Evolving Concepts in Plate Fixation
It is questionable if surgery should not ever be done except by highly trained men, with highly trained assistance, and highly trained hospitals; otherwise disaster is likely to result.—A medical referee from the Aetna Insurance Company in 1928,1 after reviewing 34,753 compensation fracture files from Northern California
Plate osteosynthesis is still recognized as the treatment of choice for most articular and metaphyseal fractures and certain diaphyseal fractures. This chapter discusses when and how to use traditional compression, hybrid, or locked plates to achieve the best results during reduction and fixation of fractures.
History and Evolution of Plating
The first dynamic compression plate (the modified Collison plate) was introduced by George Bagby in 1956. This plate had oval holes, with the distal end of the hole having a vertical slot allowing for motion of the plate relative to the conical screw head.2 The Arbeitsgemeinschaft für Osteosynthesefragen/Association for the Study of Internal Fixation (AO/ASIF) was formed in 1958 and promoted four principles of successful fracture fixation: anatomic reduction, rigid internal fixation, use of atraumatic surgical technique on the soft tissue and bone, and early pain-free active mobilization during the first 10 postoperative days.3 Three years later, Müller introduced the outboard compression plate device.4 A round-hole bone plate was attached to the bone on one side of the fracture. On the other side of the fracture, a tension/compression device was attached to the bone and hooked onto the plate. A nut was tightened on the device causing compression across the fracture (Fig. 5.1).

Since the inception of the AO/ASIF, plates and techniques of plate fixation have evolved significantly, with the aim of improving fracture healing. In 1969, the dynamic compression plate (DCP) was introduced for fracture treatment, incorporating several improvements relative to the Bagby plate.5–7 The oval hole in the DCP design includes a ramp for the screw head that causes an eccentrically placed screw to “slide” 1 mm as it inserted, thereby provided a mechanism for compression fixation using the plate alone. The same plate can be used as a tension band, for neutralization or compression, or as a buttress plate. Other techniques such as pre-bending of the plate and lag screw fixation were also introduced to improve fracture compression and load contact sharing. These methods resulted in rigid fixation, and healing occurred by direct bone formation, with scarce radiographic callus formation.
The lack of callus in rigidly fixed fractures was described by Bagby and James2 and by others8 in animal experiments. At that time, callus was viewed as detrimental and considered to be a direct sign of instability, implant overload, or fretting corrosion.9 To follow the principles of exact anatomic reduction and absolute stabilization, a wide surgical exposure was necessary. Fracture fragments were often stripped of their soft tissues to achieve precise reconstruction of the bone. Furthermore, the stability of the plate–fracture construct using bicortical screws depends on friction between the undersurface of the plate and the cortical surface of the underlying bone.10 This friction increases as the torque of the screw increases,11 a concept that is important to remember when plating fractures in osteopenic bone.
During the early 1970s, investigators began to study the pressure exerted by plates on bones following compression plate osteosynthesis.12 They found that the plate exerts an average pressure to the bone of ~ 7,000 N/cm2, possibly leading to the observed radiographic bone loss, osteoporosis, and loss of vascularity under the plate.13
Subsequent to this work, the Zespol system was developed in Poland and reported on by Ramotowski and Granowski.14 This system represented the first internal fixator for stabilizing long bones. It used screw heads that locked into a plate, which was offset from the bone and could be used as either an internal or external fixator. The plate did not apply any direct pressure to the underlying bone, thereby preserving its vascularity and avoiding pressure necrosis.
In the early 1980s, Brunner and Weber15 introduced the wave plate (Figs. 5.2 and 5.3), and Heitemeyer and Hierholzer16 developed the bridge plate. These plates were designed to span the fracture site, with the plate fixed proximally and distally to the fracture. Such plates are still available.


The Limited Contact Dynamic Compression Plate (LCDCP) (Depuy Synthes, Paoli, PA), introduced by Perren et al17 in 1990, was designed to provide less than 50% contact between the plate and the bone (Fig. 5.4). Although there is decreased contact that could better preserve the blood supply to the underlying bone, there is no clinical study to date that shows improved rates of fracture healing, decreasing the need for bone grafting, or decreasing the incidence of infection and refracture (Fig. 5.5). For more than a decade, plating methods have focused on the principles of biological fixation.18 The Point Contact Fixator (PC-Fix) (Depuy Synthes) was designed with locking head screws and pointed areas of its undersurface and significantly less plate–bone contact than the LC-DCP (Fig. 5.6).10,19,20 Clinical trials using the PC-Fix titanium plates have suggested that the plates are associated with decreased rates of infection.21–23 PC-Fix–stabilized sheep tibia fractures have been shown to heal faster and with strengths similar to those treated with a DCP.20 Other mechanical studies have shown that the fixation achieved with the PC-Fix (locked screw–plate–bone construct) is stronger than the DCP (nonlocked conventional screw–plate–bone construct) when applied to cadaveric human femurs.24 The latest stabilization techniques have evolved to provide for more biologically friendly approaches.18 Although articular fractures require anatomic reduction and stabilization, the extra-articular segments of bone simply require restoration of overall limb alignment to maximize return to function. Wide dissection of multiple metaphyseal and diaphyseal fragments adds to the devitalization of the bone and should be avoided if possible.25,26 Successful fracture healing depends on ample blood supply to the injured tissues. Ideally, implant design should facilitate revascularization of the bone, and the surgical technique should minimize any additional injury.



Practical Applications of Screw Design
Screws have varied biomechanical function depending on how they are used. Small, 3.5-mm diameter, “rafting” screws placed beneath articular surfaces are used to prevent the subchondral bone from collapsing after reduction.27–29 In AO/Orthopaedic Trauma Association (OTA) type B partial articular fractures, screws are often used in conjunction with buttress plate fixation and calcium phosphate cement augmentation. In such constructs, screws can be used for rafting purposes as well as to provide interfragmentary compression.30–32 This so-called lag screw function can be accomplished by screws alone or in conjunction with plates. To achieve interfragmentary compression with a lag screw, the near cortex is drilled perpendicular to the fracture site with a drill whose diameter is equal to the major (thread) diameter of the screw. The far cortex is then drilled with a smaller-diameter drill equal to the minor (core) diameter of the screw. When the screw is inserted, the far cortex engages the screw threads. However, the screw glides through the near cortex. Therefore, when the head of the screw engages the plate or the near cortex, the bone is compressed between the screw head and the far cortex, creating compression.
In AO/OTA type C complete articular fractures, angular stability is not present, and to restore stability, screws must be used in conjunction with either locking plates or bicondylar plate constructs in order to avoid angular collapse (Fig. 5.7).

Compression Plating: When and How
Compression plating refers to the technique of using a plate with oval holes to create compression across a fracture site. The technique relies on the stability of the plate-to-bone contact surface because the screw heads are not angularly stable relative to the plate. The stability of traditional plates with bicortical screws relies on friction, which is proportional to the compression achieved between the plate and the underlying bone by the screw heads compressing the plate onto the bone. The screw heads and plate are compressed onto the bone by the screw threads as the screw is turned. Tension along the axis of the screw is created as the screw is tightened pushing the plate onto the bone. The pullout strength and insertion torque of the screw are important factors in fixation of the bone with conventional plating.11 In a study by Thiele et al,33 the authors found that the overall bone mass, thickness, and bone mineral density of the cortical layer were the most likely to affect the pullout strength of a screw in osteoporotic cortical bone. Because nonlocking screw constructs rely on the shear strength of the bone at the screw–thread interface, standard compression plate constructs in patients with low-density osteopenic bone are more likely to fail due to the relatively lower pullout strength of the screws than would a similar construct in patients with high-density bone and increased pullout strength. Bone geometry also plays a role in the strength of fixation. For example, the average pullout strength of 3.5-mm cortical screws in the tibial diaphysis is 1,710 N, the tibial metaphysis 471 N, and for the calcaneus 238 N.34 Moreover, the number of insertions of a screw in a given hole also decreases the pullout strength of the screw. In the tibial metaphysis, for instance, there is a marked decrease in pullout strength from 471 N to 382 N from the first to the third insertion of a screw into the same hole. Therefore, surgical technique of screw insertion is paramount for successful fracture fixation with compression plating.
When a patient applies a mechanical load that exceeds the strength of a compression plate construct, angular collapse occurs across the fracture site. This collapse is due to the lack of angular stability and subsequent motion (toggling) between the plate and the screws. With conventional plates, unicortical screws have a significantly lower load-carrying capacity than bicortical screws.35
Compression plating across a noncomminuted fracture site can be achieved in two ways. The first method begins by stabilizing the plate with screws on one side of the fracture. On the other side of the fracture, the bone is drilled and a screw is inserted in the oval hole of the plate off-center and away from the fracture site. The oval hole in the plate incorporates a ramp so that as the eccentrically placed screw is tightened, the screw head moves toward the fracture, moving the bone with it and causing fracture compression. The second method also begins with the stabilization of the plate with screws on one side of the fracture. Then, on the opposite side of the fracture, the plate is pulled way from the fracture either by a separate tension/compression device or a reduction clamp, both of which use a push/pull screw that is placed at an appropriate distance from the plate. Screws are then placed through the plate, stabilizing the bone while compression is maintained at the fracture site. These techniques work well in axially stable short oblique and transverse fracture patterns.
Neutralization, Bridging, and Buttress Plating: Use of Plates as Reduction Tools
Video 5.1 Distal Radius Dorsal Wrist Spanning Plate
Some fracture patterns, such as spiral, oblique, or bending fractures with butterfly fragments, can be reduced with repaired with screws alone. However, screw fixation alone is not sufficient to neutralize out-of-plane or torsional forces that occur during loading, nor is isolated screw fixation strong enough (in most cases) to allow full weight bearing. In such cases, a so-called neutralization plate spanning the fracture zone can be used effectively to stabilize the fixation construct. In more comminuted fractures a bridging screw–plate construct can be used. In this type of construct the comminuted fracture zone is “ignored” and a plate strong enough to withstand local bending forces is rigidly fixed to the bone proximal and distal to the comminuted zone (typically six to eight cortices fixed on each side).36
In fracture patterns that involve shearing forces, a plate can be used to buttress against these forces and to facilitate more stable fixation. An example of an application of a buttress plate is the tibial plateau buttress plate. The use of a nonlocking lateral condyle buttress plate provides significant stability to the lateral condyle piece. However, additional subchondral rafting screws are needed to buttress any reduced articular fragment. The use of a nonlocking medial condyle buttress plate can significantly increase the stability of fixation constructs for bicondylar tibial plateau fractures.37 Other examples of buttress plating include distal femoral buttress plate,38 proximal humerus,39 femoral neck,40 and distal radius.41
Finally, plate precontouring can be used as an aid to fracture reduction or to shorten the operative time. Plates can either come as precontoured anatomic plates by the manufacturer or be self-made by the surgeon base on a plastic model and sterilized prior to surgery.42 A straight plate can also be used as a reduction tool when used as an antiglide plate.43 In this technique the reduction is obtained by one bone fragment being pulled down to the plate, creating an axilla into which the second bone fragment is allowed to “fall.” Compression of the two fragments is then achieved using either an articulating tensioning device or the dynamic compression holes.44
Locking Screw–Plate Constructs
Video 5.2 Rules of Locked Plating
There are significant biomechanical differences between traditional compression plating and fracture stabilization with a locked screw–plate construct. As described above, the stability of traditional bicortical screws relies on obtaining compression between the plate and underlying bone, and creates tension along the axis of the screw that is transferred to the bone as shear stress. As the screw is tightened, there is an increase in compressive force between the plate and the bone, which generates friction between the two and allows for applied force to be transferred along the plate. A tight frictional interface, which is dependent on the adequate pullout strength and insertion torque of the screws, is paramount for successful load transmission. Such constructs rely on the shear strength of the bone at the screw–thread interface. In patients with high-density bone, higher forces can be generated than in patients with osteopenic bone. When a patient applies a load that exceeds the fixation capacity of the construct, collapse occurs across the fracture site. This collapse is due to the lack of angular stability and subsequent motion between the plate and the screw.
Unlike traditional plating systems utilizing bicortical screws, locked screw-plating systems have angular stability, increasing their load-carrying capacity (Fig. 5.8). The angular stability results from the threaded screw head that is locked into the threaded plate hole, forming a fixed-angle construct. Functionally, each locked screw behaves like a miniature blade plate. The locked screw–plate construct enables load transmission from the screw to the plate and relies on frictional forces applied from the threaded screw head to the threaded screw hole in the plate. Although the loads applied to conventional and locking plates are the same, the preloads necessary for construct stability are by necessity higher in the conventional plates and screws.

Mechanical studies showed the advantage of angular stability between a locking plate and screws.12,13,26,38 In a study that evaluated the mechanical properties of unicortical locking screws compared with conventional bicortical plating in cadaveric sheep tibia, no differences in torsional or bending strengths of the constructs were found.20 However, it was noted that the fractures that were stabilized by locked plates failed by pullout of the entire construct compared with the bicortical screw plates that showed individual screw pullout. Another distinct advantage of locking plates is that the fixation strength of the plate–screw construct is less affected by cortical density. Wallace et al45 investigated the relationship between cortical thickness in a proximal humerus fracture gap model as related to the pullout strength of locking screw constructs. They found that the load-at-failure, stiffness, maximum load, and fracture gap closure did not vary as a function of cortical thickness. Similarly, even with stripping of the screw hole, or over-drilling of both the near and far cortex (situations in which nonlocking screws completely fail), locking screws confer marked advantage in endurance, bending, and torsional stiffness.46
Several investigators have shown that there are specific configurations of locking plates that increase the overall rigidity of the constructs. A study analyzing the configuration of screws with both in vitro and finite element models illustrated that the near-near, far-far concept of pin placement in external fixators also applies to screw placement in locking plates, which are also commonly referred to as internal fixators.47 Using 12-hole locking plates with a gap and standardized homogeneous composite cylinders, Stoffel and colleagues47 were able to elucidate the extent to which an internal fixator can provide stability. The gap size, working length, number and position of screws, plate length, and material properties of the implants were tested for fatigue, axial stiffness, and torsional rigidity. The authors found that using a 12-hole plate with locking screws in the 1, 2, and 6 positions on either side of the fracture gap provided the most significant rigidity. Additional stability is conferred with decreasing distances from the plate to the bone and with increasing lengths of the plate (increased working distances).
External fixators become more rigid with the increased proximity of the connecting bars to the bone. Locked plates or internal fixators can be seen as an external fixator that is positioned very close to the bone, thus offering a very rigid construct that does not compromise the periosteal blood supply, as compression plates do, and is not subject to the infectious complications of external fixators. Additional parameters that influence the stiffness of the locked plate construct are the length of the plate, its cross-sectional area, and the material properties of the plate, as well as the proximity, density, diameter, and cortical configuration (unicortical versus bicortical) of the inserted screws.48
A misunderstanding of the biomechanical principles governing the use of locked plates may lead to failure of fixation. Fracture stabilization with locked plating relies on relative stability and secondary bone healing for its success. Allowing a construct to become too rigid without first anatomically reducing and compressing the fracture fragments may lead to nonunion. When the ends of a simple fracture are brought within close proximity and fixed to a plate with locking screws without first compressing the fragments, the stiffness of the locked plate construct prevents bone contact, while the interfragmentary strain at the persistent fracture gap is high. These conditions inhibit the formation of bone. It has been shown that fracture gap strain must be kept between 2% and 10% for optimum secondary bone healing (endochondral ossification) and callus formation.49
Distal femur fractures, normally considered a fracture zone that is not prone to healing complications, have proven to be highly sensitive to the rigidity of fixation when locking plates are used. The reported rate of complications related to healing ranges from 0% to 32%, with nonunion being reported in up to 20% of distal femur fractures treated with locked plates.50 Comminuted fractures with locking screws near the fracture site were in particular risk for nonunion.50 When compared with intramedullary nails, locking plates reduce the amount of callus formation in the treatment of distal femoral fractures.51 In a study of 64 consecutive patients with distal femoral fractures treated with locking plates, the callus formed in the distal femur tended to be asymmetric, whereas most of the callus is formed on the medial cortex.52 In the same study, titanium plates allowed more callus formation than stainless steel plates, whereas the spanning bridge length (the length of the plate without screws bridging the fracture zone) did not have a significant effect on callus formation.52 A detailed description of the surgical techniques for the repair of distal femoral fractures is provided in Chapter 29.
Adjunct plate augmentation techniques using combination locking hole fillers that do not thread into the locking hole do not increase plate stiffness and are not utilized frequently.53 However, two studies in which locking screw holes were filled with locking screw heads or locking screws showed that there was increased stiffness and fatigue life of the locking plates.54
Newer “hybrid” plate–screw systems enable surgeons to incorporate both locked plating and compression plating into one implant. Although this option enables surgeons to take advantage of both types of fixation strategies, it also has the potential to negate the theoretical advantages of each if used incorrectly. When using hybrid plate constructs, nonlocking screws are typically placed first to compress fracture fragments or facilitate reduction by “pulling” the plate down to bone. Locked screws are introduced last to increase the rigidity or angular stability of the construct. A study showed that in a hybrid plate construct, at least two locked screws should be placed on each side of the fracture in order to maximize stiffness and stability as well as prevent loosening.55 Adding conventional screws to a construct significantly increases its bending strength compared with an all-locked construct. By replacing the outermost locking screw with a conventional screw, it is possible to reduce the stress concentration at the plate end and potentially decrease the rate of periprosthetic fractures.56
Traditionally, with locking plate constructs there is increased motion at the fracture gap on the opposite side of the plate, and decreased motion on the same side as the plate. There is some initial evidence that uniform motion across the fracture gap may be beneficial. The Dynamic Locking Screw (Depuy Synthes) reduces the plate–screw stiffness but increases uniform micro-motion at the fracture site.57 Clinical evidence is not yet available regarding the clinical benefits of this approach. Another way to modulate the motion seen at the fracture site is by creating near-cortical slots in locking plate constructs (also referred to as “far-cortical locking).58 In a preliminary study, Gardner et al58 illustrated that simple modification of the near cortex with slots decreases the construct stiffness but does not sacrifice fixation under cyclic loading. Like the dynamic locking screw, this simple technique facilitates modulation of stiffness at the fracture site.
Unfortunately, it is currently impossible for the surgeon to measure the optimal stiffness of a locked plate construct for a given fracture pattern. However, the available literature suggests that when locking plates are used, bone healing is more reliably achieved with less rigid constructs. A common approach to achieve this goal is to increase plate length and decrease screw density.59 Factors that affect the rigidity of fixation include the length of the plate, the screw density, and the distance of screws to the fracture. It is recommended that the length of a locked plate construct be eight to ten times the length of the fracture in simple patterns and three times the length in comminuted fracture patterns. The length of the plate can also be chosen considering the number of screws needed in the diaphysis. Usually, at least four screws are placed in the diaphysis proximal to the fracture. A longer plate is beneficial because the internal stresses within the plate decrease as the most proximal screw in the plate is farther from the fracture. If a fragment of bone is to be fixed and not bridged, then at least two screws should be inserted into that fragment. Bicortical screws should be utilized in all holes. A screw-to-hole ratio less than 0.5 limits the bending moments experienced at the most proximal and distal screws, and a span of two to three open screw holes should be left over the fracture site to help limit the concentration of stress at the adjacent screw–bone interfaces. The construct stiffness can also be modulated depending on diaphyseal locking screw placement. If a stiff construct is desired, then a near-near, far-far screw location is chosen, with two screws close to the fracture and two screws further from the fracture. If a more flexible construct is desired in patients with multifragmentary metaphyseal fractures, screws can be spaced farther from the fracture. The proximal most screw can be unicortical as long as there is sufficient fixation in other locations.
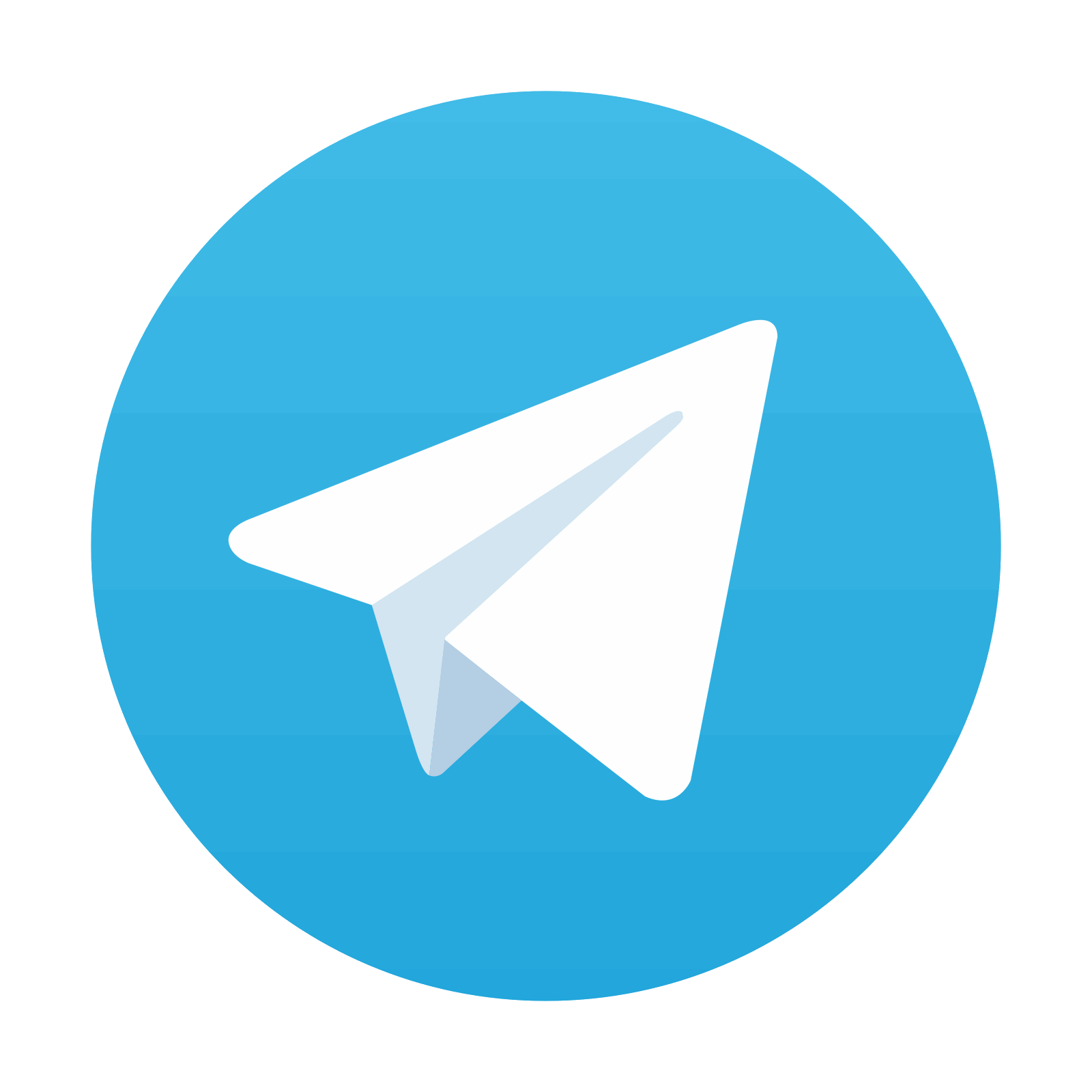
Stay updated, free articles. Join our Telegram channel

Full access? Get Clinical Tree
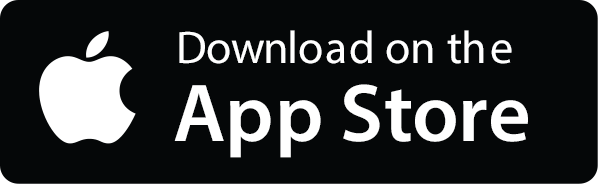
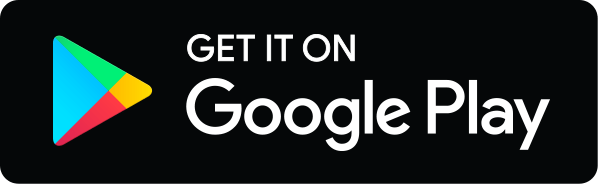