Effects of aging on vascular function
Kristin Von Nieda
Introduction
The study of aging has grown significantly in recent years. Several factors, such as increase in the aging population, the increase in life expectancy and the increase in health expenditure, contribute to growth. Studies concerned with aging have also evolved in scope. Earlier studies focused on identifying a single cause or explanation for aging. More recently, aging has been viewed as a complex process in which many factors and processes interrelate. Thus, a single cause or process is no longer sufficient to address the intricacies of the vascular aging process (Weinert & Timiras, 2003; Kovacic et al., 2011a).
Age-associated changes occur in the musculoskeletal, neuromuscular, cardiovascular, pulmonary and integumentary systems. This chapter addresses a subset of the cardiovascular and pulmonary systems and focuses on the effects of aging on the vascular system. Just as these systems function interdependently, it is impossible to isolate and limit aging effects to the vascular system without having an awareness of concomitant age-associated changes in other systems.
Review of the structure and functions of the vascular system
The oxygen transport system is the biological system responsible for (i) bringing oxygen into the body from the ambient environment; (ii) circulating oxygen throughout the body; (iii) supplying oxygen at the tissue level; and (iv) ultimately removing the waste products created as a result of utilizing oxygen. The vascular system is the means by which oxygen and nutrients are delivered to the working tissues, and by which the metabolic byproducts are removed from the tissues. The vascular network supplies a steady stream of oxygen-rich blood that allows working tissues and muscles to function at optimal levels. The ability to shunt blood preferentially and to deliver oxygen to the areas of greatest metabolic demand makes the vascular system an essential component of the oxygen transport system.
The vascular system is made up of three basic types of blood vessel: the arteries, capillaries and veins. The thickness of each layer of the vessels varies throughout the vascular system depending on the location and function of the specific vessel (see Fig. 9.1).
The arterial system functions to accommodate the large volume of blood received as cardiac output and to propel it forward using the property of elastic recoil. The presence of smooth muscle in the arterial system allows it to control and direct the flow of blood throughout the vascular system via autonomic and endothelial controls and in response to local metabolic demand.
The normal structure of the arteries includes three layers. The adventitia is the outermost layer and attaches the vessel to the surrounding tissue. It consists of longitudinally oriented connective tissue with varying amounts of elastic and collagenous fibers. The middle layer or the media is usually the thickest and is a highly elastic, circumferentially oriented fibromuscular layer. Its function is to provide vascular support and to regulate blood flow and blood pressure by facilitating changes in diameter. The intima, the innermost layer, is composed of a single, continuous layer of endothelial cells that separates blood from the vessel wall. The endothelium serves as a barrier between the circulating blood and the underlying interstitium and cells, allowing selective transport on macromolecules in the blood to meet metabolic demands in surrounding tissues. The endothelium responds to regulatory substances released by physical and chemical stimuli and has many important functions, including regulation of vascular tone and growth, thrombosis and thrombolysis, and interaction with platelets and leukocytes.
The capillaries are the smallest and most numerous of the blood vessels, forming the connection between the arteries and the veins. The capillaries are thin and fragile in comparison to arteries and veins, and there is little resistance to the diffusion of oxygen and other metabolic products. The capillary wall is one endothelial cell thick, which allows for the exchange of nutrients and waste products at the tissue level.
The veins have the same three layers as the arteries; however, the walls are thinner and less rigid because there is less smooth muscle and connective tissue. The veins are capacitance vessels, which serve as collecting tubules for blood as it exits the capillary beds. At any given time, the majority of the blood volume is located in the venous circulation. The maintenance of a larger reservoir of venous blood allows for adequate venous return as well as a necessary reserve during periods of increased oxygen demand.
Venous return is the principle determinant of cardiac preload, and sufficient venous return is necessary to ensure sufficient cardiac output. This function is accomplished by a combination of venous smooth muscle contraction, external muscle compression and a series of unidirectional internal venous valves.
Age-Associated vascular changes
Advancing age and associated vascular changes are recognized as a major risk factor for cardiac disease. Aging in the presence of cardiovascular disease accelerates structural and physiological changes, and the presence of other risk factors further influences the rate at which the changes occur. Hence, physiological aging and chronological aging cannot be considered equivalent.
In addition to the alteration of the underlying cardiovascular structures and functions, the increase in life expectancy also lengthens the exposure time to certain risk factors. In this sense, age-associated cardiovascular changes in structure and function are ‘partners’ with cardiovascular disease mechanisms. More specifically, it is the interaction between age, disease and several additional factors, such as lipid levels, diabetes, sedentary lifestyle and genetics, that determines the threshold, severity and prognosis of the disease in older people (Lakatta & Levy, 2003). The presence of risk factors such as abdominal obesity, which is associated with metabolic risk factors, such as insulin resistance, metabolic syndrome and impaired glucose tolerance, compounds the effects of aging on the vascular system (Scuteri et al., 2005).
Age-related cardiovascular changes occur in healthy, unhealthy and seemingly healthy older people. Lakatta and Levy (2003) differentiated between ‘successful’ and ‘unsuccessful’ aging. ‘Successful’ aging refers to healthy individuals, for whom the age-associated changes pose little or no threat to the development of disease. ‘Unsuccessful’ aging encompasses individuals who do not have or have not yet experienced clinical cardiovascular disease, but whose age-related cardiovascular changes put them at risk for future disease.
Structural changes associated with aging
Structural changes associated with aging occur throughout the vascular system. Significant changes occur within the walls of the large elastic arteries, in which the intimal medial thickness increases two- to three-fold between the ages of 20 and 90 years (Nagai et al., 1998). The adventitia is most affected by a decrease in the number of elastic fibers and an increase in collagen, resulting in a loss of distensibility and a reduction in elastic recoil, essential for accommodating blood volume and propelling the blood into the vascular system. Both within the intima and the media, there is increased calcification, loss of elastin content, fragmentation of the elastin and an increase in collagen. These changes lead to increased systolic blood pressure, decreased diastolic blood pressure and widened pulse pressure, which in turn may affect ventricular hypertrophy, renal impairments and adverse cerebrovascular events (Kovacic et al., 2011b). Lipid deposits in the intima further contribute to vascular wall thickening. These structural changes appear to be similar to the atherosclerotic changes seen in disease states, but occur even in the absence of occult disease and within populations with a low incidence of atherosclerosis (Moore et al., 2003).
Recently, telomere shortening was implicated in vascular aging and cardiovascular disease (Kovacic et al., 2011a). A telomere is a region of repetitive nucleotide sequences at each end of a chromosome, which makes it possible for cells to divide and protects the ends of the chromosome from deteriorating. Telomeres shorten with each cell division, and cells are generally unaffected until the telomeres become too short. When this occurs, cellular division ceases and cellular senescence or cell death ensues. Kovacic et al. (2011) reported on several studies linking increased incidence of coronary artery disease or atherosclerotic disease and shortened telomere length in peripheral blood leukocytes. Further, leukocyte telomere length was found to decrease by 6–9% per decade, and there was a predictive value for cardiovascular events associated with shortened telomeres. To some extent physical activity has a positive effect on the maintenance of telomere length (Werner et al., 2009).
Aging is linked to significant changes in the microcirculation, resulting in age-associated endothelial dysfunction. The endothelial cells become irregularly shaped and are no longer longitudinally oriented along the vessels. Endothelial permeability is increased (Ferrari et al., 2003), disrupting the selective transport system and resulting in concentrations of macromolecular materials and proinflammatory substances that further contribute to plaque formation or atherosclerosis. Within the media, vascular smooth muscle cells proliferate, migrate and infiltrate into the subendothelial space (Lakatta & Levy, 2003). The irregular alignment and the increase in intimal medial thickness affect the dynamics of and resistance to blood flow, thereby affecting the transport of oxygen and other nutrients.
Lakatta and Levy (2003) also reported that arterial stiffness may be influenced by endothelial regulation of vascular smooth muscle tone. The age-associated changes in endothelial function further contribute to vascular stiffness in both the large and peripheral arteries, thereby hindering the normal contractile capability of vascular smooth muscle.
The age-associated increase in vascular wall thickness is accompanied by dilatation of the large arteries, loss of compliance and an increase in arterial stiffness, which may not be uniform throughout the vascular system (D’Alessio, 2004; Ungvari et al., 2010). In peripheral vessels there is less of an increase in diameter of the vessels and more of an increase in wall thickening. In large arteries there is an age-dependent loss of capacitive compliance, whereas the reduction in small artery compliance is oscillatory or reflective (McVeigh et al., 1999). Both types of compliance changes contribute to modifications in the generation, propulsion and reflection of pulse waves in the aging vascular system.
Pulse wave velocity (PWV), a noninvasive measure of vascular stiffness, increases with age in populations with little or no atherosclerosis, indicating that the increase in stiffness can develop independent of atherosclerotic changes (Lakatta & Levy, 2003; Strait & Lakatta, 2012). The increase in PWV is associated with changes in vascular structure, most notably the increased collagen, decreased elastin, increased elastin fragments and calcification in the media. When a forward pulse wave reaches an area of mismatch in the vascular structure, the wave is reflected back through the artery to the central aorta. Whereas in young subjects the reflected wave arrives at the proximal aorta in diastole, the reflected wave in older vessels travels faster and arrives during late systole, causing an increased load for the ventricle and a potential adverse affect on coronary blood flow (Strait & Lakatta, 2012).
Age-associated changes also affect the venous vessels. There is an overall increase in stiffness and a decrease in venous compliance Hernandez and Frank (2004). The venous valves begin to lose their integrity and the efficiency of unidirectional flow is lessened. It becomes more difficult to maintain venous return, and there exists the potential for venous stasis and retrograde flow. In a study of cross-sectional area (CSA) of the femoral and long saphenous veins, CSA was found to be associated with body mass index, gender and the presence of varicose veins but not necessarily with age (Kroeger et al., 2003).
Varicose veins are more commonly found in the lower extremities and are characterized by tortuous dilatation and changes in the smooth muscle composition and extracellular matrix in the vessel walls, resulting in the venous stasis and venous back flow (Jacob, 2003). Peripheral edema formation is common. The incidence of varicose veins increases with age and is also affected by body mass index, prior or family history, and the presence of the disease during pregnancy. The estimated incidence of varicose veins in women increases from 41% to 73% between the fifth and seventh decades. For the same time span, the incidence for men increases from 24% to 73% (Statistics from Varicose Veins, 2006). Although recognized as the most common vascular disease, the presence of varicose veins is not clearly linked to disease development. Results from the Normative Aging Study population, taken over more than 35 years of follow-up, showed that men with varicose veins were less likely to develop symptomatic congestive heart failure than men without varicose veins (Scott et al., 2004).
Physiological changes associated with aging
The age-associated structural alterations in blood vessels are further influenced by physiological changes that have a significant impact on the cardiovascular system. It is difficult to elucidate the intricacies of all of the interrelationships, and this section addresses only some of the interactions between the systems.
Systolic blood pressure (SBP) is known to increase with age. Blood pressure analysis of 2036 subjects over a period of 30 years in the Framingham Heart Study indicated age-related increases in SBP, pulse pressure (PP) and mean arterial pressure (MAP), with an early rise (until 50 years of age) and a late fall (after 60 years of age) in diastolic blood pressure (DBP) (Franklin et al., 1997). The increase in MAP is attributed to the progressive increase in vascular resistance associated with aging, but vascular change in PP is a function of left ventricular ejection, large artery stiffness, early pulse wave reflection and heart rate. The rise in SBP is a result of both the increase in vascular resistance and increased stiffness in the large arteries. Under normal conditions and when arterial tone and PWV are normal (before the influence of age-associated changes on the vascular system), the reflected pulse wave reaches the heart after the aortic valve closes, thereby enhancing DBP. With the age-associated increases in arterial stiffness and PWV, the reflected pulse wave reaches the heart before the aortic valve closes, resulting in an increase in SBP and the loss of the diastolic pressure enhancement. The late fall in DBP is associated with large artery stiffness. Franklin et al. (1997) concluded that large artery stiffness rather than vascular resistance becomes the predominant factor for blood pressure changes as aging progresses. As the arterial walls become stiffer, they also become less distensible, and the lumen diameter of large central arteries increases to help accommodate blood volume as it is ejected from the left ventricle.
The loss of the elastic recoil together with the increased stiffness precipitates a decrease in the ability of the vessel to compress and propel the blood forward through the vascular system. A higher PP must then be generated to move a given volume of blood through a vessel. Because the heart is the pump that generates the initial propelling force, a decrease in the compliance of the arterial system results in an increase in the workload being placed on the heart.
At the level of the arterioles, capillaries and endothelium, alterations in structure result in alterations in function. Endothelial dysfunction has an enormous impact on the vascular system because the actions of endothelial cells are complex and involve several systems. With aging, the integrity of the endothelium is damaged and there is decreased activity of endothelium-derived relaxed factors (EDRF) including nitric oxide (NO), bradykinin and hyperpolarizing factor. Release of EDRF normally results in vasodilatation and serves to counter the actions of endothelium-derived constricting factors (EDCF) (e.g. endothelin, angiotensin II), both on vascular tone and on the stimulation of growth factors derived from endothelial cells. With the decrease in EDRF activity, the vessels remain more narrowed, thus contributing to the increase in resistance to flow and the increase in PP and SBP. NO is essential for endothelial health and factors in the control of vascular tone, inhibition of platelet function and reduction in the proliferation of the intima. Oxidative stress is associated with aging and results in inactivation or reduction of NO synthesis. The age-related alteration in NO activity results in an increase in the growth and proliferation of these cells, which accumulate and add to vascular wall thickening and platelet formation (Taddei et al., 2001). The effect on areas of the vascular system include severe impairment of blood flow and stress-induced vasodilatation, which limit necessary minute adjustments in blood supply in response to demand (Ungvari et al., 2010).
Another important function of the endothelium that changes with aging is the mediation of proinflammatory and anti-inflammatory responses through a complex series of reactions to changes in EDRF and EDCF activity, growth factors, adhesion molecules, monocytes, cytokines, lipids and enzymes. Proinflammatory substances are no longer adequately inhibited, resulting in local inflammation, plaque formation, thrombosis and plaque rupture. With endothelial dysfunction there is an increase in plasma C-reactive protein, which is both a mediator and a marker of inflammation.
A variable pattern in the distribution of blood flow at rest is noted. Much of this decrease may be attributed to the diminished ability of the smaller arteries and arterioles to vasodilate. The net change toward vasoconstriction in these vessels also increases the turbulence of the blood flow. The endothelium responds to mechanical forces, such as the shear force of turbulent blood flow, promoting inflammation and its related sequelae. The shear forces from normal laminar flow act in a protective manner against atherogenesis. Turbulent flow is significantly more resistive than laminar flow, and the work required by the cardiovascular system to overcome the increased resistance intensifies.
Age-associated hormonal changes play a role in the development of vascular changes. Vascular aging differs in men and women. In men, circulating testosterone levels decrease with age. Low testosterone is associated with arterial stiffness and is recognized as a cardiovascular risk factor (Hougaku et al., 2006). Circulating testosterone aids in the synthesis and bioavailability of NO essential for vascular health. Endothelial dysfunction occurs earlier in men than in women, because the decline in testosterone levels begins in early adulthood (Lopes et al., 2012). Lopes et al. reported after a thorough review of studies related to testosterone and aging, that age-associated decline of testosterone levels is associated with age-related metabolic and cardiovascular diseases, insulin resistance and atherosclerosis. Estrogen appears to have a protective effect on the endothelium, and there is an abrupt and sharp decline in endothelial function after the onset of the menopause (Taddei et al., 1996). Menopause and the associated decline in natural estrogen often occurs at a time when age-related vascular changes occur, making it difficult to determine age-related changes from lack of estrogen (Novella et al., 2012).
Given the structural and functional changes in aging blood vessels, it is not surprising to recognize aging as a major risk factor for cardiovascular disease.
Autonomic system changes with aging
Autonomic control declines with age, primarily reflecting an enhancement of sympathetic nervous system activity and a suppression of parasympathetic nervous system activity (Harris & Matthews, 2004). Because of changes in the interplay between the autonomic nervous system and the cardiovascular system, it becomes more difficult to maintain hemodynamic stability. There is a decrease in beta-adrenoreceptor responsiveness in the vasculature. The vascular responses of alpha-1-adrenoreceptors are either unaltered or may be increased with age (Priebe, 2000). The loss of responsiveness to beta-adrenergic stimuli with or without an increase in alpha-1-adrenoreceptor stimulation results in a predominance of alpha-1-adrenergic-mediated responses. Without sufficient vasodilatory input from beta-adrenoreceptors, the autonomically mediated vasoconstriction compounds the vasoconstriction resulting from the previously described mechanisms.
A significant decrease in the reactivity of cardiopulmonary reflexes, especially the reflex mediated by the baroreceptors, occurs with advancing age. Baroreceptor activity is directed by the stretch demand of vascular walls in the aorta and the carotid arteries by the blood flowing through the vessels. A decrease in the required stretch, and thus in baroreceptor activity, normally results in signals ordering restoration of cardiac output to increase blood pressure. This reflex activity is essential to prevent the orthostatic hypotensive response that can occur when moving from the reclining to the upright position. The pressor effect on SBP and PP, which normally occurs when moving into an upright position, changes with aging. The reduction in autonomic control may result in orthostatic hypotension if the elevated PPs do not adequately compensate (Cleophas & Van Marum, 2003). A decrease in overall baroreceptor activity coupled with the decreased compliance of the vessel wall hinders the short-term regulation that normally occurs in the cardiac and vascular systems as a result of body position changes.
Deconditioning is another physiological state that results in an exaggerated orthostatic response. Many elderly individuals are sedentary and therefore deconditioned. This state results in less efficient oxygen transport and poorly functioning skeletal muscles. A deconditioned person needs more energy to perform tasks and is less able to adapt quickly and efficiently to alterations in the body’s homeostasis. Thus, the exaggeration of the orthostatic response during body position changes can be great. Any health professional working with an elderly patient must be aware of this potential for an increased orthostatic response and know how to monitor and treat it.
Typical alterations in the vascular response to exercise with aging
The ability to adapt and respond to the changing needs of the body during exercise is an essential function of the vascular system. One of the primary differences in the response to exercise in the elderly is the more rapid onset of fatigue, resulting from the demands placed on the cardiovascular system. The structural and functional changes in the vascular system impede the ability of the vasculature to supply the tissues with the increased oxygen needed during exercise. Maximal aerobic power refers to the body’s ability to transport and use oxygen ( max). Oxygen consumption increases linearly with the intensity and magnitude of exercise. With aging,
max decreases as a function of body weight and age-related changes in the oxygen transport system, including the reduced ability to use oxygen and to shunt blood flow to active muscles. This deficit often causes an older individual to reach fatigue more quickly when exercising (see Chapter 6).
In a study comparing the responses of young and old adults to peak exercise, Stratton et al. (1994) reported differences in several measured variables. There was a lower heart rate response and a smaller increase in ejection fraction. Systolic, diastolic and mean blood pressures were higher in the old than in the young. During submaximal exercises, there were no differences in ejection fraction between the young and the old.
Under normal circumstances, exercise or any other period of increased activity is sympathetically mediated and results in an increased release of the adrenergic mediators. This in turn raises the activity level in most body systems and triggers an increase in cardiac output and oxygen transport during exercise. The age-associated changes in beta-adrenergic receptors in the vascular system lessen the ability to facilitate the increased need for oxygen delivery during exercise (Stratton et al., 1994).
At a more local level, the peripheral vessels are less responsive to alterations in metabolic activity. Normally, increased metabolic activity in skeletal muscle results in vasodilatation to meet the oxygen demand of the tissue. The stimulation of skeletal muscle vascular adrenergic receptors during exercise also results in vasodilatation. Vessels in older individuals have less ability to vasodilate in response to increased metabolic activity. Older individuals also demonstrate decreased activity mediated through the adrenergic receptors. The inability to increase blood supply quickly coupled with a decrease in structural ability to vasodilate prevents blood from being shunted rapidly from areas of low metabolic activity to areas of more active muscle metabolism. Loss of this mechanism decreases an older individual’s ability to do skeletal muscle work (Evans, 1999).
When exercising, older individuals have been shown to have a higher percentage of their cardiac output shunted to the skin and viscera and a lower percentage directed toward working muscle. Thermoregulation is decreased in the elderly as a function of the loss of muscle mass associated with age and inactivity (Marks, 2002). Normal thermoregulation relies on the processes of conduction, convection and evaporation. Sweating decreases with aging (Marks, 2002). Evaporation, which is sympathetically mediated, is the predominant mechanism for heat loss during exercise. Older individuals attempt to compensate for this loss by shunting more cardiac output to the skin to regulate heat loss via conduction and convection, which are not efficient mechanisms for adequate heat loss at rest or during exercise. This shunting also prevents the delivery of an adequate blood volume to skeletal muscle (see Chapter 10 Thermoregulation).
With aging, muscle capillary density decreases and further limits the blood supply available to working muscle. An important measure of the oxygen transport function is the arteriovenous oxygen difference, which is a measure of the utilization of oxygen by working muscles. Changes in skeletal muscle tissue structure, mitochondria and metabolic enzymes result in a decreased arteriovenous oxygen difference, which indicates that less oxygen is being extracted from the capillary bed for use during exercise.
Typical alterations in the vascular response to exercise training with aging
Stratton et al. (1994) showed that exercise training had significant effects on all vascular variables except end-systolic volume index. They also showed that the increase in maximal oxygen consumption and workload, and the percentage increases, were not significantly different between old and young. They concluded that, despite differences in the response to a single episode of exercise, similar changes in cardiovascular functions in old and young men occurred as a result of endurance exercise training.
Marks (2002) reported a decrease in max associated with aging, specifically a loss of 9–15% between the ages of 45 and 55, with accelerations in losses between the ages of 65 and 75 and further accelerations from 75 to 85. This decline in aerobic power can be improved by 10–25% in older individuals who participate regularly in aerobic exercise. The loss of
max in women is greater than in men. Marks (2002) reported on studies indicating that an increase in walking of 2 miles per day may be as effective as traditional exercise for lowering blood pressure in women.
In a cross-sectional study of healthy men, DeSouza et al. (2000) concluded that regular aerobic exercise can prevent age-associated loss of endothelium-dependent vasodilatation (EDV). Aerobic exercise can also restore EDV in previously sedentary middle-aged and older healthy men. The aerobic exercise intervention consisted primarily of walking.
Carotid artery compliance decreases by 40–50% in healthy sedentary men and women between the ages of 25 and 75 years. Regular aerobic exercise attenuates the loss and was shown to restore compliance to some degree (Seals, 2003).
In a case controlled study of 17 patients with chronic obstructive pulmonary disease (COPD), Vivodtzev et al. (2010) investigated whether exercise training reduces arterial stiffness. The results indicated an improvement in arterial stiffness in patients in the training group, as evidenced by a reduction in PWV proportional to changes in exercise capacity measured as changes in maximal heart rate and oxygen consumption.
Conclusion
Age-associated changes occur in all of the various components of the oxygen transport system, including the vasculature. In rehabilitation, it is important to note that strength and fitness training programs in the elderly have been shown to decrease the amount of decline in function of many bodily systems, including the vascular system. Although training will not entirely eliminate the inevitable decline that occurs with advancing age, the severity of the decline will be lessened.
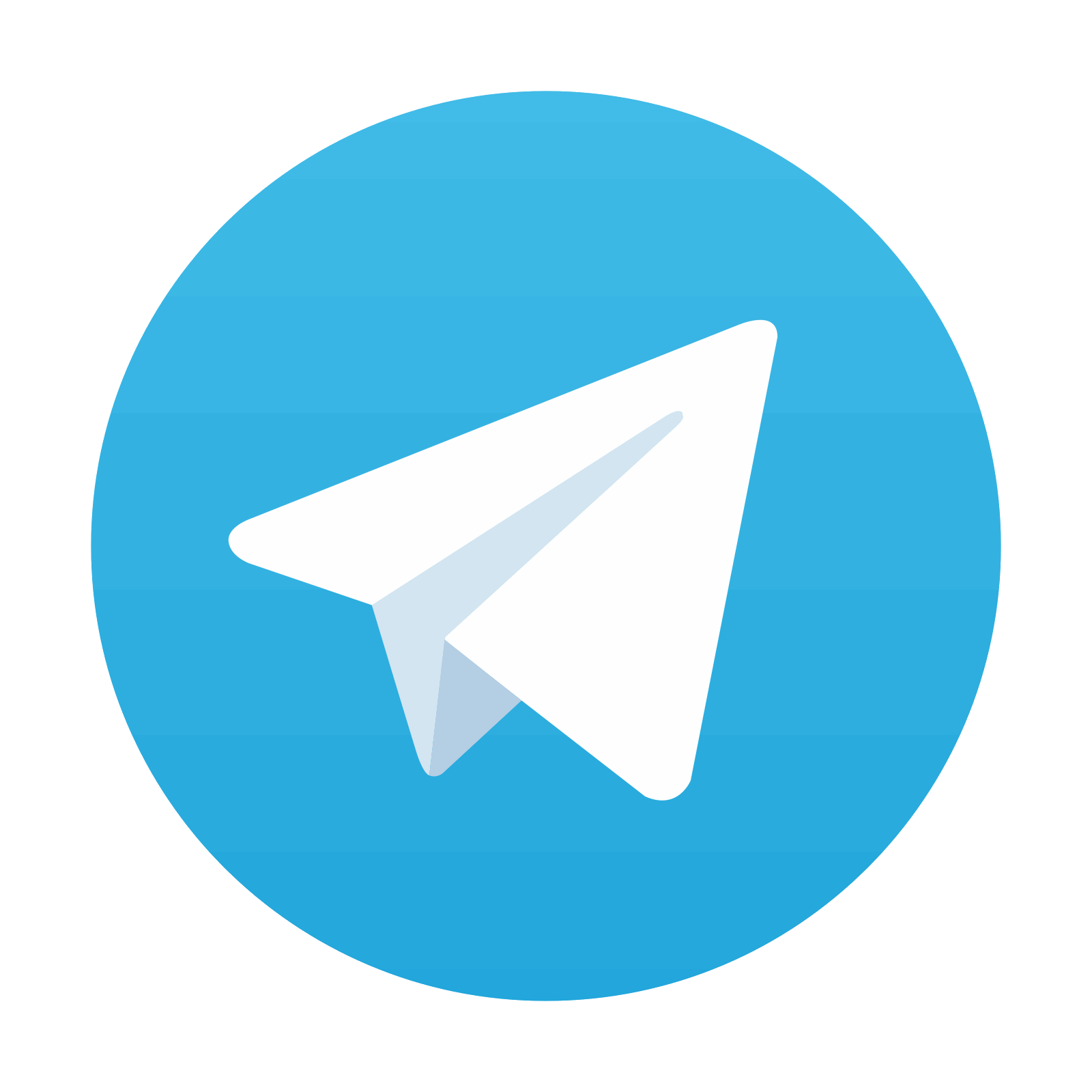
Stay updated, free articles. Join our Telegram channel

Full access? Get Clinical Tree
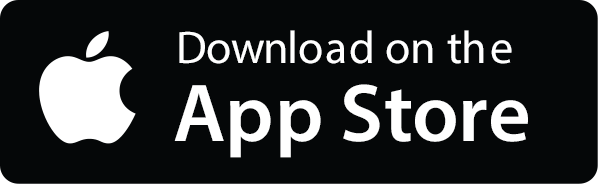
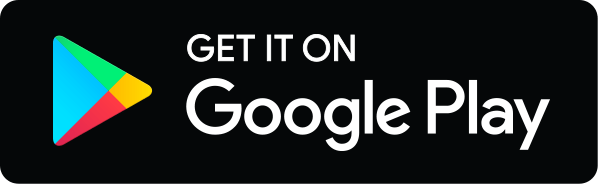