12
Electrothermal Chondroplasty
Few effective options are available to the orthopedic surgeon in the treatment of advanced chondromalacia and arthritis following failure of medical management. Currently, lavage1–3 and debridement,4–6 repair stimulation techniques,7,8 cell and tissue transplantation,9–11 osteochondral plugs,12–15 and biologic and synthetic matrices16 are options in the treatment of arthritis. Cartilaginous defects that do not penetrate through to the subchondral bone show little or no reparative response.17,18 It is believed that grade I, II, and III lesions propagate through a process of delamination, fragmentation, or fibrillation.19 This degradative process can be slowed in grade II, III, and IV lesions with debridement of the loose cartilage to a stable rim. Generally, this is accomplished by the use of mechanical instruments such as rotary shavers, hand instruments, or thermal devices such as lasers and more recently the use of radiofrequency energy (RFE) probes.
Mechanical debridement with shavers and probes has been the mainstay of cartilage debridement and regeneration but it is not without deleterious effects.20,21 It results in fine surface fibrillation as well as iatrogenic defects involving normal cartilage of various sizes. Cartilage regeneration can be accomplished by abrading the subchondral bone, which results in subchondral bleeding and formation of fibrocartilage, which is not as functional as normal hyaline cartilage.22
Thermal energy used to debride cartilage was initially attempted with laser, and it showed some early promise.23,24 However, the depth of thermal damage and healing response following application of lasers has been a concern.25 Histologic and biochemical studies on rabbit articular cartilage after laser treatment confirmed extensive damage reducing cell viability and expression of proteoglycans.26 Another study of acute and chronic response of articular cartilage to holmium:yttrium-aluminum-garnet (Ho:YAG) laser treatment confirmed no healing of full- or partial-thickness defects in hyaline cartilage.27 At present the healing of articular cartilage following laser energy application remains controversial.
Thermal chondroplasty with RFE has become tremendously popular over the past few years. It is believed that up to 10,000 procedures are being performed monthly. RFE chondroplasty has gained popularity based on anecdotal reports of clinical success and lack of peer reviewed prospective clinical studies.
Several basic science studies have shown the effect of bipolar (bRFE) and monopolar (mRFE) radiofrequency energy on animal and human cartilage models.21,28–38 Chondrocyte death has been observed following RFE chondroplasty and remains a concern in the clinical setting.29–31,35–38 It is believed that thermal chondroplasty alters the mechanical and or structural properties of the superficial layer of the articular cartilage that slows or possibly stops the degenerative cycle.21 Some hypothesize that RFE may also reduce the release of collagen and proteoglycan epitopes into the synovial fluid, thus reducing the cycle of cartilage degradation, synovial inflammation, and further articular degeneration.30 Radiofrequency energy is an inexpensive tool that is easy to use for the surgeon and operative personnel, and it can be delivered arthroscopically with a wide variety of probes that offer extended flexibility to the surgeon. In addition, mRFE has the advantage of providing temperature-controlled application, thus potentially widening the margin of safety when treating intraarticular structures.30,35–38 Nevertheless, application of RFE for chondroplasty in the clinical setting should proceed with caution until more basic and clinical studies can confirm the efficacy and safety of these devices.
Radiofrequency Energy
There are two basic types of radiofrequency (RF) circuitry for use in orthopedic applications: monopolar and bipolar. The tissue temperature profiles for these two systems are vastly different. The RFE oscillates the electrolytes in the intracellular and extracellular solutions producing molecular friction.
In the monopolar setting, the current flows from the current generator (Fig. 12–1), which takes power from a line source such as 110 V at 60 Hz, boosts the power to as high as 600 V or even greater, and transforms it into an alternating current at 460 kHz by oscillating a crystal tuned to 460,000 on/off per second. The current passes from the box through the connecting cable, through the probe (active electrode) into the patient, through the patient to the return electrode (indifferent electrode or ground pad), and back to a return within the box. Because the patient’s tissue has a higher resistance than the rest of the circuit, heat is produced at the point where there is a step-up in the resistance. During chondroplasty, the RFE may choose to pass from the probe through the cartilage surface and subchondral bone to the grounding plate on the skin, or from the probe through the irrigation solution to the joint capsule and then to the grounding plate. The path selected is most likely determined by the impedance encountered as it passes along the cartilage surface and may be influenced by the cartilage thickness, water content, proteoglycan concentration, collagen content, subchondral bone thickness, and the conductive characteristics of the irrigation solution selected.30 Primary heating occurs in the upper layers of the tissue, which then heats the deeper layers of tissue.39 The temperature is monitored 50 times per second, providing a constant feedback to the RF generator that automatically adjusts power output accordingly, much like the thermostat in a room. The gradient of thermal effect seen with monopolar RF arthroscopy is capable of achieving a deeper tissue response if necessary.40
FIGURE 12–1 The Oratec Generator and TAC C probe.
This is in distinction to laser energy, which produces heat through direct photostimulation of cellular ions, and bipolar RF, which creates a conduction path through the arthroscopy fluid rather than through the treated tissue. The current produced by bipolar RF follows a path of least resistance from the probe tip, through the conductive irrigating solution, and back to another conductive electrode a very short distance away. High temperatures between bipolar probe electrodes are reached as the current “arcs” between them heating the surrounding solution. The heated solution provides high surface temperatures with limited ability to penetrate deeper tissue. Less current is required with a bipolar device to achieve the same effect, because the current passes through a much smaller volume of tissue. The thermal effect of RFE is determined by the level of energy used, duration of treatment, type of tissue treated, and type of electrode used. In addition, the extent of thermal modification is operator dependent and due to the lack of tactile response in the no contact approach, it relies on visual observation of the tissue response.
Basic Science of Thermal Chondroplasty
The Ho:YAG laser has been used to treat chondromalacia,21 but concerns of avascular necrosis in the clinical setting and large zone of thermal injury in animal studies22 has resulted in limited use of this approach. Lane et al26 performed matrix assessment in a rabbit model following Ho:YAG laser treatment of articular cartilage and found that extensive articular cartilage damage is caused by the laser. Biochemically, the amount of glycosaminoglycan in the treated cartilage, and the amount of sulfate incorporation into proteoglycans was significantly less in laser-treated articular cartilage than controls. In addition, subchondral changes including bony architectural damage, blood vessel membrane damage, and lack of osteocytes in lacunae was also observed. They postulated that cell viability was greatly reduced, and the subchondral changes could possibly explain the clinical avascular necrosis observed in some cases. Another study of acute and chronic response of articular cartilage to Ho:YAG laser treatment confirmed no healing of full- or partial-thickness defects in hyaline cartilage.27
Several in vitro and in vivo studies have demonstrated the ultrastructural and histologic changes that occur after mRFE treatment of capsular tissue.39–45 However, studies on RFE chondroplasty are limited. Peer-reviewed prospective clinical outcome studies have not been published, but basic science studies of the effect of RFE on human and animal cartilage models have been published.21,28–38
A study compared rotary shaver debridement of articular cartilage with that of a bipolar RF probe on distal femur of sheep. A modification of Mankin’s scoring system using six histologic variables was evaluated in terms of favorable and unfavorable response to treatment. The outcome was superior for the bipolar probe, and in addition, viability of remaining cartilage with less destruction of normal architecture was noted.21 However, this study did not report on the power settings and energy delivery and did not use the currently accepted methods for evaluating cell viability. In addition, a major shortcoming of this study was that the cartilage defects did not represent degenerative chondromalacia changes. Instead, the investigators created a fracture in healthy cartilage and used this to simulate the changes seen with grade II and grade III chondromalacia.
To address this concern, Kaplan et al33 evaluated the effects of RF ablation on freshly acquired human knee cartilage from patients undergoing total knee arthroplasty. Although cartilage smoothing was obtained, the authors discovered no increased incidence of chondrocyte death in the adjacent areas after the debridement. They concluded that the probe did not cause any significant injury to the surrounding tissue. The shortcomings of this study include the use of insensitive techniques of light microscopy with hematoxylin and eosin and periodic acid-Schiff staining to determine cell viability. In addition, the authors recognized that their study examined the changes at time zero, and that the need for additional evaluation of this technique to determine longterm chondrocyte survival and function was necessary. A case report of a second-look arthroscopy 1 year following bipolar chondroplasty revealed a scalloped cavity with appreciable thinning of the articular surface in the treated region.46 This raises concern about the delayed appearance of injury to the chondrocytes with subsequent formation of a depression in the articulating surface. The authors of this chapter have also encountered similar isolated cases.
Lu et al35 evaluated in vitro chondrocyte viability and surface contouring of human articular cartilage using confocal laser microscopy (CLM) and scanning electron microscopy (SEM) after treatment with different time intervals of RFE and bRFE. Monopolar RFE probe was applied in light contact without flow and the bRFE probe was applied noncontact with flow as per manufacturers recommendations. The authors found that bRFE caused significantly greater chondrocyte death than did mRFE at identical treatment time intervals. The SEM results showed that mRFE created a smoother surface than bRFE at 5-second application, but no significant differences were seen at longer application intervals. At a 15-second interval the cartilage met the authors’ definition of optimal contouring based on SEM grading system. The authors feel that arthroscopy may overestimate the degree of smoothing given its low magnification, but it remains unclear if SEM grading correlates with clinical outcome. In a previous study, Lu et al30 compared two bipolar and one monopolar RFE probes. Each probe type was applied to the cartilage using a controlled mechanical jig, noncontact mode, and paintbrush pattern. A higher chondrocyte death in the two bipolar probe types was confirmed. In addition, the bipolar systems penetrated to the level of subchondral bone in all osteochondral sections during arthroscopically guided paintbrush pattern treatment. Monopolar probes did not penetrate the subchondral bone in the three modes of application studied. In summary, the researchers concluded that RFE should be used cautiously for thermal chondroplasty until viability of chondrocytes could be improved. In addition, a recent study reported that the lavage solution temperature may have significant influence on chondrocyte viability when thermal chondroplasty is being conducted.38 Further in vivo studies investigating the long-term effects of lavage solution temperature are needed.
Clinical Considerations for Thermal Chondroplasty
The term chondroplasty implies a surgical procedure, which promotes or facilitates the development or regeneration of normal articular cartilage. Traditionally cold cutting instruments such as drills and mechanical burrs or curettes have been used to perform these procedures. The nature of these procedures involves debriding eburnated bone to stimulate a bleeding response and allow migration of marrow cells with high potential for repair. Under appropriate biomechanical conditions, the ensuing blood clot becomes fibrous tissue, which subsequently undergoes metaplasia to fibrocartilage and, it is hoped, hyaline-like articular material. The use of thermal energy, such as RFE or laser energy, in the performing of “chondroplasty” really represents a surgical debridement of damaged articular surfaces. The primary goal is not to stimulate repair but debride unstable fragments and prevent further mechanical disruption of remaining cartilage layers. Although, some studies have suggested that repair is stimulated in lasertreated articular cartilage.23,24
The Ho:YAG laser when properly used is tangentially applied parallel to the articular surface to avoid iatrogenic damage to underlying normal chondrocytes. Grade II and III chondromalacia represent the best indications for this thermal coagulation of damaged articular surfaces. Many second-look arthroscopies have noted the sealing of articular cartilage fissures to be mechanically stable without further expansion of the degenerative areas previously treated.47
Great confusion currently exists over the indications and contraindications for RFE-assisted chondroplasty. Earlier RF chondroplasty studies were done on animal models; however, more recent in vitro human cartilage studies have allowed potential extrapolation of data to clinical situations. Despite an abundance of basic science studies, there is a lack of well-designed clinical studies, which can truly assess the benefits of RFE chondroplasty. Anecdotal reports of successful mRFE chondroplasty as assessed by second-look arthroscopies and high-field magnetic resonance imaging (MRI) have been published.47 Khan et al48 performed a retrospective study of 12 patients with grade III chondromalacia of the patella who were treated with mRFE chondroplasty. All patients had chronically symptomatic patellofemoral pain with clinical findings indicative of chondromalacia, and all patients had failed a conservative program of physical therapy and patellar taping for at least 3 months. All patients underwent preoperative MRI evaluation including T1, T2, and fast spin echo short-time inversion recovery (STIR) sequences of patellofemoral joint in sagittal, coronal, and axial planes. Special attention was paid to the evaluation of the subchondral bone for osseous edema. All patients underwent low power temperature regulated arthroscopic monopolar thermal chondroplasty of the patella. Postoperatively, three patients had the MRI evaluation at 3 months, six at 6 months, and three at 1 year. All pre- and postoperative MRIs were assessed by a board-certified radiologist. None of the 12 patients had any discernible signal changes on postoperative MRI scans of the underlying bone on T1, T2, and STIR sequences to suggest avascular necrosis. Eleven of 12 patients reported improvement in clinical symptoms at 1 year, and one patient was symptomatically unchanged compared with preoperatively.
Similar clinical successes have been presented following RFE chondroplasty.49–52 Baker et al53 noted excellent functional results along with favorable MRI findings following ablative chondroplasty. Owens et al50 reported superior clinical outcomes with bRFE debridement of patellar grade II and III chondral lesions when compared with mechanical devices. However, Stein et al,51 in a prospective study of 146 patients who were randomly placed in either a control group (chondroplasty alone) or a treatment group (chondroplasty and electrocautery), showed that electrocautery as an adjunct to chondroplasty offered little benefit in the treatment of chondromalacic lesions and in fact may limit successful outcome. Several multicenter trials are ongoing and early reports suggest RFE to be promising in the treatment of chondromalacia.52 Nevertheless the effect of RFE on articular cartilage confirms that chondrocytes are temperature sensitive, and temperatures greater than 45°C can cause excessive chondrocyte death and may promote further degeneration of the articular cartilage.28 Great care is emphasized in the selection of operative parameters regarding the RFE chondroplasty until safety and efficacy are confirmed via well-designed prospective randomized clinical studies.
Surgical Technique
Current options for thermal chondroplasty include the use of laser, bRFE (Fig. 12–2), or mRFE (Fig. 12–3). In our institution mRFE treatment of the articular surface is performed with a specially designed TAC-C probe (Oratec Interventions, Menlo Park, CA). Our selection of this probe is based on basic science studies that confirm mRFE to have the safest profile in regard to depth of chondrocyte death. Operative parameters for performing thermal chondroplasty should not exceed 50°C and 30 W of power. The current Oratec system automatically senses the probe being attached and defaults to the set parameters. In practice, the lowest possible temperature and power settings are used to achieve the desired visual thermal effect. At this time, the lavage solution is kept at room temperature but further studies are being conducted to identify the optimal lavage solution temperature for mRFE chondroplasty.
FIGURE 12–2 Bipolar Mitek Vapor probe device being used to treat the cartilage.
FIGURE 12–3 Oratec TAC C probe being used in a “near contact” or gentle contact method to treat the cartilage.
Initially, loose and fibrillated cartilage is mechanically shaved. This is done to even out the cartilage fibril length and remove larger unstable flaps allowing a more effective thermal depth of penetration and contouring. A no-contact application by bipolar probes can produce smoothing of the articular cartilage and application longer than 2 to 3 seconds is discouraged. However, a no-contact application of the monopolar probe does not produce any smoothing of the articular cartilage.30 Therefore, a “near contact” or gentle contact method is used, lightly brushing the damaged cartilage surface as it congeals and shrinks the fibrils like the end of a nylon rope. Avoid prolonged application of RFE to one place and avoid pushing the probe deep into the lesion. In vitro studies have suggested application times of up to 15 seconds for optimal contouring. However, we rely on visual assessment of smoothing and do not apply the probe for more than a few seconds to one area. At this time, the concern of causing chondrocyte death supersedes optimal contouring. In cases of grade IV lesions, the edges of the defect should be carefully smoothed to minimize step-off against areas of normal cartilage. Avoid direct application of RFE to the subchondral bone as irreversible damage may occur. The safety of this procedure lies in the surgeon’s understanding of the potential deleterious effect of temperature on chondrocyte viability and the potential of full-thickness loss of articular cartilage secondary to inappropriately high power settings. We have encountered a few cases of cartilage damage of the femoral condyles referred to us following treatment with bRFE chondroplasty. Other reports of cartilage damage following bRFE have also been published.46
Postoperatively, rehabilitation is begun with no specific restrictions other than patient comfort. Generally, this occurs a few days after surgery and emphasizes range-of-motion and progressive closed kinetic chain exercises. Although we have not had any cases of avascular necrosis of the subchondral bone following monopolar RF chondroplasty, the presence of continued pain postoperatively may warrant a workup for avascular necrosis.
Conclusion
Radiofrequency energy is currently being used in the treatment of grade II, III, and IV chondromalacia. Basic science studies have confirmed that RFE can mechanically debride the articular surface by smoothing, thus potentially reducing further delamination. However, a zone of thermal injury in the areas of treatment has been observed with loss of chondrocyte viability. bRFE appears to create a larger zone of cartilage injury than does mRFE, but other factors such as application technique, duration of application, and lavage solution temperature may also effect the viability of chondrocytes. Therefore, further use of this procedure lies in the surgeon’s understanding of the limitations of alternate treatment options and the risks and benefits of RFE chondroplasty on chondrocyte viability. At this time, we prefer mRFE, although bRFE remains a potential alternative. Great care should be taken in the selection of operative parameters by individual clinicians when using RFE chondroplasty until more clinical and basic science studies can define appropriate safety and efficacy protocols.
REFERENCES
1. Jackson RW. Arthroscopic treatment of degenerative arthritis. In: McGinty JB, Caspari RB, Jackson RW, Poehling GG, eds. Operative Arthroscopy. New York: Raven Press, 1991:319–323
2. Livesley PJ, Doherty M, Needoff M, Moulton A. Arthroscopic lavage of osteoarthritic knees. J Bone Joint Surg Br 1991;73: 922–926
3. Gibson JN, White MD, Chapman VM, Strachan RK. Arthroscopic lavage and debridement for osteoarthritis of the knee. J Bone Joint Surg Br 1992;74:534–537
4. Baumgaertner MR, Cannon WD Jr, Vittori JM, Schmidt ES, Maurer RC. Arthroscopic debridement of the arthritic knee. Clin Orthop 1990;253:197–202
5. Sprague NK III. Arthroscopic debridement for degenerative knee joint disease. Clin Orthop 1990;253:197–202
6. Hubbard MJ. Articular debridement versus washout for degeneration of the femoral condyle: a five year study. J Bone Joint Surg Br 1996;78:217–219
7. Buckwalter JA, Mankin HJ. Articular cartilage: tissue design and chondrocyte-matrix interactions. Instr Course Lect 1998;47: 477–486
8. Steadman JR, Rodkey WG, Singleton SB, Briggs KK. Microfracture technique for full-thickness chondral defects: technique and clinical results. Op Tech Orthop 1997;7:300–304
9. Grande DA, Pitman MI, Peterson L, Menche D, Klein M. The repair of experimentally produced defects in rabbit articular cartilage by autologous chondrocyte transplantation. J Orthop Res 1989;7:208–218
10. Brittenberg M, Nilsson A, Lindahl A, Ohlsson C, Peterson L. Rabbit articular cartilage defects treated with autologous cultured chondrocytes. Clin Orthop 1996;326:270–283
11. Peterson L, Minas T, Brittberg M, Nilsson A, Sjogren-Jansson E, Lindahl A. A two year to 9-year outcome after autologous chondrocyte transplantation of the knee. Clin Orthop 2000;374: 212–234
12. Hangody L, Kish G, Karpati Z, Udvarhelyi I, Szigeti I, Bely M. Mosaicplasty for the treatment of articular cartilage defects: application in clinical practice. Orthopedics 1998;21:751–756
13. Garrett JC. Osteochondral allografts for reconstruction of articular defects of the knee. Instr Course Lect 1998;47:517–522
14. Czitrom AA, Keating S, Gross AE. The viability of articular cartilage in fresh osteochondral allografts after clinical transplantation. J Bone Joint Surg Am 1990;72:574–581
15. Garrett JC. Osteochondritis dissecans. Clin Sports Med 1991;10:569–593
16. Jackson DW, Felt JC, Song Y, Van Sickle DC, Simon TM. Restoration of large femoral trochlear sulcus articular cartilage lesions using a flowable polymer: an experimental study in sheep. Trans Orthop Res Soc 2000;25:670
17. Mankin HJ. Responses of articular cartilage to mechanical injury. J Bone Joint Surg Am 1982;54:460–466
18. Goldberg VM, Caplan AI. Biologic restoration of articular surfaces. Instr Course Lect 1999;12:474–484
19. Kevin AJ, Coleman A, Wisnom MR, et al. Propagation of surface fissures in articular cartilage subjected to cyclic loading. Orthopedic Research Society 1998, New Orleans
20. Grigka J, Boenke S, Schreiner C, Lohnert J. Significance of laser treatment in arthroscopic therapy of degenerative gonarthritis. Knee Surg Sports Traumatol Arthrosc 1994;2:88–93
21. Turner AS, Tippett JW, Powers BE, Dewell RD, Hallinckrodt CH. Radiofrequency (electrosurgical) ablation of articular cartilage: a study in sheep. Arthroscopy 1998;14:585–591
22. Wirth CJ, Ruddert M. Techniques of cartilage growth enhancement: a review of the literature. Arthroscopy 1996;12:300–308
23. Collier MA, Haugland LM, Bellamy J, et al. Effects of holmium:YAG laser on equine articular cartilage and subchondral bone adjacent to traumatic lesions: a histopathological assessment. Arthroscopy 1993;9:536–545
24. Miller DV, O’Brien SJ, Arnoczky SS, Kelly A, Fealy SV, Warren RF. The use of the contact Nd:YAG laser in arthroscopic surgery: effects on articular cartilage and meniscal tissue. Arthroscopy 1989;5:245–253
25. Fink B, Schneider T, Braunstein S, et al. Holmium:YAG laser-induced aseptic bone necroses of the femoral condyle. Arthroscopy 1996;12:217–223
26. Lane JG, Amiel ME, Monosov AS, Ameil D. Matrix assessment of the articular cartilage surface after chondroplasty with the holmium:YAG laser. Am J Sports Med 1997;25:560–569
27. Trauner KB, Nikoshita NS, Flotte T, Patel D. Acute and chronic response of articular cartilage to holmium:YAG laser irradiation. Clin Orthop 1995;310:52–57
28. Lu Y, Hayashi K, Hecht P, et al. The effect of monopolar radiofrequency energy on partial-thickness defects of articular cartilage. Arthroscopy 2000;16:527–536
29. Edwards RB III, Markel MD. Radiofrequency energy treatment effects on articular cartilage Op Tech Sports Med 2001; 11: 96–104
30. Lu Y, Edwards RB III, Cole BJ, Markel MD. Thermal chondroplasty with radiofrequency energy, an in vitro comparison of bipolar and monopolar radiofrequency devices. Am J Sports Med 2001;29:42–49
31. Lu Y. MD, Edwards RB III, Kalscheur VL, Nino S, Cole BJ, Markel MD. Effect of bipolar radiofrequency energy on human articular cartilage: comparison of confocal laser microscopy and light microscopy. Arthroscopy 2001;17:117–123
32. Krenzel BA, Mann CH, Kayes AV, Speer KP. The effects of radiofrequency ablation on articular cartilage: a time dependent analysis. Presented at the 67th Annual Meeting of the American Academy of Orthopaedic Surgeons 2000, March 15–19, Orlando FL
33. Kaplan L, Uribe JW, Sasken H, Markarian G. The acute effects of radiofrequency energy in articular cartilage: an in vitro study. Arthroscopy 2000;16:2–5
34. Gundel J, Saskin H, Popovitz L, et al. The effect of bipolar radiofrequency energy on partial-thickness defects in a sheep model. Arthroscopy Association of North America 20th Annual Meeting, April 19–22, 2001, Seattle
35. Lu Y, Edwards RB, Nho S, Heiner JP, Cole BJ, Markel MD. Thermal chondroplasty with bipolar and monopolar radiofrequency energy: effect of treatment time on chondrocyte death and surface contouring. Arthroscopy 2002;18:779–788
36. Edwards RB, Lu Y, Nho S, Heiner JP, Cole BJ, Markel MD. Thermal chondroplasty of chondromalaic human cartilage. Am J Sp Med 2002;30:90–97
37. Edwards RB, Lu Y, Nho S, Rodriguez E, Markel MD. Thermometric determination of cartilage matrix temperatures during thermal chondroplasty: comparison of bipolar and monopolar radiofrequency devices. Arthroscopy 2002;18:779–788
38. Lu Y, Edwards RB, Nho S, Heiner JP, Cole BJ, Markel MD. Lavage solution temperature influences depth of chondrocyte death and surface contouring during thermal chondroplasty with temperature-controlled monopolar radiofrequency energy. Am J Sp Med 2002;30:667–673
39. Hayashi K, Markel MD. Thermal modification of joint capsule and ligamentous tissues. Op Tech in Sports Med 1998;6:120–125
40. Hecht P, Hayashi K, Cooley AJ, et al. The thermal effect of monopolar radiofrequency energy on the properties of joint capsule. An in vivo histologic study using a sheep model. Am J Sports Med 1998;26:808–814
41. Lopez MJ, Hayashi K, Fanton GS, Thabit G III, Markel MD. The effect of radiofrequency on the ultrastructure of joint capsule collagen. Arthroscopy 1998;14:495–501
42. Hecht P, Hayashi K, Lu Y, et al. Monopolar radiofrequency energy effects on joint capsule tissue: potential treatment for joint stability. An in vivo mechanical, morphological, and biochemical study using an ovine model. Am J Sports Med 1999;27:761–771
43. Lopez MJ, Hayashi K, Vanderby R, Thabit G III, Fanton GS, Markel MD. Effects of monopolar radiofrequency energy on ovine joint capsular mechanical properties. Clin Orthop Rel Res 2000;374:286–297
44. Hayashi K, Thabit G, Massa KL, et al. The effect of thermal heating on the length and histologic properties of the glenohumeral joint capsule. Am J Sports Med 1997;25:107–112
45. Naseef GS, Foster TE, Trauner K, Solhpour S, Anderson RR, Zarins B. The thermal properties of bovine joint capsule. The basic science of laser and radiofrequency induced capsular shrinkage. Am J Sports Med 1997;25:670–674
46. Hogan CJ, Diduch DR. Progressive articular cartilage loss following radiofrequency treatment of a partial-thickness lesion. A case report. Arthroscopy 2001;17:E24
47. Dillingham MF. Arthroscopic electrothermal surgery of the knee. Op Tech Sports Med 1998;6:154–156
48. Khan AM, Bonzon CJ, Indelli PF, Fanton GS. Magnetic resonance imaging of the patella following arthroscopic monopolar radiofrequency chondroplasty. Presented at the Arthroscopy Association of North America 20th Annual Meeting, April 19–22, 2001, Seattle
49. Antounian F. Nonrandomized study of coblation treatment in articular cartilage surgery: interim findings. Presented at the Arthrocare-sponsored symposium during the 67th Annual Meeting of the American Academy of Orthopaedic Surgeons, March 15–19, 2000, Orlando, FL
50. Owens BD, Stickles BJ, Balikian P, Busconi BD. Arthroscopy. Prospective analysis of radiofrequency versus mechanical debridement of isolated patellar chondral lesions. Arthroscopy 2002;18:151–155
51. Stein DT, Ricciardi CA, Viehe T. The effectiveness of the use of electrocautery with chondroplasty in treating chondromalacic lesions: A randomized prospective study. Arthroscopy 2002;18: 190–193
52. Tasto J. Radiofrequency and articular cartilage debate. Presented at the Arthroscopy Association of North America 21st Fall Course, November 17–20, 2002, Palm Desert, CA
53. Baker MD, Branche MD. Megnetic resonance imaging evaluation of ablative chondroplasty. Presented at the Arthroscopy Association of North America 21st Fall Course, November 17–20, 2002, Palm Desert, California
< div class='tao-gold-member'>
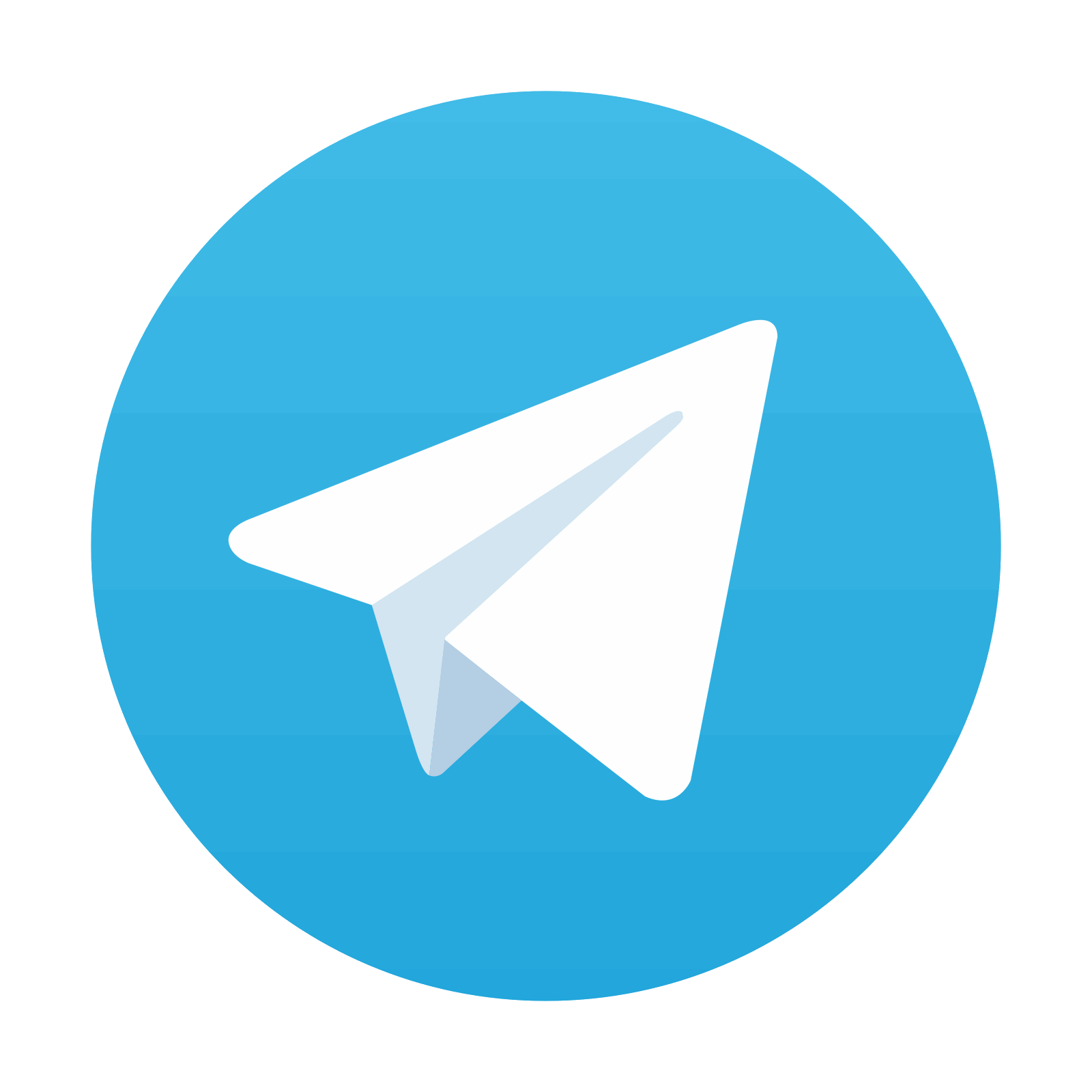