Emerging treatments for fractures and nonunions—growth factors and beyond

Introduction
The intrinsic capacity of bone healing is well-known. It involves a complex orchestrated sequence of molecular and cellular events. Use of transgenic animal models has increased our understanding of this biologically optimized process. During bone healing thousands of genes are up- or down-regulated [1, 2]. Key players in the bone-healing response are growth factors, small polypeptides that are produced by the cells themselves and excreted into their microenvironment.
Efforts in the last decades have mostly focused on the specific temporal and spatial expression of the growth factors that drive fracture healing. Many growth factors have been investigated in regard to their potential role in bone healing, such as fibroblast growth factor (FGF), platelet-derived growth factor (PDGF), transforming growth factor-β (TGF-β), insulin-like growth factor (IGF), and bone morphogenetic proteins (BMPs) [3]. To date, most evidence has suggested that of all these growth factors, the BMPs are most crucial to skeletal development, growth, homeostasis, and repair. As 5–10% of all types of fractures do not heal properly, it is obvious that the opportunity to stimulate fracture healing by adding BMPs or modulating its local expression has been greatly anticipated ever since their discovery.
Bone morphogenetic proteins (BMPs) and nonunions
As early as 1954, Urist et al wrote that “bone tissue is ever turned over” and that “there is no definite time after which a pseudarthrosis cannot unite simply by prolonged immobilization” [4]. He noted that a new proliferative process starts after any surgical operation, and after implantation of any type of bone graft. In 1965 he discovered an osteo-inductive capacity that could induce bone formation upon implantation in ectopic sites in animals by decalcifying bone matrix [5]. This discovery ultimately led to the cloning of the bone morphogenetic protein (BMP) in the late 1980s [6].
Since then it has become apparent that BMPs might have clinical value in accelerating bone healing and in the treatment of bone defects and nonunions. After countless studies, the clinical application of BMPs in fracture and nonunion treatment and in specific spinal procedures was finally approved in early 2000s. It was expected that local application of BMPs would increase and/or accelerate bone healing and formation. Two large randomized clinical trials involving open tibial fractures and tibial nonunions introduced the use of BMPs to the orthopaedic traumatologist [7, 8]. Results in these trials seemed encouraging, but flaws in the methodology have since undermined their conclusions.
The BMPs that are currently commercially available are for specific clinical use only. Recombinant human bone morphogenetic protein-2 (rhBMP-2) can be used for open tibia fractures at risk for nonunions and rhBMP-7 or osteogenic protein-1 can be used for treatment of recalcitrant long-bone (tibia) nonunions. Since their FDA (Federal Drug Administration) approval, their use has sky-rocketed in the US and Europe [9–19] (for review of clinical data until 2005 see [20]). It should be noted that both factors have also been extensively used “off label” (85% of applications). Despite their excellent results in animal studies, so far the effects of BMPs in human fracture and nonunion healing have been somewhat disappointing [20–23].
Mechanism of BMP in bone healing
BMPs are members of the TGF-β superfamily [21, 22]. Their roles in bone healing involve stimulation of blood vessel formation (angiogenesis), proliferation, differentiation, chemotaxis, and cell adhesion. Nowadays more than 20 BMPs are known. Not all have the unique property of promoting de novo bone formation and bone healing. The most important BMPs in the skeleton are BMP-2, -4, -6, -7, and -9. These BMPs are all able to induce undifferentiated mesenchymal stem cells to differentiate into osteoblasts and chondroblasts. BMPs elicit their effects through binding to specific transmembrane receptors. These receptors, once activated by the bound BMP, subsequently phosphorylate and activate so-called Smad proteins that are located intracellularly. These Smad proteins translocate into the nucleus where they regulate gene transcription.
Genes that are stimulated by BMP-activated Smads (eg, Runx2 (CBFA1)) are critical for osteoblast differentiation. After a fracture, the various osteogenic BMPs are strongly up-regulated in the periosteum near the fracture site, followed by a gradual reduction in expression. Recent studies suggest BMPs function to control fracture healing by inducing differentiation and proliferation of chondrocytes and osteo-blasts. Definitive evidence that BMP-2 controls fracture repair was obtained from a study using a limb-targeted deletion of BMP-2 which showed no healing [24].
BMP signaling is tightly regulated at various levels both outside and inside the cell. BMP antagonists have been identified in the extracellular space that directly bind BMPs, preventing them from binding to their receptors (eg, Noggin, Chordin, and Gremlin). In addition, transmembrane (BAMBI), intra-cellular (Smad-6 and Smad-7), and intranuclear inhibitors (c-Ski, Smurf 1/2) exist that can block BMP activity [25, 26]. The BMPs induce their own inhibitors, providing negative feedback mechanism to hamper their own activity. The activity of surgically administered BMPs will modulate these natural inhibitors [27].
Evidence for clinical use of BMP in bone healing
Numerous clinical series have now been published using exogenous BMPs for fracture and nonunion treatment. The author reviewed the clinical literature in 2005 and concluded that evidence was very limited with methodological flaws in the two available RCTs [20]. Since this review in 2005 many studies have appeared, most of them being case series [9–19]. Many of the patients in these series had undergone various procedures and were “desperate” cases. Understandably, their treatment not only included BMP, but also often revision osteosynthesis, application of local autogenous bone grafts, or other adjunctive therapies. This has been coined polytherapy [28]. Within these polytherapy cases it is difficult to determine the specific role of BMP.
A recent Cochrane review on the use of BMPs in fractures and nonunions came to the same conclusion [29]. The authors highlighted the paucity of data on the use of BMP in fracture and nonunion healing, as well as heavy industry involvement in the available RCTs and economic evaluations [29]. Of note is that an eagerly awaited trial (not included in the Cochrane trial) on the use of rhBMP-2 in acute open tibial fractures recently showed a higher infection rate in the BMP group versus control (no bone graft) and no improvement in rate of fracture healing [30]. This unexpected result will certainly add to the controversy on the use of BMP in trauma.
Complications of clinical BMP use
Off-label use in periarticular locations (eg, tibial plateau fractures, distal humeral nonunion) has been related to bone overgrowth or heterotopic ossification, some of which needed reoperations [31, 32]. Swelling, hematoma formation, and bony resorption were noted in spine applications. A recent paper showed that BMP is used in approximately 25% of spine fusions in the USA. Complications seem to be more frequent for anterior cervical fusions and with greater hospital charges for all categories of fusions [19, 33].
Economic evidence
Cost effectiveness has been evaluated by at least four groups [29]. The cost of exogenous BMP is high (around $ 5,000 per treatment). So far there is limited economic evidence that the use of BMP for acute open tibial fractures may be more favorable economically when used in the most severe injuries. No such evidence exists for the use of BMP in non-unions or other fractures [29].
In summary, the excitement over the potential clinical applications of BMPs in fracture and nonunion treatment has somewhat subsided due to lack of compelling clinical evidence. It is unclear why the impressive result in preclinical studies cannot yet be replicated in patients. There is a clear species-specific dose response with the dosage used in humans (several milligrams) being a 106-fold (!) higher than the endogenous amount (nanograms). A potential explanation may be related to the lack of target cells, presence of BMP-inhibitors, short half-life, diffusion, and/or suboptimal delivery vehicles.
Second-generation BMPs that are currently under investigation might be more potent, safer, and cost effective [27]. In addition, at least part of the answer might lie within the complexity of the BMP signaling pathway. BMP action is driven by many factors, including receptor-binding affinity, expression of ligand, and receptor and the expression of BMP coreceptors and antagonists.
In any case, it seems unlikely that adding a single BMP can resolve a nonunion. The complexity of growth-factor synergy and inhibition is too great. For now, it might very well be that a combination of BMPs (eg, BMP-2 and BMP-7) is more potent. Unfortunately, this strategy may never be realized, given that the commercial rights for these two BMPs are owned by different companies. Also, testing of a combination product is more complex than testing one growth factor at a time.
An alternative approach might be to maximize the effects of exogenous and endogenous BMPs by inhibiting the inhibitors. Application of supraphysiological dosages of BMP as currently used might lead to a dose-dependent increase of BMP-inhibitors limiting their functional therapeutic application. Minimizing these negative effects could be achieved by pharmacologic or antibody/protein-mediated regulation of the BMP antagonists, their upstream modulators, or both [23]. Recently, an alteration was shown in the balance of BMPs and their inhibitors in cartilaginous areas of fractures with different clinical outcomes [34]. This may impair the transition from cartilage to bone formation, which is an integral part of the endochondral ossification pathway. Elevated levels for BMP-inhibitors were indeed noted in nonunions [35].
Other potential clinical approaches to increase local and sustained presence of BMPs involve gene transfer and tissue engineering. As shown in animal studies, these hold great promise. Progress of these techniques towards a clinical trial is, however, slow and very expensive [36].
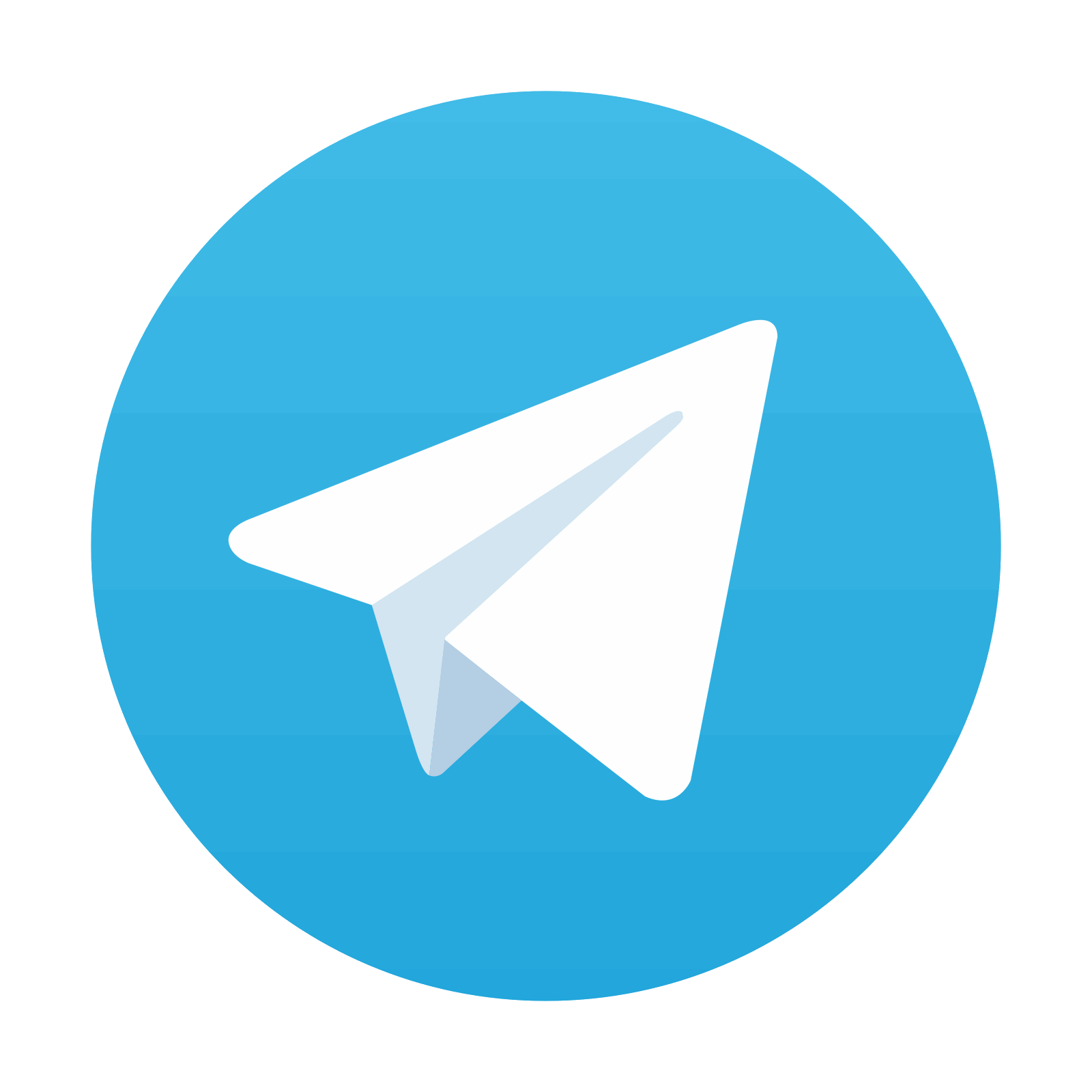
Stay updated, free articles. Join our Telegram channel

Full access? Get Clinical Tree
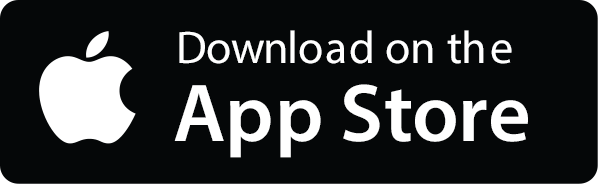
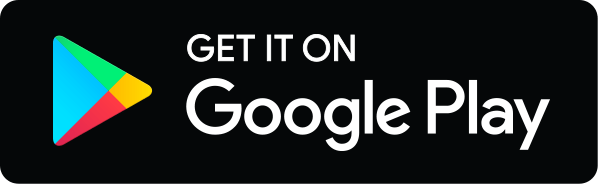
