Failure Scenarios and the Innovation Cycle
Michael D. Ries
The Innovation Cycle
Innovation can be considered as a clever solution to a problem, but the success of the innovation is also dependent on many factors including the identification of the user’s needs which may be concealed (1). In the service industry, this can develop into a cycle which starts with process improvements to increase the efficiency of delivery of existing services, followed by process innovations which improve service quality, and then leads to product innovations through new types of services (2). If the innovation substantially affects supply prices may also decline (3). However, this type of theory is based largely on an economic model that does not necessarily apply to innovation in total hip arthroplasty since orthopedic implants are purchased by hospitals and paid for by insurance companies rather than the consumers.
Innovation in orthopedics may also be represented by a somewhat different sequence of events since the outcome of the innovation is often not known for some time after the introduction of new technology. The motivation for innovation in total hip arthroplasty includes a need to increase the durability of hip replacements, improve function, and reduce complications. Other goals of innovation include reduced healthcare costs and improved efficiency of healthcare delivery.
The effect of innovation in total hip arthroplasty has been one of incremental progression. The relative success of an innovation can be assessed by the length of time it takes the community to adopt the innovation for regular clinical use. Although innovations may be based on sound scientific principles and provide rational solutions to clinical problems, many innovations are not successful. However, unsuccessful innovations will be abandoned whereas successful innovations have remained in use in clinical practice. This results in incremental improvements over time in the quality and durability of total hip arthroplasty (Fig. 45.1) (4,5).
The Charnley HIP
The Charnley hip stands out as the single most important development in hip arthroplasty which fundamentally changed the treatment of patients with end-stage arthritis of the hip. There were prior attempts at hip arthroplasty, but these had not overcome the problem of achieving durable fixation of the implant to bone (6). Charnley introduced the use of polymethyl methacrylate bone cement and a low friction bearing surface (metal on ultra-high-molecular-weight polyethylene) to reduce stresses at the bone–implant interface which provided a reliable solution to this problem over 50 years ago (7). Ironically, if these innovations were developed today, they likely would require rigorous in vitro testing, animal experimentation, and randomized clinical trials to obtain regulatory approval for use in the United Sates. However, regulatory standards were much less strict at the time of development of the Charnley hip which permitted early adoption of this technology.
The Charnley hip femoral component was initially made from stainless steel, and early failures occurred as a result of fatigue fracture of the stem (8,9). The failures typically occurred at the proximal third of the stem where a fracture initiated at the anterolateral part of the stem. This represents the portion of the stem which is subjected to cyclic tensile stresses as a result of joint loading. Since this was a material failure, stainless steel was replaced with “superalloys” made of cobalt-chrome or titanium which have much more favorable fatigue strength. Although fractures can occasionally occur with modern cobalt-chrome or titanium stems, the failures are usually related to problems with implant design or surgical technique rather than the material properties of the substrate metal (10,11).
Cement Disease
Although cemented total hip arthroplasties have been highly successful, late failure of fixation occurred which was initially attributed to degradation of the cement bond and termed “cement disease.” Follow-up of early total hip arthroplasties with first-generation cementing techniques indicated that at 10 years of in vivo service femoral component loosening of 30% and acetabular component loosening of 11% were observed (12). Loosening was attributed to mechanical failure at the cement–prosthesis interface causing cracking
of the cement mantle or necrosis or fracture of trabeculae which had interdigitated with cement resulting in failure at the cement–bone interface (12,13).
of the cement mantle or necrosis or fracture of trabeculae which had interdigitated with cement resulting in failure at the cement–bone interface (12,13).
The high femoral component loosening rate prompted the development of improved cementing techniques. Instead of finger packing cement into the femoral canal, second-generation cementing techniques were developed which included a medullary plug, irrigation of the canal, use of a cement gun, and pressurization of the cement which resulted in reduced loosening rates (14). Techniques to improve the mechanical properties of the cement bond and reduce cement porosity were also developed. Vacuum mixing of cement has been an effective technique to reduce cement porosity which is still used today while other methods such as centrifugation of cement and low-viscosity cement have demonstrated beneficial effects in vitro, but have not been proven to be effective in vivo (15,16,17,18).
In vivo postmortem studies revealed debonding of the cement from the metal implant along with fractures in the cement itself (19). The femoral components that had been used were relatively smooth or polished and did not adhere well to cement. Roughened stems and stems precoated with polymethyl methacrylate bone cement were then developed in an effort to provide more durable bonding of the cement to the implant. However, early loosening and osteolysis occurred with some roughened or precoated stems because of abrasion between the rough stem surface and cement, causing cement debris to be produced (20,21). Cement debonding of polished stems has not necessarily been associated with clinical failure suggesting that the effects of debonding on late failure are related to both the surface finish and design of the implant (21,22). The ideal surface finish and implant geometry for a cemented femoral component remains controversial since a number of stem designs and surface finishes have been associated with variable results (20,21,22,23,24,25,26,27).
Porous Coatings
Although the etiology of loosening is multifactorial, the contribution of particulate wear debris-induced osteolysis was largely underestimated in the 1970s and 1980s. Since cement loosening was attributed to mechanical failure of the cement or adjacent bone, cementless porous-coated surfaces designed for ingrowth of bone were developed and implanted in large numbers since the early 1980s to avoid problems with cement fixation.
Porous-coated implants were shown to be effective in animal studies prior to use in humans (28) and the clinical results in primary and revision total hip arthroplasty have also been favorable (29,30,31,32,33). Ingrowth depends on a number of factors including surface properties unique to a given material type and the pore size and interconnectivity of the roughened or porous surface, as well as the postimplantation stability of the bone–implant interface, the distance between the bone and the implanted material, and the local biology of the bone bed. An appropriate constellation of these factors interact in ways that can provide durable long-term biologic fixation of the implant to bone through osteointegration.
The successful functional outcome as well as the durability of cementless total hip arthroplasty is influenced by implant design. Distally porous-coated cylindrical femoral
components have been associated with stress shielding and thigh pain (34,35). Modifications designed to reduce distal stem stiffness and extent of porous coating have resulted in improvement in thigh pain while still maintaining favorable osteointegration characteristics (36,37). Cementless modular acetabular components permit variable liner options, but require a liner-locking mechanism that is subject to micromotion and subsequent mechanical failure. First-generation modular cementless acetabular components achieved durable fixation to bone but also had a relatively high rate of mechanical failure at the liner-locking mechanism (38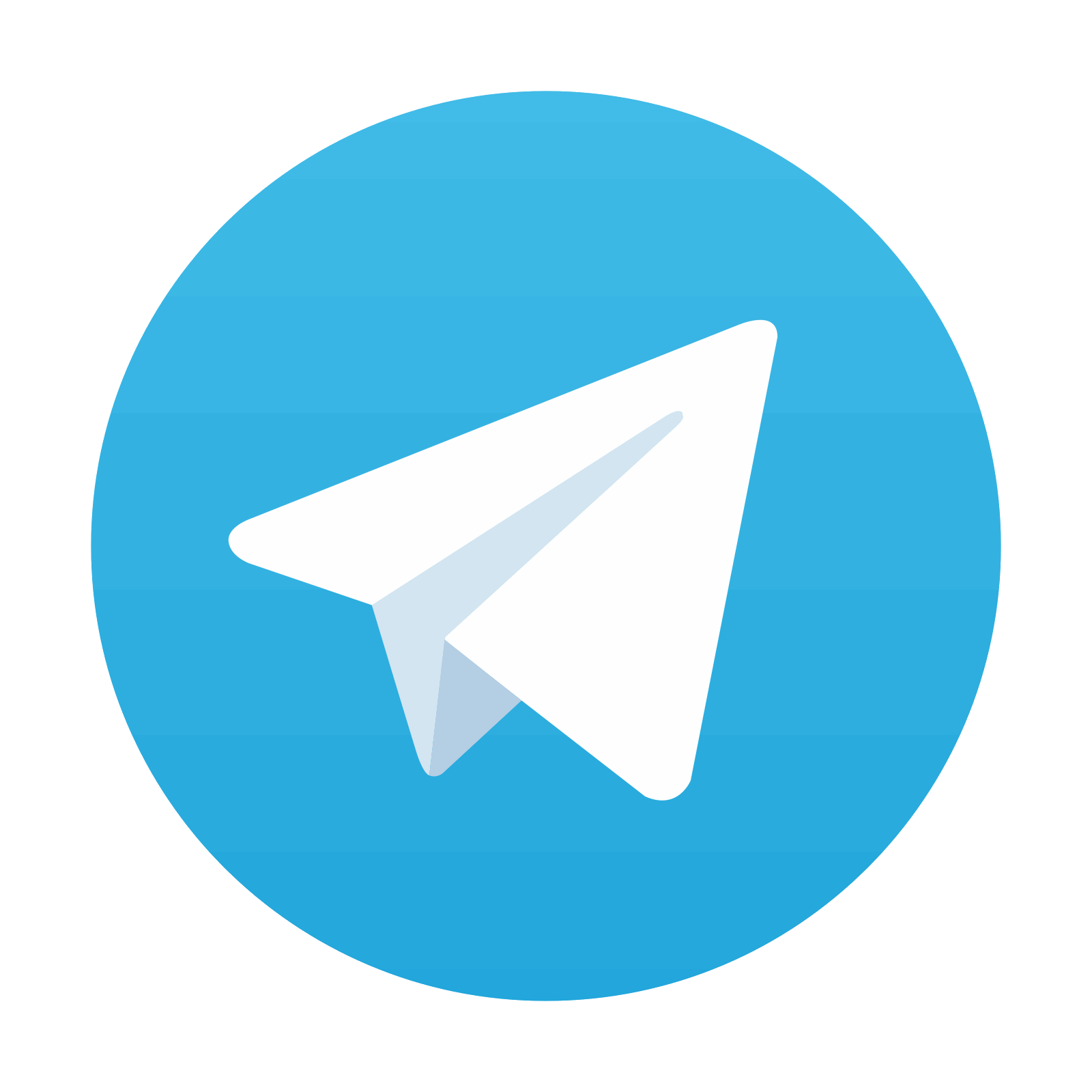
components have been associated with stress shielding and thigh pain (34,35). Modifications designed to reduce distal stem stiffness and extent of porous coating have resulted in improvement in thigh pain while still maintaining favorable osteointegration characteristics (36,37). Cementless modular acetabular components permit variable liner options, but require a liner-locking mechanism that is subject to micromotion and subsequent mechanical failure. First-generation modular cementless acetabular components achieved durable fixation to bone but also had a relatively high rate of mechanical failure at the liner-locking mechanism (38
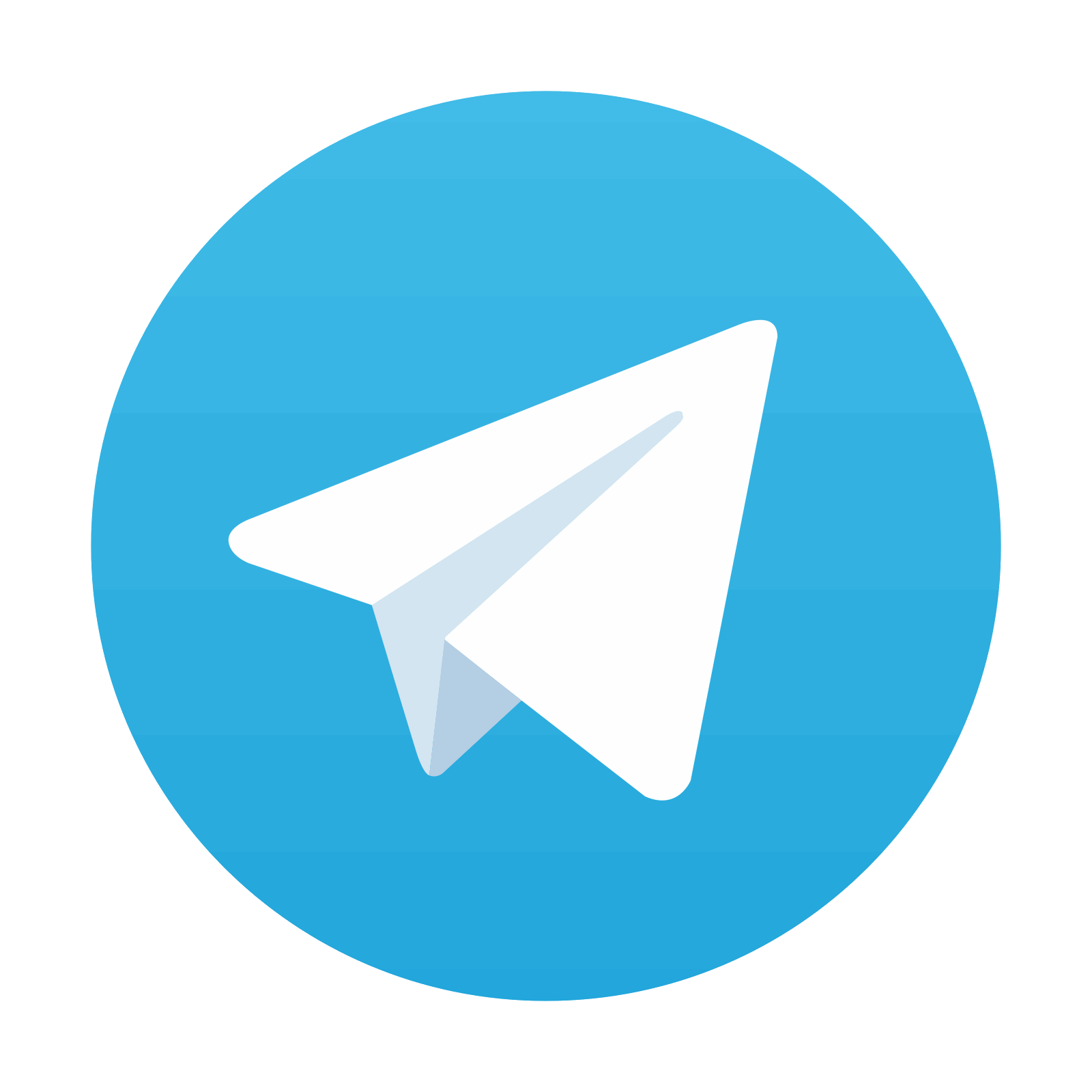
Stay updated, free articles. Join our Telegram channel

Full access? Get Clinical Tree
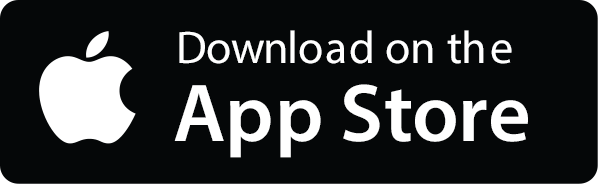
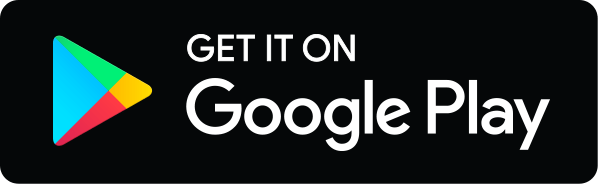