7.24 Fascial fitness Whenever a football player is not able to take the field because of a recurrent calf spasm, a tennis star gives up early on a match due to knee problems, or a sprinter limps across the finish line with a torn Achilles tendon, the problem is most often neither in the musculature nor the skeleton. Instead, it is the structure of the connective tissue – ligaments, tendons, joint capsules, etc. – which has been loaded beyond its capacity (Renström & Johnson 1985; Hyman & Rodeo 2000; Mackey et al. 2008; Counsel & Breidahl 2010). A focused training of the fascial network could be of great importance for athletes, dancers and other movement advocates. If one’s fascial body is well trained, that is to say optimally elastic and resilient, then it can be relied on to perform effectively and at the same time to offer a high degree of injury prevention (Kjaer et al. 2009). Until now, most of the emphasis in sports has been focused on the classic triad of muscular strength, cardiovascular conditioning, and neuromuscular coordination (Jenkins 2005). Some alternative physical training activities – such as Pilates, yoga, Continuum Movement, and martial art – are already taking the connective tissue network into account. Here the importance of the fasciae is often specifically discussed, though modern insights in the field of fascia research have often not been specifically included. We suggest that in order to build up an injury-resistant and elastic fascial body network it is essential to translate current insights in the field of fascia research into a practical training program. Our intention is to encourage physical therapists, sports trainers and movement enthusiasts to incorporate the principles presented in this chapter, and to apply them to their specific context. The varied capacities of fibrous collagenous connective tissues make it possible for these materials to continuously adapt to the regularly occurring strain, particularly in relation to changes in length, strength and ability to shear. Not only the density of bone changes, for example, as happens with astronauts who spend time in zero gravity wherein the bones become more porous (Ingber 2008); fascial tissues also react to their dominant loading patterns. With the help of the fibroblasts, they react to everyday strain as well as to specific training, steadily remodeling the arrangement of their collagenous fiber network (Kjaer et al. 2009). For example, with each passing year half the collagen fibrils are replaced in a healthy body (Neuberger & Slack 1953). The intention of fascial fitness is to influence this replacement via specific training activities which will, after 6 to 24 months, result in a ‘silk-like bodysuit’ which is not only strong but also allows for a smoothly gliding joint mobility over wide angular ranges. Interestingly, the fascial tissues of young people show stronger undulations within their collagen fibers, reminiscent of elastic springs, whereas in older people the collagen fibers appear as rather flattened (Staubesand et al. 1997). Research has confirmed the previously optimistic assumption that proper exercise loading – if applied regularly – can induce a more youthful collagen architecture, which shows a more wavy fiber arrangement (Wood et al. 1988; Jarniven et al. 2002) and which also expresses a significant increased elastic storage capacity (Fig. 7.24.1) (Reeves et al. 2006). However, it seems to matter which kind of exercise movements are applied: a controlled exercise study using slow-velocity and low-load contractions only demonstrated an increase in muscular strength and volume; however, it failed to yield any change in the elastic storage capacity of the collagenous structures (Kubo et al. 2003). Fig. 7.24.1 • Increased elastic storage capacity. Kangaroos can jump much farther than can be explained by the force of the contraction of their leg muscles. Under closer scrutiny, scientists discovered that a spring-like action is behind the unique ability: the so-called ‘catapult mechanism’ (Kram & Dawson 1998). Here, the tendons and the fascia of the legs are tensioned like elastic bands. The release of this stored energy is what makes the amazing jumps possible. The discovery soon thereafter that the same mechanism is also utilized by gazelles was hardly surprising. These animals are also capable of impressive leaping as well as running, though their musculature is not especially powerful. On the contrary, gazelles are generally considered to be rather delicate, making the springy ease of their incredible jumps all the more interesting. The possibility of high-resolution ultrasound examination made it possible to discover similar orchestration of loading between muscle and fascia in human movement. Surprisingly, it has been found that the fasciae of humans have a similar kinetic storage capacity to that of kangaroos and gazelles (Sawicki et al. 2009). This is not only made use of when we jump or run but also with simple walking, as a significant part of the energy of the movement comes from the same springiness described above. This new discovery has led to an active revision of long-accepted principles in the field of movement science. In the past, it was assumed that in a muscular joint movement, the skeletal muscles involved shorten and this energy passes through passive tendons, which results in the movement of the joint. This classic form of energy transfer is still true – according to these recent measurements – for steady movements such as bicycling. Here, the muscle fibers actively change in length, while the tendons and aponeuroses scarcely grow longer. The fascial elements remain quite passive. This is in contrast to oscillatory movements with an elastic spring quality, in which the length of the muscle fibers changes little. Here, the muscle fibers contract in an almost isometric fashion (they stiffen temporarily without any significant change of their length) while the fascial elements function in an elastic way with a movement similar to that of a yo-yo (Fig. 7.24.2). It is this lengthening and shortening of the fascial elements that ‘produces’ the actual movement (Fukunaga et al. 2002; Kawakami et al. 2002). Fig. 7.24.2 • Length changes of fascial elements and muscle fibres in an oscillatory movement with elastic recoil properties (A) and in conventional muscle training (B). The elastic tendinous (or fascial) elements are shown as springs, the myofibers as straight lines above. Note that during a conventional movement (B) the fascial elements do not change their length significantly while the muscle fibers clearly change their length. During movements like hopping or jumping, however, the muscle fibers contract almost isometrically while the fascial elements lengthen and shorten like an elastic yoyo spring. In Kawakami Y, Muraoka T, Ito S, Kanehisa H, Fukunaga T 2002 In vivo muscle fibre behaviour during countermovement exercise in humans reveals a significant role for tendon elasticity. From Kawakami et al. 2002 J Physiol 540 (2): 635–646. It is of interest that the elastic movement quality in young people is associated with a typical two-directional lattice arrangement of their fasciae, similar to a woman’s stocking (Staubesand et al. 1997). In contrast, as we age and usually lose the springiness in our gait, the fascial architecture takes on a more haphazard and multidirectional arrangement. Animal experiments have also shown that lack of movement quickly fosters the development of additional cross-links in fascial tissues. The fibers lose their elasticity and do not glide against one another as they once did; instead, they become stuck together and form tissue adhesions, and in the worst cases they actually become matted together (Fig. 7.24.3) (Jarvinen et al. 2002). The goal of the fascial fitness training is to stimulate fascial fibroblasts to lay down a more youthful and ‘gazelle-like’ fiber architecture. This is done through movements that load the fascial tissues over multiple extension ranges while utilizing their elastic springiness. Fig. 7.24.3 • Collagen architecture responds to loading. Figure 7.24.4 illustrates different fascial elements affected by various loading regimens. Classic weight training loads the muscle in its normal range of motion, thereby strengthening the fascial tissues, which are arranged in series with the active muscle fibers. In addition, the transverse fibers across the muscular envelope are stimulated as well. However, little effect can be expected on extramuscular fasciae as well as on those intramuscular fascial fibers that are arranged in parallel to the active muscle fibers (Huijing 1999). Fig. 7.24.4 • Loading of different fascial components. Classic Hatha yoga stretches, on the other hand, will show little effect on those fascial tissues which are arranged in series with the muscle fibers, since the relaxed myofibers are much softer than their serially arranged tendinous extensions and will therefore ‘swallow’ most of the elongation (Jami 1992). However, such stretching provides good stimulation for fascial tissues which are hardly reached by classic muscle training, such as the extramuscular fasciae and the intramuscular fasciae oriented in parallel to the myofibers. Finally, a dynamic muscular loading pattern in which the muscle is both activated and extended promises a more comprehensive stimulation of fascial tissues. This can be achieved by muscular activation (e.g. against resistance) in a lengthened position while requiring small or medium amounts of muscle force only. Soft elastic bounces in the end ranges of available motion can also be utilized for that purpose. The following guidelines are developed to make such training more efficient.
Suggestions for a fascia-oriented training approach in sports and movement therapies
Introduction
Fascial remodeling
Regular oscillatory exercise, such as daily rapid running, induces a higher storage capacity in the tendinous tissues of rats, compared with their nonrunning peers. This is expressed in a more spring-like recoil movement, as shown on the left. The area between the respective loading versus unloading curves represents the amount of ’hysteresis’: the smaller hysteresis of the trained animals (grey) reveals their more ’elastic’ tissue storage capacity, whereas the larger hysteresis of their peers signifies their more ’viscoelastic’ tissue properties, also called inertia. Illustration modified after Reeves 2006. Reeves ND, Narici MV, Maganaris CN (2006) Myotendinous plasticity to ageing and resistance exercise in humans. Exp Physiol 91(3): 483–498.
The catapult mechanism: elastic recoil of fascial tissues
Fasciae of young people – shown on the left – express more often a clear two-directional (lattice) orientation of their collagen fiber network. In addition, the individual collagen fibers show a stronger crimp formation. In contrast, fasciae from older persons show a more irregular alignment with less crimp formation. As evidenced by animal studies, application of proper exercise can induce an altered architecture with increased crimp-formation. Lack of exercise, on the other hand, has been shown to induce formation of a multidirectional fiber network and a decreased crimp formation.
A Relaxed position: the myofibers are relaxed and the muscle is at normal length. None of the fascial elements is being stretched. B Usual muscle work: myofibers contracted and muscle at normal length range. Fascial tissues, which are either arranged in series with the myofibers or transverse to them, are loaded. C Classic stretching: myofibers relaxed and muscle elongated. Fascial tissues oriented parallel to the myofibers are loaded as well, as are extramuscular connections. However, fascial tissues oriented in series with the myofibers are not sufficiently loaded, since most of the elongation in that serially arranged force chain is taken up by the relaxed myofibers. D Actively loaded stretch: muscle active and loaded at long end range. Most of the fascial components are being stretched and stimulated in that loading pattern. Note that various mixtures and combinations between the four different fascial components exist. This simplified abstraction therefore serves as a basic orientation only.
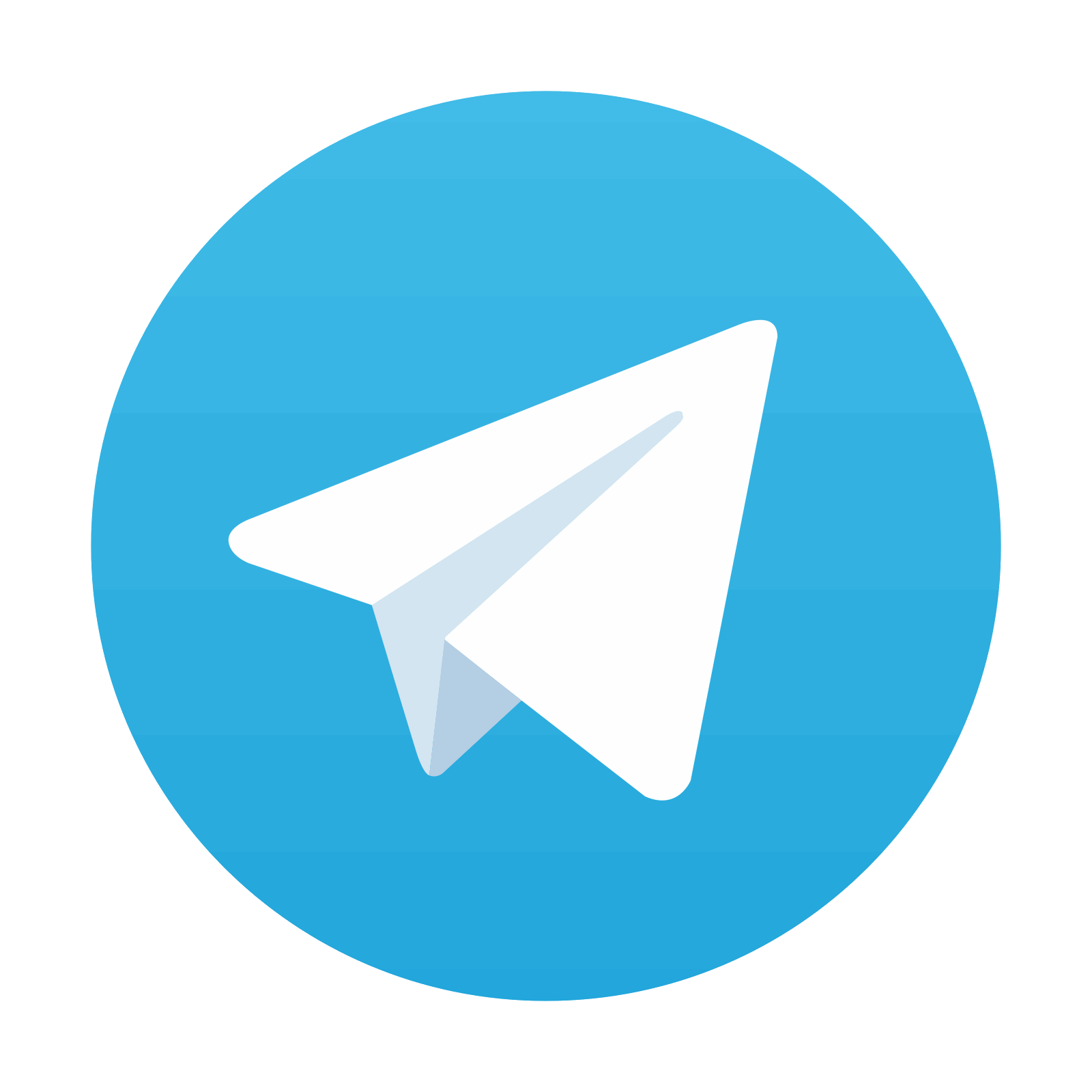
Stay updated, free articles. Join our Telegram channel

Full access? Get Clinical Tree
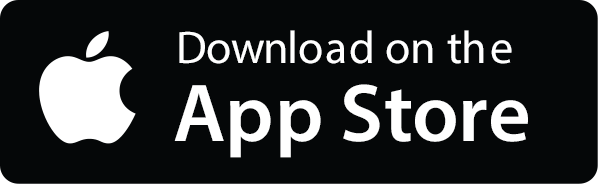
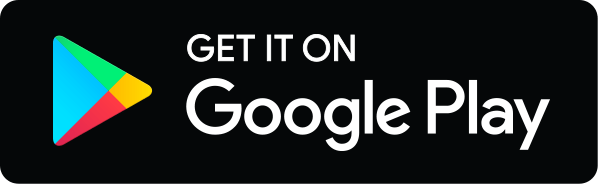