix), reproducibility (consistency of result from the same lot of demineralized bone matrix), and sensitivity (correlated increasing response to implants of higher activity) of the model. Such a model can then be used to assess a variety of factors that are thought to influence demineralized bone matrix activity, including age of the donor, source of the bone, processing variables and excipient.
Factors Influencing Osteoinductivity
Cortical and cancellous bone have different inherent capacities for osteoinductivity. When measured quantitatively using this method, cancellous bone is less osteoinductive than cortical bone, which is why formulated demineralized bone products are made of either particulated or milled cortical diaphyseal bone, rather than cancellous bone. In 1970, Urist and co-workers [22] performed an experiment in rabbits to quantify the amount of new bone that is formed from implants of either rabbit cortical bone or rabbit cancellous bone. They found that the amount of new bone induced by demineralized diaphyseal cortices implanted in a muscle site was four times that of an equivalent cancellous implant from the metaphysis. Others have later confirmed this in dogs [74].
In formulated demineralized bone products, the excipient is added to impart handling characteristics to the final formulation which are useful in its targeted surgical applications. However, the many materials that have been combined with demineralized bone to make formulated products can also negatively influence the biological properties of the graft material [75] and contribute, along with the processing and sterilization method to substantial differences in performance among marketed demineralized bone products [58].
Likewise, sterilization procedures that can be used to effect sterility of the graft, including gamma irradiation [20, 76], ethylene oxide [76, 77], hydrogen peroxide treatment [78], steam sterilization [79, 80], gas-plasma sterilization [80] and some proprietary methods [81] used by various tissue banks can all damage the proteins responsible for osteoinductivity.
The American Association of Tissue Banks (AATB) established standards for moisture in demineralized bone products [34] of less than 6 % moisture content. Among other reasons for this, water can affect the storage stability of the demineralized bone [82] by damaging the inductive proteins present in the matrix. As a result, formulations that include water in the carrier can have a harmful effect on osteoinductivity, particularly as the product approaches its expiration date.
Process Effects on Osteoinductivity
As we have seen, the processing methods used in preparing demineralized bone directly define its safety characteristics. Less well understood, the processing history also defines the activity and inductive performance of the demineralized bone. Marshall Urist, and others after him, taught us that demineralized bone must be treated carefully during processing to assure osteoinductivity at the end. In processing demineralized bone, a host of factors can negatively impact biological activity [83], ranging from the form and temperature of tissue storage [25, 82, 84] to the pH, time and order of the demineralizing and antibacterial solutions [83] to the selection of a strategy for achieving sterility of the tissue [20, 76, 80, 83] to the choice of excipient added to offer handling characteristics of the graft [75, 85]. These can all dramatically affect the osteoinductive character of the demineralized bone [33]. Since the processing history cannot be identified by viewing the graft, the differences in product performance associated with processing history of one demineralized bone product versus another are often invisible to the surgeon.
Processing of allograft bone is a balance between providing process treatments that enhance the safety of the tissues, and conversely, minimizing the damage induced by these same treatments. This is because nearly all treatments so far devised in the name of enhanced safety carry some additional detrimental effects to the proteins and matrix that activate new bone formation. Gamma irradiation, often chosen as a sterilization method for metallic implants and medical supplies, will cause a decline in osteoinductive performance in demineralized bone [20, 76] as well as BMP’s purified from demineralized bone [86]. The effect is dose-dependent, with greater declines corresponding to greater radiation doses [20, 87]. As a result, the most highly osteoinductive demineralized bone formulations are aseptically processed, rather than being terminally sterilized [33, 87].
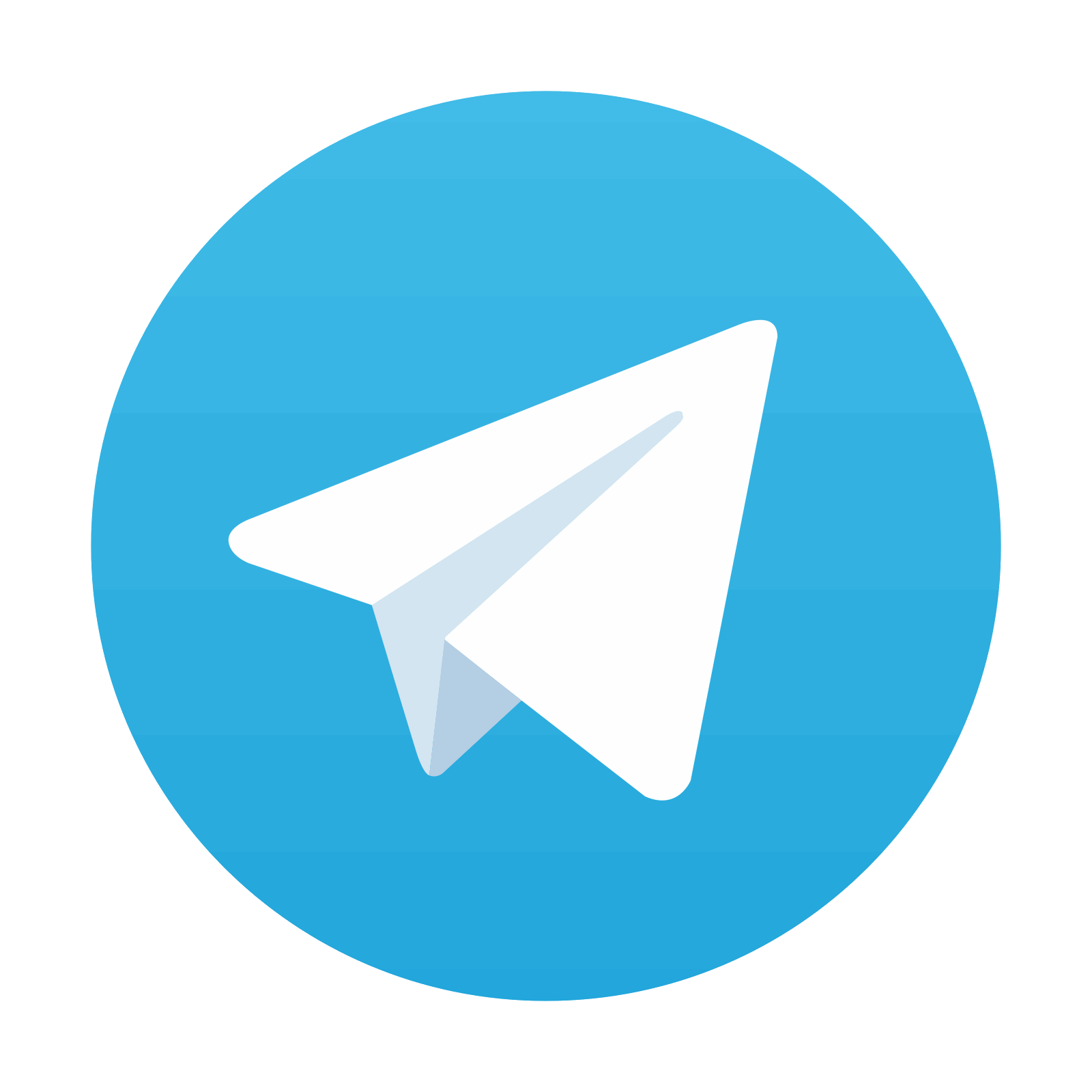
Stay updated, free articles. Join our Telegram channel

Full access? Get Clinical Tree
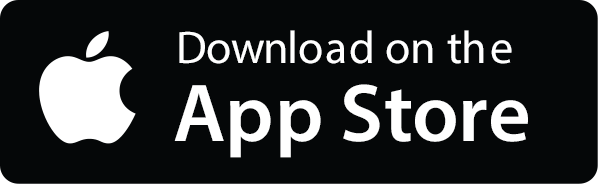
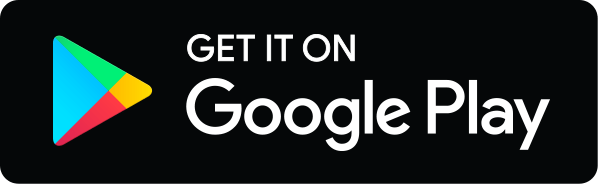