This review primarily focuses on how the macromolecular composition and architecture of articular cartilage and its unique biomechanical properties play a pivotal role in the ability of articular cartilage to withstand mechanical loads several magnitudes higher than the weight of the individual. Current findings on short-term and long-term effects of exercise on human articular cartilage are reviewed, and the importance of appropriate exercises for individuals with normal and diseased or aberrated cartilage is discussed.
Joints are composed of specialized connective tissues which, from a functional point of view, act synergistically to effectively and efficiently deal with the mechanical loads encountered over a lifetime. When performing various tasks such as standing, walking or running, the knee frequently encounters forces up to several magnitudes higher than body weight.
Articular cartilage plays a crucial role in maintaining mechanical competence by providing an almost frictionless gliding surface of synovial joints. Under physiologic conditions, knee cartilage has the unique capability to withstand hydrostatic pressure and the ability to uniformly transmit and dissipate enormous forces across the joint from one subchondral bone to the other during motion. These cartilage features are due to its molecular composition and 3-dimensional architecture, coupled with its highly hydrated state and biomechanical properties. As such, articular cartilage has the ability to withstand high compressive forces associated with not only activities of daily living but also activities with markedly increased locomotive stresses such as sports. However, the ability of the cartilage to perform its biomechanical functions can be compromised by changes in tissue properties that occur with normal aging, injury, or diseases such as chondromalacia patella, osteochondritis dissecans, osteoarthritis (OA), and rheumatoid arthritis.
Traditionally, the effects of exercise on articular cartilage have been examined in animal models, and little information was available on human cartilage. Recent improvements in magnetic resonance imaging (MRI) technology has allowed visualization of human articular cartilage in vivo enabling researchers to investigate the variations in the morphologic and biochemical components of human knee articular cartilage during loading and compressive forces while exercising as well as in diseased states.
Physical exercise, performed frequently over time, has been shown to increase bone and muscle mass (eg, body building), whereas states of inactivity or microgravity have been associated with tissue atrophy. The biosynthetic activity of chondrocytes has also been experimentally shown to be regulated by mechanical stimuli. Based on these in vitro findings, mathematical models have been developed that explain the variable thickness of cartilage between joints based on differences in mechanical loading magnitude. Current findings suggest that the human cartilage deforms very little in vivo during physiologic activities and recovers from deformation within 90 minutes after loading.
Several studies have shown that with immobilization cartilage undergoes atrophy when subjected to reduced loading conditions and that cartilage thickness is only partially restored with exercise. Both clinical and animal research have shown that continuous passive motion of the joint, after joint procedures, is an important stimulus for articular cartilage regeneration. Cartilage adapts to mechanical stimuli by altering its morphology, 3-D architecture, and composition (proteoglycan, collagen, and interstitial water content). This article reviews the structural and biochemical composition of articular cartilage and the macroenvironment and microenvironment of chondrocytes. Also summarized are the effects of exercise on articular cartilage macromolecular synthesis and degradation as well as the extent to which the level of exercise would result in beneficial effects on normal and injured knee cartilage.
Articular cartilage
Articular cartilage is a highly specialized connective tissue with biophysical properties consistent with its ability to withstand high compressive forces. The biochemical properties and organization of the macromolecular constituents of the cartilage matrix and their interaction with water molecules is the key to the shock-absorbing property of the tissue.
During growth and development of long bones (a period from fetal development to cessation of bone growth), articular cartilage goes through a series of structural, morphologic, and biochemical changes. Adult cartilage is typically avascular, alymphatic, and aneural. As such, nourishment is provided through long-range diffusion of the joint fluid. Until the epiphyseal plate is fully ossified in childhood, the articular cartilage is capable of receiving some nutrition through diffusion of substances from blood vessels in the subchondral bone. The unique biological and mechanical properties of articular cartilage depend on the cartilage architecture and the interactions between the extracellular matrix (ECM) and the chondrocytes that maintain the cartilage function.
Chondrons
Chondrons are the microanatomical, micromechanical, and metabolically active functional units of articular cartilage. Anatomically, a chondron comprises the chondrocyte and its pericellular microenvironment. Morphologically, a transparent pericellular glycocalyx is present on the chondrocyte surface and is enclosed by a fibrillar pericellular capsule containing type VI collagen. The composition and structural integrity of the chondron pericellular microenvironment have been shown to influence the chondrocytic response to experimental osmotic challenge. The chondron plays an important role in vivo to maintain the homeostasis of the articular cartilage and to mediate the chondrocytic response to physicochemical changes of the cartilage macromolecules and the movement of water and ions within the matrix. Thus, chondron acts hydrodynamically to protect the integrity of the chondrocyte and its pericellular microenvironment during compressive loading.
Throughout life, the articular cartilage undergoes continual internal remodeling. Chondrocytes can synthesize and secrete the cartilage macromolecular components and can also degrade the matrix by releasing degradative enzymes, such as collagenase, and other metalloproteinases, including stromelysin. During growth and development, synthesis outweighs the degradation. In adults, matrix synthesis is finely balanced by controlled matrix degradation. Hence, chondrocytes continually replace the matrix macromolecules lost during normal degradation. However, disruption to the normal balance of synthesis and degradation can lead to variation in the intrinsic characteristics of the cartilage zones, resulting in a gradual degeneration of the ECM that is responsible for the development of clinically recognizable diseases.
Chondrons
Chondrons are the microanatomical, micromechanical, and metabolically active functional units of articular cartilage. Anatomically, a chondron comprises the chondrocyte and its pericellular microenvironment. Morphologically, a transparent pericellular glycocalyx is present on the chondrocyte surface and is enclosed by a fibrillar pericellular capsule containing type VI collagen. The composition and structural integrity of the chondron pericellular microenvironment have been shown to influence the chondrocytic response to experimental osmotic challenge. The chondron plays an important role in vivo to maintain the homeostasis of the articular cartilage and to mediate the chondrocytic response to physicochemical changes of the cartilage macromolecules and the movement of water and ions within the matrix. Thus, chondron acts hydrodynamically to protect the integrity of the chondrocyte and its pericellular microenvironment during compressive loading.
Throughout life, the articular cartilage undergoes continual internal remodeling. Chondrocytes can synthesize and secrete the cartilage macromolecular components and can also degrade the matrix by releasing degradative enzymes, such as collagenase, and other metalloproteinases, including stromelysin. During growth and development, synthesis outweighs the degradation. In adults, matrix synthesis is finely balanced by controlled matrix degradation. Hence, chondrocytes continually replace the matrix macromolecules lost during normal degradation. However, disruption to the normal balance of synthesis and degradation can lead to variation in the intrinsic characteristics of the cartilage zones, resulting in a gradual degeneration of the ECM that is responsible for the development of clinically recognizable diseases.
Cartilage extracellular matrix
The ECM of cartilage (95% cartilage volume) is a resilient gel comprising 60% to 80% (cartilage wet weight) tissue fluid with a complex macromolecular organization consisting mainly of type II collagen and proteoglycans, which in humans represent 55% and 35% of cartilage dry weight, respectively. Evidence exists that there are interactions between proteoglycan aggregates and collagen fibers and that collagen-proteoglycan binding increases with age. The intrinsic physical properties of collagen fibers (relatively insoluble with high tensile strength) and proteoglycan (soluble with high fixed charge density and compressive rigidity) primarily determine the biomechanical properties of cartilage. The compressive properties of articular cartilage are a function of the balance between the osmotic swelling generated by water bound to the sulfate and carboxylate groups of the proteoglycan and the tension developed in the collagen network surrounding the proteoglycan. The noncollagenous proteins in cartilage ECM include fibronectin, anchorin, tenascin, cartilage oligomeric matrix protein, and cartilage matrix glycoprotein.
Articular cartilage collagens
Five genetically distinct collagen types are known to exist in adult articular cartilage. Type II collagen (90%–95% of the total cartilage collagen types) is the major structural protein in cartilage and is also essentially unique to cartilaginous tissues. Through its high tensile strength, type II collagen provides the structural integrity and resiliency to articular cartilage. Type XI collagen, a fibril-forming collagen, contributes to about 2% to 3% of the total collagen and is incorporated in the type II collagen fiber in a ratio of about 1:30 in mature tissues. Type XI collagen is thought to mediate physical interactions between collagen fibrils and proteoglycans in cartilage and to regulate the size of the type II collagen fibers. Type IX collagen represents 1% to 2% of collagen in adult cartilage and at least 10% in fetal cartilage. Majority of type IX collagen is covalently cross-linked to the surface of type II collagen fibril. Type IX collagen is also distributed in the ECM without association with type II collagen. Type IX collagen contains chondroitin sulfate or dermatan sulfate glycosaminoglycan side chains, which are thought to stabilize type II collagen fibril structure. Type VI collagen, a short-helix molecule concentrated pericellularly that represents 1% to 2% of the total collagen, helps mediate the attachment of chondrocytes to the macromolecular framework of the matrix. Type X collagen (1%), a short nonfibrillar collagen present adjacent to hypertrophied chondrocytes and in the calcified zone, is thought to play an important role in the development of the growth plate and cartilage calcification.
Collagen fibrils are stabilized by covalent cross-links formed between adjacent collagen chains (intramolecular cross-link) and adjacent collagen molecules (intermolecular cross-link). The tensile strength of the collagen fibers is dependent on the formation of intramolecular cross-links. Pyridinoline cross-links are abundant in cartilage collagen, and their concentration remains relatively constant with age.
Proteoglycans
Proteoglycans are strongly hydrophilic, thus providing the lubrication and shock-absorbing property (resistance to compression) of articular cartilage. Proteoglycans are composed of a protein core onto which 1 or more glycosaminoglycan chains are covalently bonded. The glycosaminoglycan molecules are unbranched chains of repeating disaccharides. Hyaluronic acid is the only glycosaminoglycan that is not bound to a core protein and is nonsulfated. The heterogeneity of proteoglycan structure is a reflection of variation not only in the protein core but also in the type and size of the glycosaminoglycan chains. Variation in the position of sulfation can also increase diversity in the chemical and physical properties of the glycosaminoglycan chains. The glycosaminoglycan groups present in cartilage proteoglycan are primarily chondroitin sulfate (87%), which exists both as chondroitin-4-sulfate and chondroitin-6-sulfate, as well as keratan sulfate (6%) and hyaluronic acid. In articular cartilage, large aggregating proteoglycans (aggrecan and versican) form 50% to 58% of total proteoglycans, whereas large nonaggregating proteoglycans form 40% of total proteoglycans. The relative concentration of glycosaminoglycan varies markedly with age. In immature cartilage, there is a preponderance of chondroitin-4-sulfate and little keratan sulfate. With advancing age, there is an appreciable increase in keratan sulfate content and a corresponding decrease in chondroitin-4-sulfate.
Noncollagenous proteins
Small noncollagenous proteins and glycoproteins present in the cartilage ECM are thought to modulate several fibril properties. Although glycoproteins form a small fraction (2%–5%) of the cartilage ECM, they play an important role in matrix assembly and/or regulation of matrix metabolism. Cartilage oligomeric matrix protein, a 524-kDa glycoprotein, is found in cartilage during chondrogenesis. It is preferentially localized in the territorial matrix surrounding the chondrocytes. Biglycan, decorin, and fibromodulin are members of a family of structurally related proteoglycan. They bind to the ECM macromolecules and help to stabilize the matrix. Biglycan, a 100-kDa molecule with a core protein of 38 kDa, is localized in the pericellular matrix. Decorin, a 74-kDa proteoglycan with a core protein of 36 kDa, is present throughout the interterritorial ECM with increased amounts in the superficial zone of articular cartilage, is thought to mediate interactions between aggrecans and collagens. Fibromodulin (59 kDa), a small collagen-binding proteoglycan, is abundant on cartilage surface adjacent to the bursae. Fibronectin is a glycoprotein of the ECM that participates in matrix assembly and affects cell adhesion, morphology, migration, and differentiation. Fibronectin plays an important role in the adhesion of chondrocytes to ECM and is implicated in tissue repair. Tenascin, an oligomeric glycoprotein, functions in processes such as wound repair and cartilage formation and is thought to influence interactions between the chondrocytes and the cartilage matrix.
Cartilage matrix glycoprotein (molecular weight = 54 kDa) is a major component of the cartilage ECM, which is thought to bind and bridge type II collagen fibrils. Matrix Gla protein is a vitamin K–dependent 10-kDa protein that is known to inhibit cartilage calcification. Anchorin CII (31-kDa noncollagenous protein) mediates in anchoring chondrocytes with type II collagen, whereas chondronectin specifically mediates the attachment of chondrocytes to type II collagen. Link protein (molecular weight = 45 kDa) binds to both cartilage aggrecan and hyaluronic acid in ECM, thereby stabilizing their aggregation.
Cartilage matrix compartmentalization
Articular cartilage consists of morphologically distinct zones that serve varied functions, including the formation of an articulating surface and a compression-resistant core as well as its attachment to the bone. The uncalcified cartilage can be distinguished microscopically into 3 zones, which are parallel to and extend from the articular surface to the tidemark. The differences between the zones are based on chondrocyte morphology and distribution, collagen and proteoglycan composition/concentration, collagen architecture, and water content. The uncalcified articular cartilage is attached to the subchondral bone via a narrow layer of calcified cartilage. In adults, a densely basophilic calcified line called the tidemark demarcates the interface between uncalcified and calcified cartilage.
The superficial zone forming the cartilage surface adjacent to the synovial space is characterized by small ellipsoid chondrocytes with their long axis parallel to the articular surface. The collagen fibers are densely packed and orientated parallel to the articular surface. The middle zone consists of large, round, and randomly distributed chondrocytes with gothic arch-like architecture of collagen fibers. The deep zone consists of large chondrocytes arranged in longitudinal columns that are oriented perpendicular to the articular surface and with radially oriented collagen fibers. The calcified zone is characterized by small chondrocytes embedded in a heavily calcified matrix.
In immature cartilage, the distinction between the middle zone and deep zone is less apparent. The cells just beneath the superficial zone have proliferative activity. This activity ceases with maturity, but all chondrocytes continue to have latent capacity to proliferate and regenerate matrix. Cellularity is considerably reduced during development, especially in the deep zone. In immature cartilage, proteoglycan content is least in the middle zone compared with the articular and deep (epiphyseal) zones. Whereas the superficial zone of the mature cartilage has the highest collagen content and lowest proteoglycan content, the middle zone has the lowest collagen content.
Matrix compartmentalization studies have revealed a clear subdivision of the middle and deep zones into pericellular, territorial, and interterritorial matrices. Each chondrocyte cell membrane is immediately surrounded by pericellular (or lacunar) matrix, which is characterized by the absence of fibrillar collagen and abundance of proteoglycan. The pericellular matrix is composed of a mixture of hyaluronan, sulfated proteoglycans, biglycan, glycoproteins, fibronectin, laminin, and collagen type VI and IX. Encapsulating the pericellular matrix is the territorial (or capsular) matrix, characterized by a fine network of fibrillar collagen. The chondrocytes establish contact with these collagen fibrils by extending fine cytoplasmic processes. Adjacent to this is the outermost matrix compartment, the interterritorial region, which constitutes the largest domain of the matrix and lies in the space between various territorial matrices. The interterritorial matrix is characterized by collagen fibers interspersed with varying concentrations of proteoglycans, depending on the zone in which the chondrocytes lie.
Aging of articular cartilage
Aging is associated with changes in the biochemical activity of the chondrocytes resulting in alterations in the ECM composition and decreased ability of articular cartilage to withstand compressive forces. This in turn could accelerate the cartilage degradation. Mitrovic and colleagues documented age-related decrease in cell density in all zones of the human femoral condyle articular cartilage, although more markedly in the superficial zone. Vascularity of the cartilage calcified zone (a sign of remodeling) is well developed after 55 to 65 years of age. Reduction in the cartilage fluid content from 70% to 80% (normal cartilage wet weight) to 50% to 65% accompanies the aging process, especially in the deeper zone. Using bovine patella articular cartilage (with intact surfaces) from immature, mature, through to mildly degenerated tissue, Broom and colleagues showed a consistent pattern of increased free swelling of the isolated cartilage matrix with age and degeneration. In humans, with aging, the knee cartilage is especially susceptible to becoming worn down and weakened, given the loads it is subjected over the years. Because of age-related changes in cartilage fluid and macromolecular content, structure, and architecture, the capability of articular cartilage to withstand compressive forces also decreases with aging.
Effect of physical activity on articular cartilage and adjacent tissues
Joint exercise not only increases the blood flow to the various connective tissues of the joint but also keeps the joints lubricated. Consistent low to moderate daily activity facilitates cycles of catabolism and anabolism within these tissues. When breakdown and repair are well balanced, homeostasis of joint tissues is maintained. However, a drastic increase or decrease in the intensity of the physical activity results in homeostatic imbalance. Often, after long periods of immobility, the joints become stiff and lose some of their range of movement. As such, strengthening of the knee tissues through regular exercise is of paramount importance.
Articular cartilage resides within the joint interior, and its health is dependent to a large extent on the integrity of other components such as the synovium, muscle, ligaments, tendons, and bone. Exercise has a number of effects on the skeletal system, both in the short and the long term. However, injuries due to extreme or improper exercise can not only affect the bone, muscle, ligament, or tendon but also unfavorably affect the articular cartilage, causing joint swelling, pain, and stiffness. Frequent movement of the joints can relieve the symptoms of pain due to increased circulation of tissue fluid in the joints. Weight-bearing exercise such as walking, jogging or running has several benefits, including improved blood circulation and decreased tissue fluid stagnation in a given joint area. Synovial fluid produced by the synovial membrane within the joints has a short-term response to exercise, thus requiring regular exercise to stay lubricated, nourished, and healthy.
Several studies have alluded to the significant effects of exercise on the bones, muscles, tendons, ligaments, and cartilage of the skeletal system. Weight-bearing exercises, such as strength training and running, can stress long bones. In response to this stress, the osteoblasts build new bone, specifically at the sites of stimulus, resulting in greater bone density that is more capable of withstanding the impact when consistently stimulated. The bone is continuously resorbed and remodeled in response to external mechanical loads. However, excessive weight-bearing exercise without sufficient recovery results in greater breakdown than rebuilding ratio and could eventually contribute to stress fractures or osteoporosis. As such, high-impact exercise may cause harm to bones and other joint tissues due to high level of mechanical stress. Likewise, too little weight-bearing exercise will not provide a stimulus for bone building and lead to overall bone loss.
Increases in sports performance such as marathon running can be at least partly attributed to strengthening of connective tissue components of the joint as well as adaptations in muscle, although the cellular mechanisms underlying these adaptations remain unclear. Of the skeletal tissues, muscle responds most rapidly to exercise. The muscles used in the particular exercise rebuild themselves by synthesizing more muscle proteins and increasing in size. Consistent exercising of these muscles is necessary to maintain this size increase. Without adequate stimulation via exercise, the particular muscles will experience an accelerated catabolic activity resulting in muscle atrophy as observed during the extended period of immobilization or non–weight bearing after knee surgery.
The integrity of tendons and ligaments is critical for skeletal system function. Because tendons and ligaments connect bones to muscles and bones to bones, respectively, they facilitate coordinated movement by transmitting force across the joints. When exposed to regular exercise, ligaments become stronger and more resistant to injury. Because ligaments have limited blood supply, adaptations of any kind are slow to develop. A study on the effect of certain types of exercise on ligaments and tendons revealed that the cross-sectional area of both structures increases in response to running and other load-bearing activities and conversely decreases during periods of immobilization postsurgery. Tendons and ligaments are bundles of collagen-containing fibers that transmit muscle force across the joint. Consistent stimulation through exercise increases the size, density, and number of collagen fibers making up tendons and ligaments. In addition, a stronger muscle transmits more force through the tendon, increasing its strength over time.
Articular cartilage is a highly hydrated specialized connective tissue with the capability to withstand impact forces. The therapeutic value of exercise to cartilage is now known. Regular exercise facilitates diffusion of nutrients through the cartilage, and moderate exercise likely improves the molecular composition of cartilage. Too little exercise or immobilization eventually compromises the lubricant properties of cartilage, although this can be reversed with more activity. However, too much loading on the cartilage at the joints can lead to OA, characterized by excessive cartilage breakdown and eventual cartilage loss.
Research on the effects of exercise on cartilage has shown that similar to bone, cartilage tends to weaken without regular loading. However, unlike bone, cartilage does not actually appear to thicken even in highly active people such as elite athletes, although studies contradicting this observation exist. Researchers agree that vast differences in cartilage thickness between individuals exist, but what remains unclear is whether exercise is a significant contributor to this finding. Using MRI to accurately measure cartilage thickness during loading, Gratzke and colleagues showed that direct measurements of muscle forces do not predict cartilage thickness more accurately than muscle cross-sectional areas. Their findings suggested that relative to muscle thickness, cartilage thickness has much less ability to adapt to mechanical loading.
Joint arthropathy and effect of exercise
Exercise is important for healthy individuals as well as for people with conditions such as chondromalacia patella and arthritis and in those who have had a surgical procedure involving the knee joint. Studies have shown that maintaining healthy weight and keeping joint tissues strong through regular exercise is beneficial for patients with the above-mentioned conditions.
Chondromalacia patella is a term used to describe softening of the articular cartilage on the underside of the patella. Chondromalacia can occur by simple wear and tear on the knee joint or arthritis as people age. In young individuals, especially those who are actively involved in sports, chondromalacia is most likely a consequence of an acute injury such as a traumatic fall, repetitive overuse, knee malalignment, or even muscle weakness. This injury is common in runners, skiers, cyclists, and soccer players. Frequently, chondromalacia is associated with a dull pain under or around the patella that increases while walking downstairs or climbing upstairs as well as sitting or getting out of a chair. Low-impact exercise for chondromalacia strengthens the muscles, particularly the inner part of the quadriceps. Swimming, stationary bicycle, and cross-country skiing are good ways to strengthen the joint with minimal impact.
OA is the leading cause of disability among adults, resulting in knee pain, swelling, and stiffness. With increasing age, the “sponginess and cushioning” ability of cartilage is subject to degeneration due to wear, a process that can be exacerbated by previous injury, resulting in OA. Exercise is one of the most effective ways to reduce pain and improve function in patients with knee OA. Strength training as well as low-impact exercises such as cycling, tai chi, and swimming can also reduce pain in the knee affected by OA.
Based on the erroneous wear-and-tear concept of OA, the commonly held myth is that exercise will aggravate cartilage loss. However, with the advancement of MRI technology, Roos and colleagues were able to determine the impact of moderate exercise on the knee cartilage of subjects at high risk for developing OA. This 4-month, randomized controlled trial was aimed to investigate the effects of moderate exercise in 45 patients (29 men and 16 women; age 35–50 years) with partial medial meniscus resection 3 to 5 years before the study period. Subjects were randomly assigned to either an exercise group or a control group. The exercise group was enrolled in a supervised program of aerobic and weight-bearing exercise, for 1 hour, 3 times weekly for 4 months. At the study’s onset and follow-up, subjects from both groups underwent MRI scans to evaluate knee cartilage morphology and glycosaminoglycan content. Subjects also answered a series of questions regarding their knee pain and stiffness, as well as their general activity level. In the exercise group, many subjects reported improvements in physical activity and functional performance tests compared with subjects in the control group. Their study showed that moderate exercise in these patients not only improved the joint symptoms and function but also increased the cartilage glycosaminoglycan content. The investigators corroborated previous findings that supported the therapeutic value of exercise for patients with OA, for improving not only joint symptoms and function but also the quality of knee cartilage as seen on MRI. This implies that human cartilage responds to physiologic loading in a way similar to that exhibited by muscle and bone and that previously established positive symptomatic effects of exercise in patients with OA may occur in parallel or may even be caused by improved cartilage properties.
Another study, by Racunica and colleagues, investigated the effect of physical activity with varying degrees of intensity, frequency, and duration on the knee cartilage and bone. A total of 297 healthy adults (aged 50–79 years), with no history of knee injury or OA, were recruited for the study. All the individuals underwent MRI examinations of the tibiofemoral joints of their dominant knee. Dominant knee was defined as the knee pertaining to the leg they first step forward when walking. MRI was used to assess cartilage and bone marrow lesions and to measure cartilage volume. Subjects answered specific questions regarding their exercise and walking habits, as well as routine activity at home and at work, to determine their level of physical activity at 6 months and 7 days before the study. Baseline data on body weight, height, body mass index, and physical activity were obtained for each subject. The increase in tibial cartilage volume correlated positively with the frequency and duration of vigorous activity. Also, moderate physical activity, including regular walking, was associated with a lower incidence of bone marrow lesions, indicating more resistance to fracture in the subchondral bone. The study by Racunica and colleagues demonstrated a protective effect of vigorous physical activity on knee cartilage in healthy adults and strongly supports the benefits of exercise for individuals at risk for OA. However, this study contradicts previous reports suggesting that high-level exercise is damaging to cartilage.
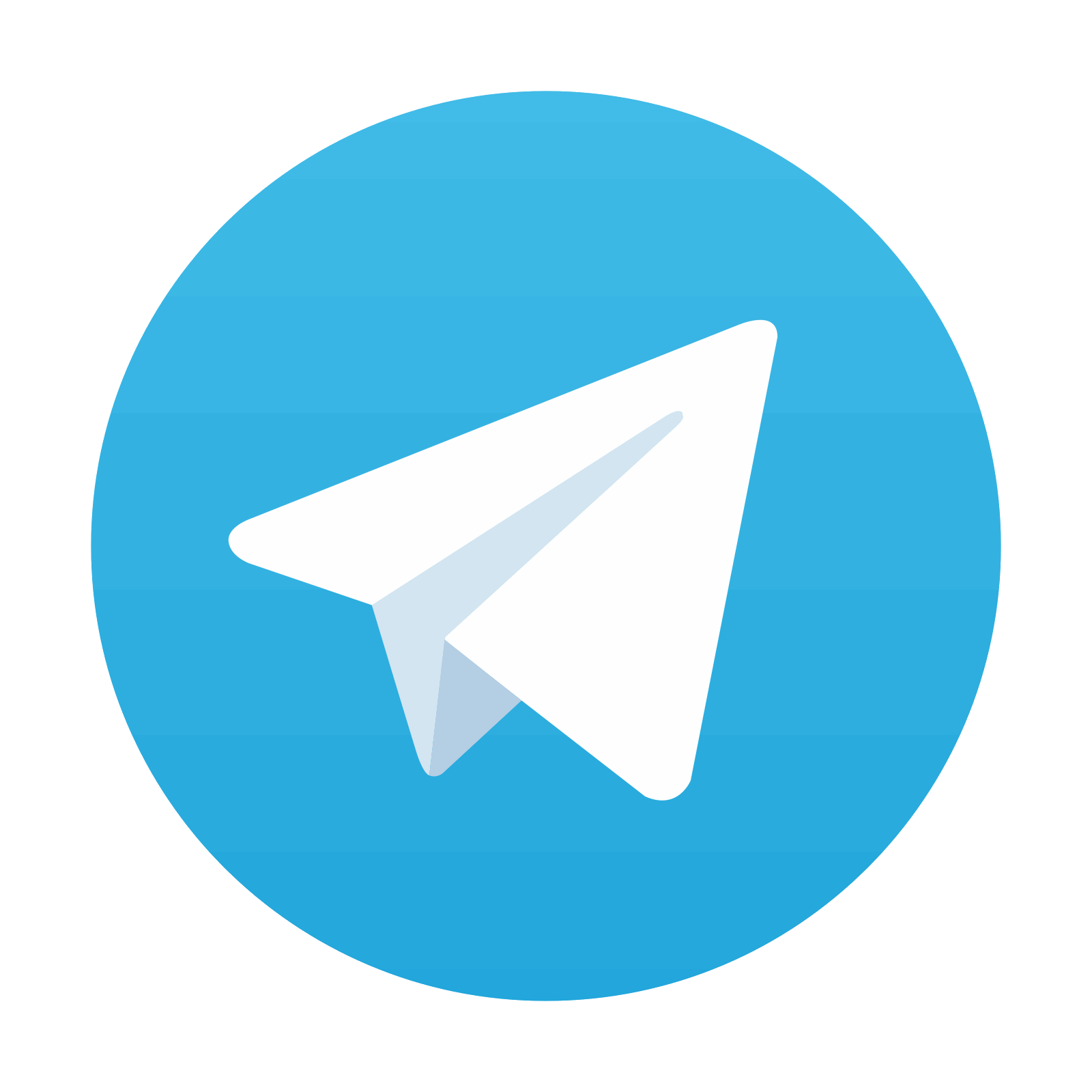
Stay updated, free articles. Join our Telegram channel

Full access? Get Clinical Tree
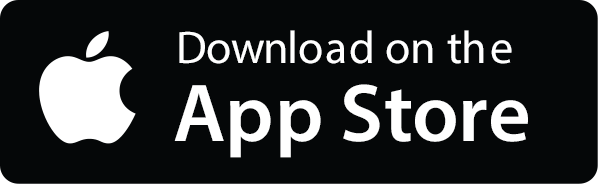
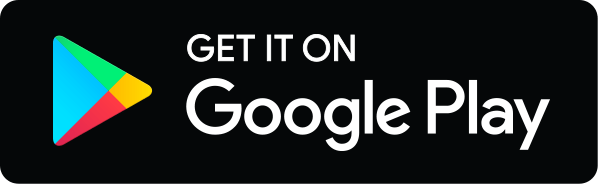