Children
Females
1–3 years
1.3
9–13 years
2.1
4–8 years
1.7
14–18 years
2.3
19–70+ years
2.7
Males
9–13 years
2.4
Pregnancy
3
14–18 years
3.3
Lactation
3.8
19–70+ years
3.7
Another 30 % of fluid intake comes from foods (e.g., fruits, vegetables). Fruits and vegetables can contain significant water (~90 %). In contrast, foods such as butter, oil, dried meats, chocolate, cookies, and cakes have comparatively low water content (<20 %). Peanut butter and shelled peanuts contain only traces of water, whereas walnuts contain 4 % water and dried coconuts and pecans around 7 %. This percentage increases for foods such as molasses (25 %), whole wheat bread (35 %), beef hamburger (54 %), and whole milk (87 %). The following foods contain at least 90 % water by weight: lettuce, raw strawberries, cucumbers, watercress, Swiss chard, boiled squash, green peppers, bean sprouts, boiled collards, watermelon, cantaloupe, canned pumpkin, celery, and raw peaches [4].
Metabolic water accounts for the remaining ~10 % of water that adds to fluid balance. In a sedentary person, metabolic water contributes approximately 14 % of the daily water requirement. The breakdown of the major macronutrients (carbohydrates, fats, protein) results in the formation of heat, carbon dioxide, and water. The breakdown of 100 g of carbohydrate releases 55 g of metabolic water, while water occurring from protein (41.3 g water/100 g protein) and fat (110 g water/100 g fat) is roughly equivalent [5]. In addition, each gram of glycogen is associated with ~2.7 g of water in storage which is liberated when glycogen is broken down during its catabolism for energy [6]. It should be noted, however, that metabolic water production alone has minimal affect at maintaining plasma volume levels during exercise [7].
The human body loses water via four mechanisms: through the skin (evaporation), in air leaving the lungs (expired air, water vapor), and in urine and feces. Under resting conditions, water lost in the urine constitutes the main route for water loss. Water loss through seepage from the skin during rest, called insensible perspiration, can account for ~250–350 mL of water per day. Additional water loss from the skin comes in the form of perspiration via sweat glands. Under normal environmental conditions, sweat losses can amount to between 500 and 700 mL/day, depending on body size. However, sweat losses can greatly exceed resting levels during exercise, especially under significant thermal stress (high ambient temperature and humidity). For example, someone exercising in a hot environment can produce up to 12 L of sweat (equivalent of 12 kg) at a rate of 1 L/h during prolonged exercise. These levels of sweat losses can exceed 3 L/h in well-acclimated athletes [2, 8]. Insensible water losses also occur when relatively dry air is brought into the lungs and moistened before reaching the alveoli. Typical water losses in the expired air amount to 250–350 mL daily but can be much higher in physically active individuals [1]. For physically active persons, the respiratory passages release 2–5 mL of water each minute during vigorous exercise, depending on climatic conditions of the environment. Ventilatory water loss is less in hot, humid climates than in cold temperatures (cold air contains little moisture) and at high altitudes. At high altitude, water losses, from insensible sources, are greater because water evaporates more quickly under lower air pressures and ventilation rates are higher resulting in more air requiring humidification as it enters the lungs [9].
The volume of urine excreted daily by the kidneys ranges from 1,000 to 1,500 mL, or about 1.5 quarts. This water loss is termed obligatory water losses because it is necessary to rid the body of metabolic by-products like urea, the end product of protein metabolism. Total daily urine output can be upregulated to match fluid intake in order to prevent excessive water accumulation in the body and the associated electrolyte disturbances. Urine output may also increase depending on the macronutrients (carbohydrate, fats, protein) in the diet. For example, eating large amounts of protein can increase obligatory water losses as each gram of solute excreted by the kidneys requires 15 mL of water [1]. Intestinal water loss in the form of feces amounts to 100–200 mL/day. However, water losses can be much higher (1,500–5,000 mL/day) in cases where individuals have intestinal disturbances and/or vomiting. In addition to losing large quantities of water, electrolytes are lost resulting in fluid and electrolyte imbalances that can be deadly if not corrected [1].
In healthy individuals, water lost through excessive sweating during exercise can quickly result in significant dehydration (≥2 % body weight loss) [10]. The intensity of the physical activity, environmental temperature, and relative humidity contribute to the amount of water lost through sweating. The mechanisms for sweat loss during exercise are covered in the next section. Overall, the equation to determine daily fluid balance can be found below where fluid balance is in L/day, body weight is in kg weighed approximately 24 h apart after voiding (urination and defecation), and insensible water loss is the sum of evaporative water loss from the lungs and skin:


6.4 Exercise and Hydration Requirements
In order to discuss hydration requirements with exercise, heat balance must first be outlined. Heat balance or heat storage (HS) is the sum of heat gain and loss (see equation below). Heat balance during exercise occurs through four main processes: evaporation (sweat and respiratory; E), conduction (contact with a solid surface; Cd), convection (contact with a fluid; Cv), and radiation (electromagnetic waves; R). Exercise increases metabolic heat production (M):


While all four mechanisms are important at rest, evaporation is the primary mechanism for heat loss during exercise. Not accounting for changes in the environment, heat loss through evaporation must make up for the increase in metabolic heat production. This is apparent in the equation below as an increase in metabolic heat production increases heat storage (+) and evaporation reduces heat storage (−). The increase in metabolic heat production during exercise occurs because the body is ~25 % efficient at converting stored fuels (fat and carbohydrates) into usable energy for muscle contraction and movement. The remaining ~75 % of the energy released in metabolism accumulates in the body as heat and raises the body’s core temperature. Consequently, higher metabolic demands on the body, or an increase in exercise intensity, will result in greater body heat accumulation and accelerated core temperature. In order to stabilize the rise in body heat, or core temperature, sweat volume increases with an increase in exercise intensity, or metabolic heat production.
Environmental factors affect all the mechanisms responsible for heat storage shown in the above equation. A hot environment can increase the stored body heat by increasing, or reducing the cooling effect of, conduction, convection, and radiation. However, a hot environment can increase the amount of sweat produced and, therefore, the potential for heat loss through evaporation. Relative humidity, or the amount of water in the air relative to the total amount the air can hold, impacts the effectiveness of sweat to maintain core body temperature by directly affecting the rate of evaporation of sweat from the skin. Under extreme environmental conditions, high ambient temperature, and relative humidity, sweat beads on the skin and rolls off before it has the chance to evaporate. In dry or less humid environments, ambient air can hold substantially more moisture, and fluid evaporates from the skin into the surrounding air and, with it, carries substantial heat from the remaining water on the skin. The overall effect is cooler water on the skin and overall heat loss from the body.
Dehydration results from loss of significant amounts of body water during exercise that exceeds 1-h duration depending on the intensity and type of exercise and resultant sweat rate. Larger amounts of water are lost as the result of exercise at greater intensity especially when coupled with hot and humid environments as shown previously. However, even when exercise is conducted in milder environmental conditions, sweat is still produced. Sweat rate is also influenced by the level of acclimation to a hot/humid environment. Acclimatized individuals can achieve sweat rates of around 3 L/h during a bout of intense exercise and may reach a total volume of 12 L in a single day.
The level of dehydration will determine the level of physiologic and performance changes that occur. Dehydration approaching 2 % negatively affects body temperature regulation and cardiovascular functioning. For example, in one study, body fluid deficits of only 1 % body weight increased the core temperature when compared with that seen during equivalent exercise and a normal hydrated state; and for each liter of fluid lost, the heart rate increased approximately 8 beats per minute and there was a 1.0 L/min decline in cardiac output [11]. As dehydration worsens, the plasma volume and peripheral blood flow decline, and thus, the sweat rate declines in the exercising individual, further complicating the body’s ability to maintain a safe core temperature. Specifically, dehydration prior to exercise or competition equal to approximately 5 % of body weight can result in an increase in heart rate and core temperature and a reduction in the sweating rate, VO2max, and exercise capacity compared to a normal euhydrated state [12]. In a dehydrated state, this is explained by the reduction in blood volume that results in less ventricular filling pressure, which ultimately causes an increased heart rate and an approximately 25–30 % reduction in stroke volume. The consequences are reduced cardiac output and arterial blood pressure, leading to reduced aerobic performance [13]. In one study, physical work capacity and physiological function were shown to decline with fluid loss of around 4–5 % body weight [14]. Dehydration equal to 4.3 % of the body mass reduced walking endurance by 48 % along with a 22 % decrease in VO2max in this study [14]. The rise in core body temperature is directly related to reduced peripheral blood flow and sweat rate. This becomes most pronounced in hot humid environments in which ambient air is, for the most part, completely saturated.
Many sports and athletic events have the potential to cause large fluid deficits if adequate fluid intake is not encouraged. Triathletes and other elite endurance athletes can lose approximately ≥5 L of fluid (~6–10 % of body mass) during competition. For athletes of lower caliber, fluid loss does not usually exceed 500 mL/h, whereas even in temperate climates of 10 °C (50 °F), soccer players can lose an average of 2 L during a 90-min game [15]. Substantial water loss occurs outside typical exercise-related events too. For example, athletes concerned with “making weight” (e.g., boxers, wrestlers, weightlifters) seek out rapid weight loss methods such as saunas, steam rooms, or severe dietary alterations including restricting fluids, diuretics, and purging. These techniques accelerate weight loss but also increase an athlete’s susceptibility to heat-related illness (e.g., muscle cramps, heat exhaustion, heat stroke) [16, 17].
6.5 Fluid Balance and Exercise
As mentioned in Sect. 6.3, fluid balance is the sum of fluid intake and fluid loss. In addition, we discussed the effect of water losses, particularly through evaporation, during exercise and the requirements for fluid intake according to environmental conditions and the types of activities in Sect. 6.4. Much research has been conducted on optimal hydration strategies to improve fluid balance during exercise. While additional research is needed to optimize and individualize these strategies, solid recommendations for the pre-exercise, during exercise, and post-exercise time frames have been made. In each section, the recommendations from the American College of Sports Medicine position stand on fluid replacement will be outlined first, followed by supporting evidence. Note that many of the strategies attempt to optimize not only fluid balance but also sodium balance recognizing that sodium, the primary extracellular and intravascular cation, has important implications in reducing water lost in urine.
6.5.1 Before Exercise
The American College of Sports Medicine recommends that individuals try to achieve at least a euhydrated, adequately hydrated state before starting exercise. Depending on previous exercise, environmental exposure, and age, among other modifying factors, more aggressive approaches than recommended may be necessary to achieve a euhydrated state. Hydration strategies should start at least 4 h before exercise and consist of ingesting 5–7 mL of fluid per kg body weight. Additional fluids should be ingested about 2 h before exercise if the urine color is still dark. In order to reduce the amount of ingested fluids before exercise, foods or snacks containing sodium are recommended. The evidence to support these recommendations is strong, in particular, core temperature and cardiovascular function (heart rate and cardiac output) are better maintained during exercise when the individual is properly hydrated at the onset. At the time the position stand was written, research into sodium-containing beverages before exercise was scarce. However, recent research in which participants ingested 350 mL of chicken noodle soup as a pre-exercise beverage showed an increase in ad libitum water intake during long-duration exercise and a potential alteration in kidney function to reduce urine output during exercise in both temperate and hot/dry environments [18, 19]. Other methods of pre-exercise hyperhydration aimed at expanding intravascular volumes, including glycerol (discussed in Sect. 6.8), have not been very successful.
6.5.2 During Exercise
Prior to exercise, additional fluid intake (hyperhydration) can provide some initial prevention against dehydration and other heat-related problems affecting performance. This is especially true during exercise in which there is no fluid intake. The pre-exercise ritual helps facilitate increases in sweating during exercise with a much less pronounced increase in body temperature. A sensible method for increasing pre-exercise hydration is to ingest around 500 mL of water the night before competition, another 500 mL first thing in the morning, and another 400–600 mL (13–20 oz.) of cool water approximately 20 min before exercise [8, 20]. In a study involving elite soccer players, a regimented intake of fluid of roughly 4.5 L/day a week (even though greater urine output) before competition helped facilitate better temperature regulation during a warm weather competition creating a 1.1 L greater total body fluid volume when compared to the athletes’ typical daily intake of fluid [21].
Pre-exercise water/fluid intake benefits:
1.
Delays dehydration
2.
Increases sweating during exercise
3.
Diminishes the rise in core temperature
The goal for fluid intake during exercise is to prevent fluid balance changes greater than 2 % body weight. As mentioned earlier, this level of dehydration is associated with reduced physical and cognitive performance as well as altered cardiovascular function and heat tolerance. Many factors play into the absolute amounts of water lost during exercise including environmental conditions, age, health status, heat acclimatization, and genetics among others. In planning the amounts of fluid replacement required during exercise, typical body weight losses, as determined by the difference between pre- and post-body weight accounting for fluid intake, can be used to estimate sweat rates and, therefore, fluid needs. For example, an 80-kg man who exercises for 1 h and weighs 79.5 kg after exercise and drinks 1 L of fluid has an approximate sweat rate, and fluid requirement, of 1.5 L/h to maintain euhydration assuming 1 L of fluid is equal to 1 kg. Thirst is not a good indicator of fluid losses during exercise, and in the absence of forced fluid intake, individuals will progressively become more dehydrated, termed voluntary dehydration. Ad libitum fluid intake during exercise, however, can be influenced by beverage temperature, flavor, and composition with cool, flavored beverages containing carbohydrates and sodium resulting in greater amounts of fluid ingested. Last, the likelihood of making an error in the amount of fluid needed to maintain euhydration is greater in events lasting several hours. In longer bouts of exercise, minor miscalculations in sweat rates can lead to significant dehydration or dilutional hyponatremia, a serious condition whereby plasma sodium is diluted by the ingested beverage [22].
6.5.3 After Exercise
As previously noted, pre-exercise fluid ingestion does not negate the responsibility of fluid replacement during the bout of exercise itself. If an athlete does not ingest fluids during an event such as a marathon, the benefits of hyperhydration before competition are soon negated, especially in a hot, humid climate, because it is not possible to match fluid loss (~2,000 mL/h) with fluid intake due to the slower gastric emptying rate (~1,000 mL/h) of the stomach. Thus, pre-exercise hyperhydration becomes imperative prior to competition, especially long-endurance events.
The main goal after exercise is to completely replace fluid and electrolytes lost during exercise. Under normal time constraints to achieve euhydration, normal meals and fluid intake is adequate. However, if a shorter time period exists until the next exercise bout, larger volumes of water and electrolyte (sodium) intakes will be necessary. The additional sodium intakes can be achieved through sports beverages and salty snacks or by adding extra salt to your food. Depending on how short the duration between exercise bouts, individuals will need to ingest more than the level of dehydration at the end of the exercise (i.e., 150 % of the dehydration level) to account for future fluid losses in urine. Less fluid will be needed if greater amounts of sodium in the beverages or foods are ingested. In addition to reducing urinary water losses, the additional sodium intake will encourage fluid intake further improving the likelihood of achieving euhydration before the next exercise bout begins.
Perception of thirst is not a good indicator of overall fluid balance. Additional methods must be used to ensure adequate hydration. As noted earlier, body weight prior to and immediately after exercise can help assess water or fluid loss resulting from physical activity and/or exercise. Monitoring urine output is another method by which the hydration level can be visually monitored, as a dark amber/yellow output with subsequent odor is a sign of dehydration whereas a normal euhydrated individual excretes lighter, clearer urine with little or no odor. Coaches and athletic trainers should encourage periodic water breaks during activity to prevent severe dehydration and subsequent loss of performance.
6.6 Electrolyte Replacement
It is generally accepted that a small increase in the amount of salt added to the food in the diet is adequate to replace any sodium lost through sweat. However, sodium balance can be disturbed by increased loses of large amounts of sweat or urine or ingesting large quantities of plain water in conjunction with a diet low in sodium. However, electrolytes and glucose found in sports drinks may hasten rehydration either through reducing urine losses or increasing fluid intake or both [23, 24]. Restoration of water and sodium balance in recovery occurs more rapidly by adding moderate to high amounts of sodium (20–60 mmol/L) to the rehydration drink or combining solid food with appropriate sodium content. Adding a small amount of potassium (2–5 mmol/L) may enhance water retention in the intracellular space and replace lost potassium during exercise [8, 20]. The American College of Sports Medicine (ACSM) also recommends that sports drinks contain 0.5–0.7 g of sodium per liter of fluid consumed during exercise lasting more than 1 h [20].
Restoring fluid balance in the body requires ingesting a fluid volume that exceeds the volume of fluid or water lost through sweat by approximately 25–50 % [25]. When a fluid is ingested, especially dilute beverages like water, the changes in blood plasma and gut distension cause the body to increase urine production and rapidly decrease the perception of thirst. Therefore, keeping a consistently higher plasma sodium concentration by adding sodium to ingested fluid may sustain the thirst mechanism and promote retention of ingested fluids. Typical activity including exercise generally results in only minimal loss of potassium (partially due to the potassium-conserving mechanism in the kidneys), and at higher-intensity exercise levels, losses appear to have no consequences [26]. Even under extreme conditions, potassium needs can be met by ingesting foods that contain this mineral (e.g., bananas and potassium-rich citrus fruits).
6.7 Hyponatremia
Though hydration is important to exercise, excessive fluid consumption during longer events (i.e., marathons and longer triathlons) under certain conditions can result in the serious medical illness hyponatremia, sometimes referred to as “water intoxication” [22]. Hyponatremia occurs when low plasma sodium concentrations are created in the blood due to the over-ingestion of water. In essence, the blood becomes “dilute” of sodium, thus creating an imbalance in osmotic pressure across the blood–brain barrier that initiates rapid water influx to the brain resulting in edema of brain tissue. In addition, the following symptoms, from mild to severe, can also be caused by this condition: confusion, headache, malaise, nausea, cramping, seizures, coma, pulmonary edema, cardiac arrest, and death [22, 27, 28].
During exercise in hot, humid weather, the body produces a sweat rate of more than 1 L an hour, with a sweat sodium concentration ranging between 20 and 100 mEq L−1 [8]. In addition, repeatedly ingesting large volumes of plain water draws sodium from the extracellular fluid compartment into the unabsorbed intestinal water, further diluting serum sodium concentration [29, 30]. Exercise compounds the problem because urine production decreases during exercise due to a significantly reduced renal blood flow. This reduces the body’s ability to excrete excess water [8, 20]. Although hyponatremia is usually associated with competitive athletes, recreational participants and occupational workers should be aware of the dangers of excessive hydration and should ensure that fluid intake does not exceed fluid loss [8, 20]. It has been recommended that the following steps be taken to prevent development of hyponatremia when planning on competing in prolonged endurance activities [8, 20]:
1.
Drink 400–600 mL (14–22 oz.) of fluid 2–3 h before exercise.
2.
Drink 150–300 mL (5–10 oz.) of fluid about 30 min before exercise.
3.
Drink no more than 1,000 mL/h (32 oz.) of plain water spread over 15-min intervals during or after exercise.
4.
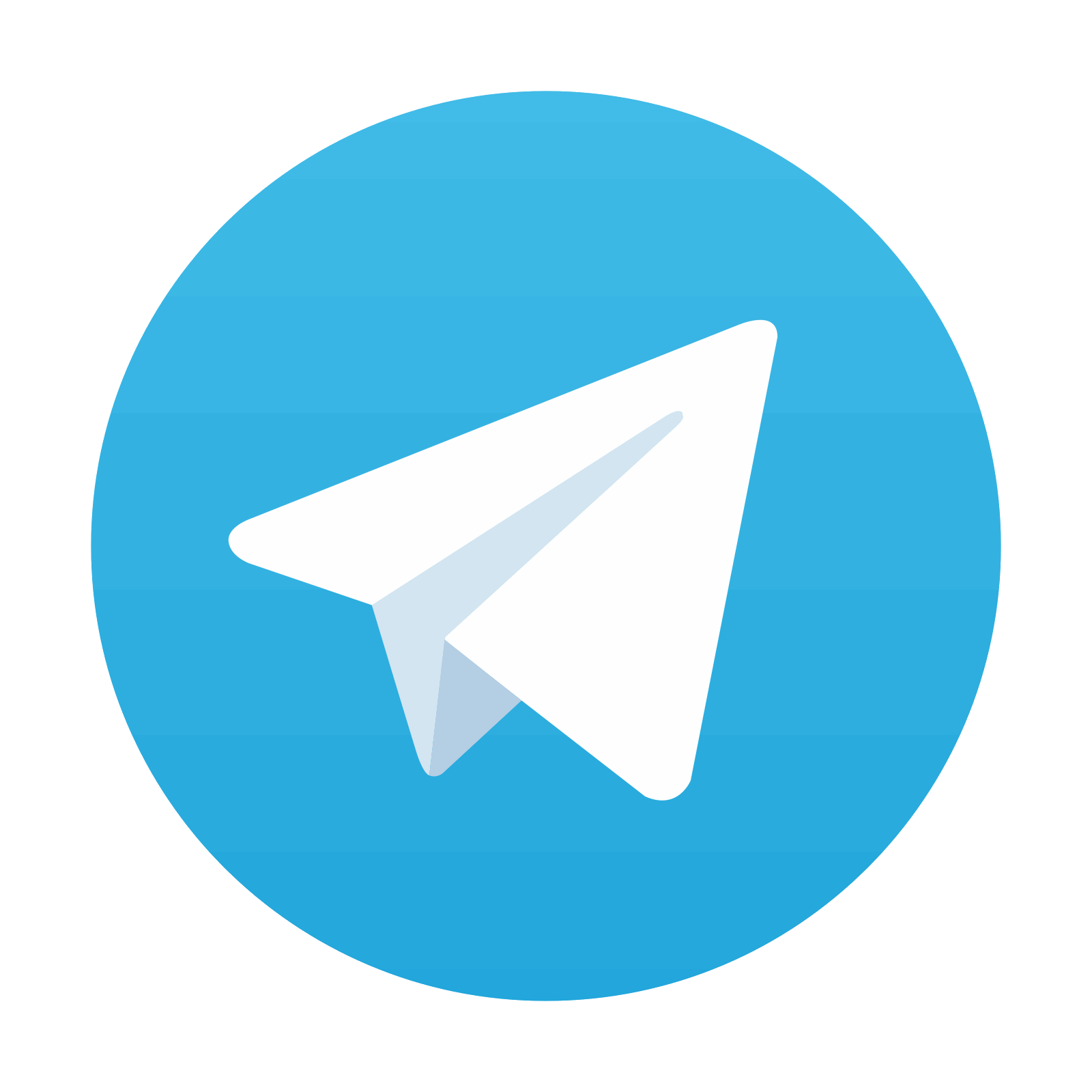
Add a small amount of sodium (~ ¼–½ tsp. per 32 oz.) to the ingested fluid. Water, carbohydrate fuel, and electrolytes from manufactured sports drinks can also be helpful.
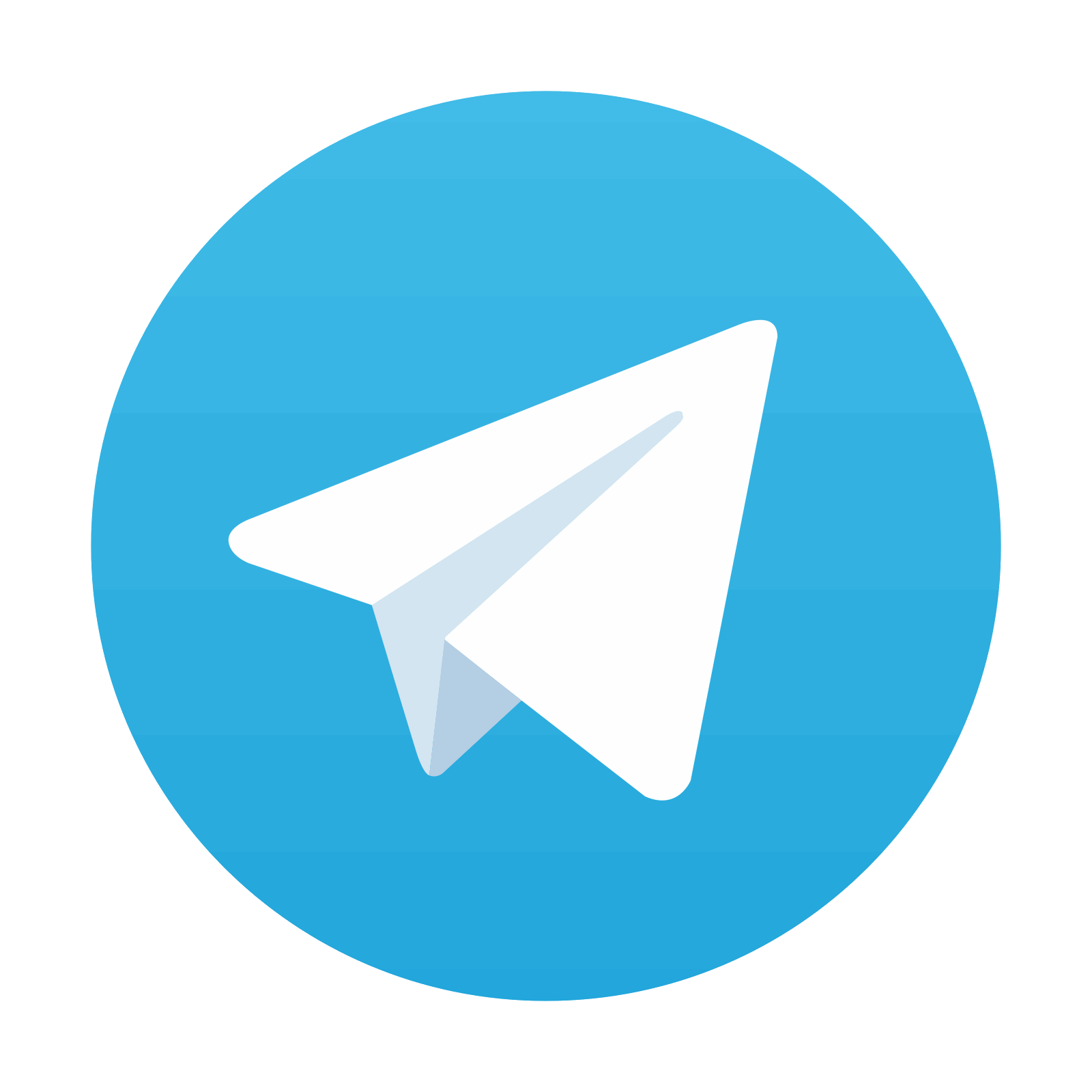
Stay updated, free articles. Join our Telegram channel

Full access? Get Clinical Tree
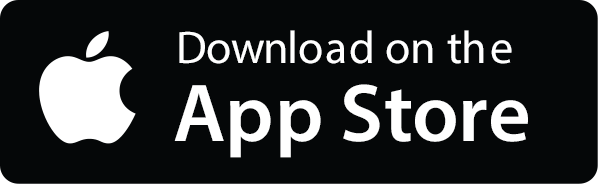
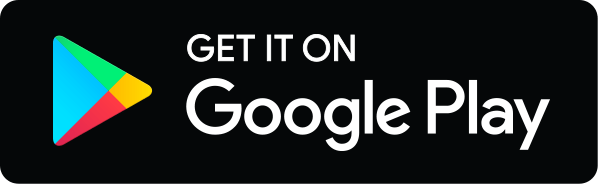
