CHAPTER 17
Effects of Noninvasive Neuromodulation in Spasticity
Lumy Sawaki
Nonpharmacological, noninvasive neuromodulation is a set of techniques that can alter the excitability of the central nervous system (1–8). The increasing use of neuromodulation with humans in recent years has enabled both measurement of corticospinal excitability and reduction of motor deficits associated with neurologic impairment. To facilitate understanding of how noninvasive neuromodulation is rapidly changing the field of neurorehabilitation and neural repair with regard to spasticity, this chapter summarizes information and evidence on basic neuromodulatory techniques and how they can be useful for evaluation of the mechanisms underlying spasticity. Techniques described in this chapter include transcranial magnetic stimulation (TMS), transcranial direct current stimulation (tDCS), and a more recent technique called trans-spinal direct current stimulation (tsDCS). Although most studies of neuromodulation focus on how it may affect motor recovery in general, enough compelling evidence exists about how neuromodulation may specifically ameliorate spasticity to justify larger studies in this regard (9–12). This chapter’s review of how neuromodulation affects spasticity presents studies in which neuromodulation is applied either in singularity or in conjunction with other therapeutic interventions, such as physical therapy.
TRANSCRANIAL MAGNETIC STIMULATION
TMS uses a rapidly changing magnetic field to activate pyramidal neurons beneath a capacitor (ie, a magnetic coil) placed over the scalp, which in turn generates a secondary electric current that depolarizes the neurons (4,7,13–16). There are several types of TMS coils (Figure 17.1). The figure-8 coil is heavily used because of its focality. However, the circular coil and the double-cone coil can deliver stronger stimuli than the figure-8 coil, although the stimulation from the circular coil becomes less focal according to the strength of the magnetic field. The double-cone coil can stimulate deeper neuronal substrates than the figure-8 and circular coils. For instance, the double-cone coil has been used to stimulate the lower extremity corticomotor representation (15).
TMS depolarizes neurons either directly or through cortico-cortical connections. Thus, TMS can modify cortical excitability of substrates either directly underneath the stimulating coil or remotely via deeper structures (eg, brain circuits, interhemispheric connections) (6,15). Several TMS techniques exist, including single-pulse, paired-pulse, or repetitive TMS (rTMS). Single-pulse and paired-pulse TMS are commonly used to evaluate corticospinal excitability. These techniques can provide information about normal neurophysiology as well as pathophysiology of diverse neurologic disorders (15). In other words, single-pulse TMS and paired-pulse TMS can serve as tools for measuring neurophysiological outcomes of interest. Additionally, single-pulse or paired-pulse TMS can identify not only biomarkers of motor recovery but also potential targets for other neuromodulatory interventions, such as tDCS or rTMS (17–21). rTMS is generally considered interventional (ie, applied as a therapeutic treatment). It uses trains of stimulation and can inhibit or excite corticospinal tracts with the effects outlasting the period of stimulation (4,15,22).
TMS measurements can vary according to the accuracy and consistency of coil positioning (23,24). To decrease this variability, several stereotaxic neuronavigational systems have been developed (Figure 17.2). These systems facilitate precise identification of the coil position in relation to an individual’s characteristics, such as shape of the head. Compared with the traditional coil positioning protocol (ie, according to the 10–20 EEG system), stereotaxic neuronavigation assists in achieving better consistency of coil angle and other positioning aspects during either a single TMS session or longitudinally (25–27). Drawbacks of neuronavigational systems include high costs of equipment and the need to have highly trained personnel.
FIGURE 17.1 Transcranial magnetic stimulation (TMS) coils. The figure-8 coil (A) delivers a magnetic pulse focused at the meeting point between the two circles, as denoted by the arrow. The double-cone coil (B) is capable of reaching deep structures in the brain, such as the motor representation of the lower extremities. This coil also delivers a magnetic pulse as denoted by the arrow.
FIGURE 17.2 Transcranial magnetic stimulation (TMS). TMS coils are placed on the scalp above the areas to be stimulated. Proper coil positioning is made easier by using a neuronavigational system. In this setup, reflective balls are attached to the TMS coil and to a headband worn by the subject. These reflective balls are tracked by an infrared camera (not shown) and read by the neuronavigational software. The software projects the coil location onto an MRI of the subject’s brain (shown on the center computer screen), which allows the study personnel to target specific areas of the cortex. When using TMS to stimulate the motor cortex, EMG is monitored in order to measure the motor evoked potentials (MEPs) that occur in response to the TMS (far-right computer monitor). The spot on the cortex that elicits the largest MEP when stimulated is considered the “hot-spot” and is marked using the software, which makes it easier to return to the spot in the same session and in future sessions.
Single-Pulse TMS
Single-pulse TMS is considered very safe and well tolerated (15). There is no known evidence of long-term adverse effects. Several single-pulse TMS techniques have been applied to investigate corticospinal motor pathways in humans (13,28). The following list delineates aspects of neural function that single-pulse TMS can measure:
• Motor-evoked potentials (MEPs) are responses to TMS applied in the primary motor cortex. MEPs are recorded by surface electromyography (EMG) of the target muscles. Usually, peak-to-peak amplitude and/or latency are measured (29–31).
• Resting motor threshold is a measure of neuronal membrane excitability. It is defined as the minimum stimulation intensity that can elicit at least 5 of 10 MEPs (usually of 50 µV) at rest. It has been shown to be reproducible across sessions and thus is extensively used in longitudinal studies (32,33).
• Cortical motor mapping provides information about location and strength of the cortical representation of a given muscle. Increase in the spatial volume of the cortical map has been associated with neuroplasticity (1,34–38).
• Recruitment curve is another measure of cortical excitability and measures the relationship between TMS stimulus intensity and motor output (eg, MEP). An increase in the slope of the recruitment curve is one of the indications that neuroplastic change has occurred (13,39–41).
• Cortical silent period is the transient suppression of EMG activity during pre-activation in response to TMS. The duration of the cortical silent period is an indirect measure of cortical excitability (1,42–48).
There are several studies that illustrate the feasibility of using single-pulse TMS to evaluate neural function and related aspects in subjects with neurologic conditions:
1. Sartucci et al used single-pulse TMS to evaluate resting motor threshold, amplitude of MEPs, and cortical silent period in 12 genetically homogeneous individuals diagnosed with autosomal dominant hereditary spastic paraparesis. They recorded MEPs from upper and lower extremities and compared results to data from healthy volunteers. For the group with paraparesis, resting motor threshold was significantly higher, and the MEP amplitude and cortical silent period were comparatively decreased, but only in the lower extremities. These neurophysiological findings correlated with results from the Modified Ashworth Scale, which is a tool that enables subjective measurement of clinical spasticity. The investigators concluded that (a) single-pulse TMS can be a useful tool to characterize hereditary spastic paraparesis and (b) that this disorder is associated with selective abnormalities in mechanisms of excitability at supraspinal and segmental levels (49).
2. Cruz Martinez et al applied TMS to evaluate upper extremity spasticity in subjects with stroke. They reported that the cortical silent period was significantly decreased in the paretic upper extremity compared to the nonparetic upper extremity. The degree of cortical silent period decrease in the paretic upper extremity was correlated with results on the Modified Ashworth Scale. Investigators also found significant differences in comparing these subjects’ cortical silent period to those of a control group (50). Similarly, Uozumi et al found a decreased cortical silent period in 17 subjects with stroke-related spasticity compared to healthy controls (51).
3. Kaufmann evaluated whether TMS and magnetic resonance (MR) spectroscopy can be used to identify objective markers of upper motor neuron involvement in amyotrophic lateral sclerosis. Results showed that although MR spectroscopy might have higher sensitivity compared with TMS, both techniques in conjunction had higher sensitivity than either technique alone in diagnosing early stages of the disease (11).
4. Single-pulse TMS to measure presence or absence of MEPs, to determine motor threshold, or to serve as a prognostic tool correlating with clinical findings (ie, spasticity) has not proved useful in cases of spinal cord injury (52). This limitation may be due partially to the complexity of spinal cord injury. For example, spinal cord trauma has potential association with pathological changes in upper motoneurons, lower motoneurons, roots, and/or spinal nerves. On the other hand, extensive neuroplastic change can occur as a matter of natural course after spinal cord injury (eg, axonal and peripheral sprouting; synaptogenesis; unmasking; corticospinal reorganization). This natural course often plays a role in recovery of function within the first year after injury.
The aforementioned studies yield several notable insights about single-pulse TMS. The first three studies demonstrate that in certain neurologic populations, when there is a clinical presentation of spasticity, resting motor threshold appears to be increased. That is, a higher intensity of TMS is needed to elicit the same amount of MEPs that are elicited in normal healthy volunteers (ie, people without neurologic impairment) at a lower TMS intensity. Thus, single-pulse TMS can serve as a tool for objectively measuring spasticity. A caveat for this interpretation is that increased resting motor threshold in the subjects for these studies could be related to their baseline neurologic condition (ie, not specific to spasticity). On the other hand, these subjects’ decreased cortical silent period compared to normal healthy volunteers may be more specific to spasticity. In sum, it appears there is a good correlation of TMS measurements with clinically measured spasticity. However, this principle appears less applicable to spinal cord injury due to the more complex trajectory of recovery associated with this condition.
Paired-Pulse TMS
Paired-pulse TMS facilitates evaluation of intracortical connections of excitatory and inhibitory mechanisms of pyramidal tract neurons (1,28,53–57). In paired-pulse TMS, two pulses are delivered contiguously, at a range of different intervals and intensities. The first stimulus is considered a conditioning pulse and the second pulse is considered the test pulse. Paired-pulse TMS can be delivered via one coil to a single location or via two coils that target two different regions. It is generally accepted that when the interstimulus interval is between 1 ms and 4 ms, intracortical inhibition will occur, and when the interstimulus interval is between 7 ms and 20 ms, intracortical facilitation will occur. Studies applying pharmacological interventions suggest that gamma-aminobutyric (GABA)-ergic mechanisms mediate the effects of paired-pulse TMS (10,58,59).
There are several studies that illustrate the feasibility of using paired-pulse TMS to evaluate neural function and related aspects in subjects with neurologic conditions:
1. Marconi et al compared physical therapy with an intervention consisting of muscle vibration combined with physical therapy for the upper extremities in 30 subjects with chronic stroke (60). Pre-/postmeasurement included single-pulse TMS techniques to collect data on resting motor threshold and cortical motor map as well as paired-pulse TMS techniques to measure intracortical inhibition and facilitation. Clinical outcomes of interest included motor function and spasticity. Follow-up evaluations occurred 2 weeks after the intervention. Results immediately after intervention as well as at 2-week follow-up showed decreased resting motor threshold and increased motor map for only the group that received vibration and physical therapy. Additionally, paired-pulse TMS showed increased intracortical inhibition of the extensor digitorum communis muscle and decrease of intracortical inhibition of the flexor carpis radialis muscle that was significantly correlated with reduction of spasticity and increased motor function for the group that received vibration and physical therapy.
2. Nielsen et al applied paired-pulse TMS to investigate the relationship between corticomotor circuitry and spasticity in 12 subjects with autosomal dominant pure spastic paraplegia linked to chromosome 2p (10). Results showed greatly increased intracortical facilitation compared with a control group. The authors speculated that antiseizure medications with gamma-aminobutyric (GABA) ergic or glutamate antagonist effects in the corticomotor area might decrease exacerbated intracortical facilitation and therefore mitigate spasticity in this condition.
3. In Iwata’s case study of a patient with stiff-leg syndrome, paired-pulse TMS revealed that intracortical facilitation was exacerbated by a degree more than two standard deviations greater than that of healthy controls (61). This excessive response to paired-pulse TMS was alleviated with oral diazepam and associated with amelioration of the spasticity.
In sum, paired-pulse TMS may be a useful tool to screen the effectiveness of different interventions (eg, drugs) to modulate spasticity. This inference arises from the aforementioned studies in which paired-pulse TMS revealed that there is an increase in intracortical facilitation and a decrease of intracortical inhibition associated with spasticity. In all of the aforementioned studies, paired-pulse TMS was used solely to understand neuropathology or to measure intervention-related change, such as change arising from occupational therapy or from a pharmaceutical agent. The interventions in these studies were associated with trends toward normalizing spasticity.
Repetitive TMS
There are two basic methods for delivering rTMS. The standard methodology is to deliver it with a constant frequency. An alternate method is to deliver it in a patterned fashion of stimulation (the most prevalent example is theta burst rTMS, also known as TBS). rTMS alters corticospinal excitability, depending on the type and frequency of the pulses. Standard rTMS can be subclassified as low frequency (1 Hz) or high frequency (equal to or higher than 5 Hz) (4,15,62). In general, low-frequency rTMS of this type has inhibitory effects, whereas high-frequency rTMS has excitatory effects. TBS (ie, the alternate form of rTMS) can be classified as intermittent (iTBS, excitatory) or continuous (cTBS, inhibitory). Several new protocols to deliver rTMS have been developed in recent years. Among these, the quadripulse stimulation is a type of patterned rTMS that appears to have longer modulatory effects than other rTMS protocols (63,64). However, no studies to date have evaluated its effects on spasticity.
Mechanisms by which standard or patterned rTMS can modify corticospinal excitability are complex and vary according to subjects’ intrinsic oscillatory rhythms and medications, the position of the TMS coil, and waveform, intensity, duration, or frequency of the trains (15). Animal and human studies have suggested that rTMS can modulate corticospinal excitability via neurotransmitters including dopamine and GABA (15). Moreover, evidence indicates that rTMS can modulate corticospinal tracts via mechanisms resembling long-term potentiation and long-term depression.
rTMS has shown therapeutic promise in several areas, including motor deficit in neurologic conditions, depression, anxiety, obsessive–compulsive disorder, chronic pain, addiction, and tinnitus (4,15,65–69). rTMS has received approval by the Food and Drug Administration (FDA) only for the treatment of patients with depression who have not responded to pharmacological interventions (70,71). The constraints on FDA approval owe mainly to the lack of large, multicenter randomized trials investigating the efficacy of rTMS for other neurologic conditions.
Rossi et al provide updated, in-depth safety guidelines and recommendations for standard and patterned rTMS protocols (15). Although adverse events for rTMS are rare, there are reports of undesired effects when rTMS deviated from these recommendations. The most serious adverse event related to rTMS is seizure. A very few occurrences of seizure have been reported when rTMS was applied outside the guidelines for exclusion criteria or in subjects with a history of seizure or who take medications that lower the threshold for seizure. Other side effects include headache, neck pain, toothache, transient hearing loss, or short-lasting alteration of working memory.
There are several studies that illustrate the feasibility of using rTMS to modulate cortico-cortical or corticospinal excitability:
1. Kumru et al applied 20 Hz patterned rTMS (40 pulses per train) for a total of 1,600 stimuli more than 20 minutes over the lower extremity cortical representation for 5 days in subjects with incomplete spinal cord injury. Investigators aimed to upmodulate the excitability of the primary motor cortex and therefore increase corticospinal inhibitory input and decrease segmental spinal hyperexcitability. Results indicated decreased spasticity measured by the Modified Ashworth Scale and the Modified Penn Spasm Frequency Scale. Improvements remained evident at the 1-week follow-up evaluation (72). Investigators then applied these parameters of rTMS for 15 days in subjects with incomplete spinal cord injury and measured effects on spasticity (Modified Ashworth Scale), gait function, and H-reflex (a reliable neurophysiological index of spinal excitability) (73). Although the intervention decreased spasticity and improved gait function, no H-reflex modification was evident.
2. Valle et al applied 5 consecutive days of standard rTMS (5 Hz, 1 Hz, and sham, in a randomized order) over the ipsilesional hemisphere in 17 children with cerebral palsy. The total number of pulses in 5-Hz and 1-Hz condition received identical number of pulses of 1,500 stimuli. Investigators hypothesized that increasing corticomotor excitability with excitatory standard rTMS would downmodulate the exacerbated spinal excitability that is associated with cerebral palsy. The 5-Hz condition led to increased range of motion and spasticity. There were no changes after 1-Hz rTMS or sham (74).
3. Kakuda et al evaluated how rTMS combined with occupational therapy affects severe poststroke spasticity (defined as Brunstromm stage 3–5). Investigators delivered standard 1-Hz rTMS over the contralesional hemisphere for 15 days in 39 subjects with chronic stroke (75). Each subject received a total of 1,200 stimuli. The investigators’ intent in applying inhibitory 1-Hz rTMS to the contralesional hemisphere was to decrease its exacerbated transcallosal inhibitory effect on the ipsilesional hemisphere, which would consequently increase the cortical influence of the ipsilesional hemisphere on the spinal cord. Outcome measures included Modified Ashworth Scale, Fugl-Meyer Assessment Scale (an assessment of motor function), and Wolf Motor Function Test. Improvements in spasticity and motor function after the intervention persisted to the 1-month follow-up. Similar findings resulted from this author’s separate study of low-frequency rTMS combined with levodopa and occupational therapy for 2 weeks (76).
4. Mally and Dinya applied 1-Hz rTMS twice daily (100 stimuli per session) for 7 days in 64 subjects with severe spasticity nearly 10 years poststroke (77). Investigators delivered rTMS over the contralesional and/or ipsilesional hemisphere according to the induction of paretic arm movements by single-pulse TMS. Thus, there were four groups: (a) movements induced via single-pulse TMS to bilateral hemispheres, followed by bilateral rTMS; (b) no movements induced via single-pulse TMS to either hemisphere, followed by contralesional rTMS; (c) movements elicited via single-pulse TMS to the ipsilesional hemisphere, followed by ipsilesional rTMS; and (d) movements elicited via single-pulse TMS to the contralesional hemisphere, followed by contralesional rTMS. Outcome measures included subjective measures of spasticity and motor function. For all groups, the intervention led to decreased spasticity that persisted at 1- and 2-month follow-up evaluations. The authors inferred that with improvement in both motor function and spasticity, the group that received contralesional rTMS improved the most. Although this study applied a very small number of rTMS pulses (ie, 200 per day), placement of inhibitory rTMS on the contralesional hemisphere appeared to lead to a decrease in transcallosal inhibition and thus improvement in function including spasticity.
5. Theilig et al combined 2 weeks of surface neuromuscular stimulation of the hand and finger extensors with either active or sham 1-Hz rTMS for 15 minutes over the contralesional hemisphere in 24 subjects with stroke (2 weeks to 58 months poststroke) (78). Outcomes of interest included spasticity (Tardieu Scale) and motor function (Wolf Motor Function Test). Although improvements in spasticity and motor function were present for both groups after the intervention period, no significant differences were found between the two groups. No long-term follow-up occurred in this study.
6. Conversely, Etoh et al conducted a randomized, double-blind, crossover study to evaluate the effects of inhibitory 1-Hz rTMS (total of 240 pulses) in 18 subjects with chronic stroke and Brunstromm stage greater than 3 (79). They delivered rTMS over a 2-week period over the contralesional hemisphere and measured F-waves, M-waves, Fugl-Meyer Assessment Scale, Action Research Arm Test, and the Simple Test for Evaluating Hand Function (measures the ability of the subject to manipulate objects). Evidence indicated improvement in motor function, but no changes in spasticity or neurophysiological measures of the hemiparetic extremity.
7. Wupuer et al applied a single session of high-frequency 10-Hz rTMS (1,000 stimuli; 50 pulses per train; 25-s inter-train interval) over the ipsilesional hemisphere of subjects with chronic stroke (n = 12) as well as subjects without neurologic impairment (ie, healthy volunteers; n = 14) (80). Outcomes included changes in F wave and F/M ratio. The F wave is a measure of spinal excitability in which the amplitude and frequency are generally increased when there is excessive excitability of spinal motoneuronal pools. As the investigators expected, they found exacerbated F wave and F/M ratio in the stroke group compared to the healthy volunteers group. The rTMS intervention led to a significant decrease in the persistence of F-waves as well as decrease in the ratio of F-waves and M-waves in the stroke group. This study suggests that upmodulating the ipsilesional motor cortex increases the cortical influence on exacerbated spinal excitability and has potential to decrease spasticity.
8. Centonze et al applied 5-Hz and 1-Hz rTMS (total number of pulses was 900 for each condition) in 19 subjects with multiple sclerosis. Outcomes of interest included changes in H-reflex, M-wave, and spasticity (Modified Ashworth Scale) (81). Initial results demonstrated that a single session of 5 Hz decreased the ratio of H-reflex/M-wave and that 1 Hz increased this ratio, but there was no change in spasticity. However, 2 weeks of daily 5-Hz rTMS over the lower extremity cortical area ameliorated spasticity to a degree that persisted at least to 1-week follow-up. This study suggests that upmodulating cortical influence to decrease exacerbated spinal excitability may be a potential avenue to normalize neurophysiological measures of H/M ratio and that repeated rTMS sessions might be required to yield clinically notable changes in spasticity.
9. In a sham-controlled study, Mori et al evaluated the effects of iTBS daily for 2 weeks in 20 subjects with multiple sclerosis (82). Before and after the stimulation protocols, blinded raters evaluated spasticity (Modified Ashworth Scale) and the H/M amplitude ratio of the soleus H-reflex. Subjects receiving active (not sham) rTMS demonstrated significant reduction of H/M amplitude ratio and spasticity. These improvements lasted for at least 2 weeks after the completion of the intervention period.
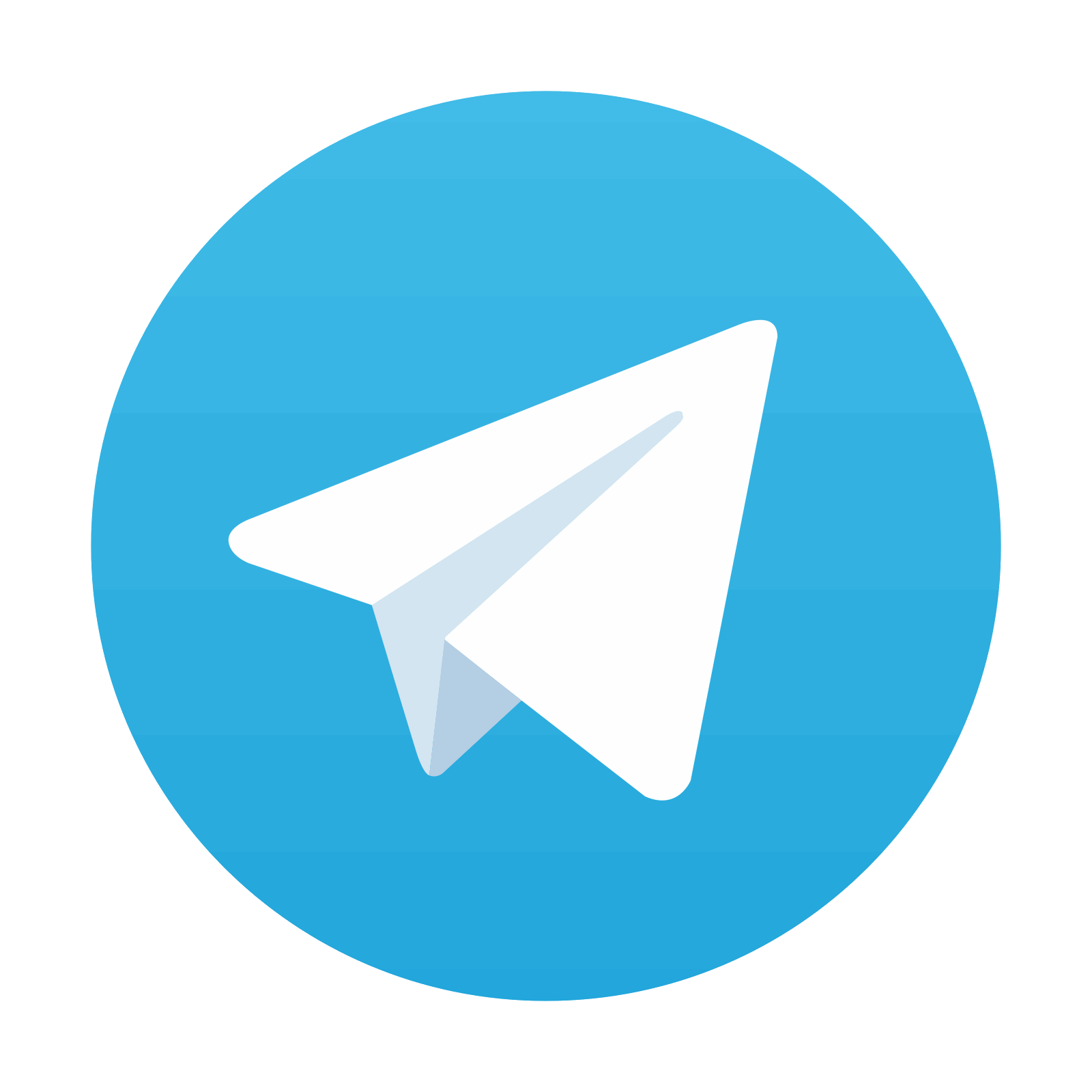
Stay updated, free articles. Join our Telegram channel

Full access? Get Clinical Tree
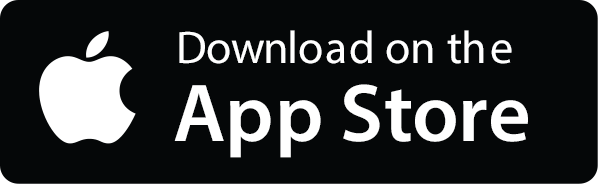
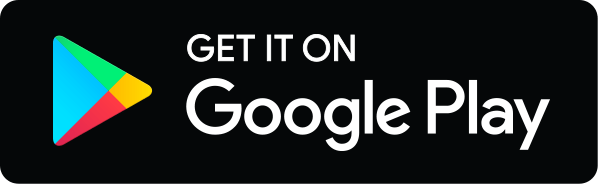