Fig. 40.1
This graph shows the number of in vitro, in vivo, and total studies that are performed on quantitative pivot shift analysis (Reprinted from Lopomo et al. [59] with kind permission of Springer Science and Business Media)
In this book chapter, we discuss (1) the different methods of quantifying the pivot shift test (how to quantify the pivot shift test), (2) the different tools of measuring the pivot shift test (how to measure the pivot shift test), and (3) the different methods of performing the pivot shift test (how to perform the pivot shift test). Finally, we will discuss the future clinical application of an objective and highly reliable pivot shift.
40.2 The Pivot Shift Test
The pivot shift test as described by Galway and MacIntosh is performed starting with the knee in extension [30, 31]. With one hand, the knee is rotated internally, while with the other hand, a gentle valgus torque is applied. The knee is then moved from extension through the flexion arch, while the internal rotation is released. In the ACL-deficient knee, the tibia will translate anteriorly and rotate internally at approximately 10°–15° of flexion. When the knee is further flexed, the tibia will translate posteriorly and rotate externally toward its original position. This so-called reduction phase usually occurs between 30° and 45° of flexion [30, 31, 53]. With this method, the hip should be abducted in 45° to optimize the amount of tibial translation [8]. When the position of the tibia is visualized in the sagittal plane with knee flexion on the y-axis and ATT on the x-axis, a P angle is noted in ACL-deficient knees as described by Lane and colleagues [54, 78]. In the next section, we will discuss several methods of quantifying the pivot shift.
40.3 Methods of Quantifying the Pivot Shift
There are different methods to quantify the pivot shift. Some authors advocate quantifying the pivot shift by the translation of the entire tibia, whereas others only measure ATT of the lateral compartment. ITR and the acceleration of the reduction phase are also elements of the pivot shift phenomenon that could be used to quantify the pivot shift. These methods will be discussed.
40.3.1 Anterior Tibial Translation
In the years after the historical articles of Slocum and Larson and Galway and MacIntosh, several biomechanical studies quantified the anterior-posterior translation of the tibia [15, 28, 66]. These studies described the ATT in the ACL-intact and ACL-deficient knees and found significant results between both. Jakob and colleagues described the subjective grades of the pivot shift [41]. The authors assessed the ATT with anesthesia by forcing the tibia in maximal subluxation under anesthesia and estimating the anterior-posterior translation by hand. They measured both the medial and lateral compartment and found the medial compartment was a better predictor for pivot shift grading. ATT can be measured either by measuring the ATT of both compartments (so-called coupled ATT or cATT) [37] or by measuring the lateral compartment only.
Bedi and colleagues used a mechanized pivot shifter to assess the correlation between different pivot shift grades and the ATT of both the medial and lateral compartment [10]. They performed the pivot shift in 77 cadavers in where they dissected the ACL or a combination of the ACL with the medial collateral ligament, medial meniscus, lateral meniscus, or a combination of these structures. This resulted in different pivot shift grades in the cadavers. A continuous passive motion (CPM) machine was used to mechanize the pivot shift test and measured the ATT in both compartments with tracking of reference points in a three-dimensional motion path system. Contrary to the findings of Jakob and co-workers [41], they found that lateral ATT was a better predictor for a positive pivot shift (grade 0 vs. grade 1) compared with medial ATT. They found there was a threshold of 6 mm of lateral ATT for a positive pivot shift, whereas there was no significant difference between grade 0 and grade 1 in the medial compartment. Knees with a grade 0 pivot shift had an average (± SD) lateral ATT of −2.1 mm (±8.1 mm), with grade 1 an average 11.1 mm (±2.2 mm) and with grade 2 correlated with 19.6 mm (±2 mm) (Fig. 40.2). Therefore, measuring lateral ATT seems useful in the quantification of the pivot shift test.


Fig. 40.2
Lateral compartment translations in mm are shown in the different pivot shift grades during a mechanized pivot shift (Reprinted from Bedi et al. [10] with kind permission of Springer Science and Business Media)
Hoshino and colleagues assessed the lateral anterior femoral translation of the reduction phase in clinical patients, which is similar to a posterior tibial translation since these landmarks are relative to each other [35]. Under anesthesia, they performed a manual pivot shift test, and the movement of the lateral compartment was captured with skin markers and a standard digital camera and was analyzed with a two-dimensional software system. They found in 5 ACL-deficient patients with a subjective pivot shift grade 1 an anterior femoral translation in the reduction phase of 3.7 ± 2.1 mm. These authors then developed an application for a computer tablet (e.g., iPad) and performed a new study where they examined 20 patients [34]. The patients were scheduled for isolated ACL reconstruction and the pivot shift was grade 1 in 10 patients and grade 2 in 10 patients. With the same method, they measured the lateral anterior femoral translation in the reduction phase and found a significant difference between grade 1 (2.7 mm ± 0.6 mm) and grade 2 (3.6 mm ± 1.2 mm). These studies showed that measuring the lateral ATT and posterior translation in the reduction phase are both reliable in quantifying the pivot shift.
40.3.2 Internal Tibial Rotation
The role of ITR in quantifying the pivot shift has also been extensively assessed [14, 21, 23, 40, 60, 62]. Bull and colleagues showed a strong correlation between the pivot shift and ITR [14]. They described that the tibia during the pivot shift test between 0° and 25° flexion showed progressive ITR, and this was suddenly reversed during the reduction phase starting at 36° (±9°) with external tibial rotation. After ACL reconstruction, this external rotation in the reduction phase was significantly reduced. Colombet and colleagues found similar results [17] when they performed manual pivot shift tests in four cadavers and measured the ITR in ACL-intact and ACL-deficient knees. They found that internal rotation was larger in the positive pivot shift (≥ grade 1) compared to the negative pivot shift (27° ± 2° vs. 20° ± 2°). Lane and colleagues found an in vivo significant correlation (R 2 = 0.77) between pivot shift grades and tibial rotation in the reduction phase [54]. Although some studies showed a high ICC for measuring ITR [62, 84], several authors state that there is a lack of validated devices for assessing rotatory knee laxity, and these techniques are considered complex and invasive and are therefore clinically not applicable [2, 3, 5, 21]. Diermann and colleagues performed a simulated pivot shift test at different flexion angles and compared ACL-intact, ACL-deficient, and ACL-reconstructed knees [21]. They did not find significant differences in ITR between the different knees, but they did find significant differences in ATT of the lateral compartment when comparing these knees. They suggested using lateral compartment ATT rather than internal rotation to assess the integrity of the ACL. Hoshino and colleagues also found that tibial rotation is not reliable in differentiating a negative from a positive pivot shift and recommended using lateral ATT or acceleration [36]. Moreover, some systematic reviews of rotational laxity are not convinced of clinical application of measuring internal tibial rotation and state that simple, accurate, and noninvasive devices are needed [2, 3, 5]. It seems there are differences in ITR between different pivot shift grades, but these are not easy measurable with the available devices.
40.3.3 Acceleration
More recently authors have quantified the pivot shift by measuring the acceleration of the tibia in the reduction phase (Fig. 40.3). Several studies have described the acceleration and used acceleration to differentiate between different pivot shift grades. Hoshino and colleagues assessed the acceleration of the reduction phase in 30 clinical patients under anesthesia [37]. They measured the acceleration with an electromagnetic system in a manually performed pivot shift. In the negative pivot shift, the acceleration was −795 mm2/s, while in grade 1, 2, and 3 pivot shifts, the accelerations were, respectively, −1247 mm2/s, −2381 mm2/s, and −2735 mm2/s. These accelerations were significantly different from negative pivot shift, and acceleration of tibial reduction was larger in correlation with higher clinical grading (p < 0.01). Other authors also assessed the relationship between acceleration and pivot shift grades and reported a strong correlation between acceleration and different pivot shift grades [3, 11, 50, 51, 54, 59–61, 65]. Labbe and colleagues assessed different components of pivot shift grades (tibial translation, rotation and acceleration) in 127 in vivo pivot shifts [52]. They found that acceleration of tibial translation better distinguished the different pivot shift grades than tibial translation and tibial rotation. Ahlden and colleagues compared the relationship of acceleration and ATT with clinical pivot shift grades using electromagnetic sensors and skin sensors [1]. With both methods, the authors found a stronger correlation between acceleration and clinical pivot shift grades than ATT with clinical grades. Contrary, Lopomo and colleagues showed in a linear regression that rotational laxity before and after reconstruction is better evaluated by measuring lateral ATT compared with measuring acceleration [58]. However, there are many studies in the literature that support using both lateral ATT and acceleration in quantifying the pivot shift and different pivot shift grades. Because several studies question the use of tibial rotation in quantifying the pivot shift, development of simple devices is necessary before reliable and clinically applicable ITR measurements can be performed.


Fig. 40.3
This graph shows anterior-posterior translation (AP) of the distal femur (y-axis) over time (x-axis) during a manual pivot shift test. In the reduction phase anterior acceleration of the distal femur is seen with 5.7 mm in 0.17 s (arrow) (Reprinted from Hoshino et al. [35] with kind permission of Springer Science and Business Media)
40.4 Tools to Measure the Pivot Shift
Jakob and colleagues were the first to quantify the pivot shift and measured medial and lateral ATT manually [41]. In the same study, they aimed to quantify the pivot shift by taking radiographs while forcing the tibia in a maximal subluxed position. In the years that followed, several different measurement systems were used and these different methods will be discussed.
40.4.1 Six-Degree-of-Freedom Robot
The six-degree-of-freedom robot is used in biomechanical studies. A knee is transected at the proximal tibia and distal femur and the bones are mounted to the robot. The robot is a six-joint manipulator, which can learn complex multiplanar kinematics [27]. The robot can perform a combination of movements that simulate the pivot shift and register the kinematics and loads with the universal force sensor. After ACL dissection, the robot can (1) repeat the intact ACL kinematics and measure the load carried by the ACL and (2) learn the new kinematics in the ACL-deficient knee and measure the pathologic kinematics. The repeatability of this system is very high (within 0.02 mm and 0.02°) [27, 95].
40.4.2 Navigation
With this measurement technique, threaded Steinmann pins are drilled in the proximal femur and distal tibia. Reflective markers are attached to the pins and a sensor can track the position of the markers (Fig. 40.4a) [72]. With repetitive cycling of the leg, the center of rotation can be determined, and the reflective markers can measure the position of the distal femur and proximal tibia. With these positions, a three-dimensional model is created, and ATT and ITR can be measured when the knee is cycled from extension through flexion. This measurement system is accurate within 1 mm and within 1° when compared to a six-degree-of-freedom robot [18, 79]. A disadvantage of this method is the invasive technique where pins are drilled in the femur and proximal tibia. In order to use this method in the clinical setting, noninvasive methods have to be developed. A study by Russell and colleagues has used a noninvasive method by attaching the pins and reflective markers to a fabric band that is strapped around the femur (Fig. 40.4b) [83]. They showed a similar ICC for internal rotation for the noninvasive method (0.94) and invasive method (0.93). Future studies must show the clinical use of these markers in both ATT and acceleration.


Fig. 40.4
Two photographs showing different methods of using reflective markers for navigation tracking; (a) (upper) shows a manual pivot shift with the invasive method of two pins in the distal femur and proximal tibia; (b) (lower) shows the noninvasive method of a strapping and baseplate to which the optical trackers are mounted (Reprinted from Russell et al. [83] with kind permission of Springer Science and Business Media)
40.4.3 Radiological Imaging
Some studies have used radiological imaging techniques to quantify the pivot shift. As previously discussed, Jakob and colleagues used radiography of the subluxed tibia to measure the ATT [41]. More recently, magnetic resonance imaging (MRI) is used to quantify the pivot shift [23, 32, 76, 77]. Okazaki and colleagues were one of the first to use an open MRI for the quantification of the pivot shift [76]. Patients with ACL-intact knees or ACL-deficient knees were scanned with and without manual application of anterior drawer force. In the ACL-intact knee, the lateral ATT was without stress −2 mm ± 1.5 mm and with stress 8.7 ± 8.0 mm. In the ACL-deficient knee, the lateral ATT was 8.7 mm (±8.0 mm) without stress. and with stress the lateral ATT was increased to 14.4 mm (±5.5 mm). The intra-rater reliability and inter-rater reliability for the lateral compartment were, respectively, 0.98 and 0.91. Although the MRI showed reliable lateral ATT increase in the ACL-deficient knee, it is expensive and not applicable in the clinical setting. Furthermore, it is difficult to use this for dynamic laxity evaluation.
40.4.4 Radiostereometric Analysis
Radiostereometric analysis (RSA) is an imaging technique that can be used to measure knee laxity in a dynamic setting [26]. With this technique, tantalum beads are implanted in the tibia and femur, and with biplanar radiographs, the exact amount of ATT can be measured while a patient is walking or running. The measurements are accurate within 10–250 μm and 0.03–0.06°, and an important advantage of this method is the possibility to measure the ATT during walking or running [26, 89]. To overcome the invasive implanting of tantalum beads, Tashman and colleagues developed a CT-imaging technique [88]. Although RSA can be used noninvasively, these measurements are considered expensive and labor-intensive, and radiation is used [2]. Some authors consider this method as the gold standard [2], but it is not widely applicable in the clinical setting.
40.4.5 Electromagnetic Device
Hoshino and colleagues used an electromagnetic device in clinical patients to quantify the pivot shift test [37]. This device consists of a transmitter that produces an electromagnetic field and three receivers. The receiver is attached to a strap, and two receivers are placed 10 cm above the patella on the proximal femur and approximately 10 cm below the patella. A third receiver was used to digitize several anatomical landmarks (Fig. 40.5). The electromagnetic system has gained increased popularity over the last decade [1, 4, 22, 56, 59, 90] and is shown to have good accuracy for both positioning (0.76 mm) and orientation (0.158°) [74].


Fig. 40.5
This photograph shows the electromagnetic device that is used to quantify the pivot shift test. A transmitter sends signals to two braces with receivers that are strapped around the distal femur and proximal tibia. A third stylus with receiver (upper right) is used to acquire different anatomical landmarks (Reprinted from Hoshino et al. [37] with kind permission of American Journal of Sports Medicine)
40.4.6 Skin Markers
Recently, a measuring system with digital cameras and skin markers has been introduced [34, 35]. With this technique, three skin markers are attached to (1) Gerdy’s tubercle, (2) the fibular head, and (3) the lateral epicondyle. The digital camera records the movement of the skin markers and thus the movement of the lateral femur and tibia (Fig. 40.6). The authors showed in these two studies that this measuring system is able to measure ATT and tibial acceleration in the reduction phase. Advantages of this system are the low threshold for using skin markers and digital cameras in the clinical setting, and this could be of value for future clinical application. However, some problems have to be overcome. First of all, even when the skin markers are properly attached, they are less accurate because they allow motion artifacts between skin and bone [3, 13, 64]. Secondly, although this measuring method ensures objective measurement of the pivot shift test, the manual performing of the pivot shift does not ensure objective results compared to a mechanized pivot shift test [72]. The different performing techniques of the pivot shift will be discussed next.


Fig. 40.6
The setup of quantifying the pivot shift with skin markers is shown in the clinical setting. The three skin markers are placed at Gerdy’s tubercle, the fibular head, and the lateral epicondyle. An iPad is used to measure the movement of the skin markers during a manual pivot shift, while a sheet is placed at the background to minimize interference (Reprinted from Hoshino et al. [34] with kind permission of Springer Science and Business Media)
40.4.7 Inertial Sensor
The use of inertial sensors for the quantification of the pivot shift test [61] will be treated deep in the dedicated chapter.
40.5 Methods of Performing the Pivot Shift
For the purpose of quantification of the pivot shift, three general methods are seen in the literature. In the 1990s, several studies used the biomechanical technique of the simulated pivot shift in order to quantify the pivot shift [58, 59, 87]. Other studies used the manual pivot shift, whereas the senior author (AP) used the mechanized pivot shifter to quantify the pivot shift. These three different methods of performing the pivot shift will be discussed.
40.5.1 Simulated Pivot Shift Test
Many biomechanical studies used the six-degree-of-freedom robot with universal force sensor to quantify the pivot shift with a simulated pivot shift test [22, 29, 44, 45, 48, 49, 59, 67, 97, 99]. Matsumoto and colleagues assessed the role of several structures in the knee on the pivot shift [67], and they found that solitary ACL injury, solitary lateral structure injury, and combined ACL and lateral structure injury could cause a positive pivot shift. With this study, they objectively confirmed the results of other studies that used the manual pivot shift [24, 38, 63]. Kanamori and colleagues further quantified the ATT in the ACL-intact and ACL-deficient knee with the simulated pivot shift [44]. They reported that different ATT between ACL-intact and ACL-deficient knees was seen between 0° and 30° of flexion. In a follow-up study, they assessed the role of internal torque and valgus torque on the ATT of the pivot shift [45]. They found that a 10 Nm valgus torque gave the largest difference in ATT between the ACL-intact and ACL-deficient knee, which is confirmed by others [29]. The largest difference in ATT between the ACL-intact and ACL-deficient knee was seen with a 1.6 Nm rotational torque and a 10 Nm valgus torque. The authors therefore suggested that optimal performance of the pivot shift requires a moderate amount of valgus force with only a small internal rotation torque. Engebretsen and colleagues performed a simulated pivot shift test and compared the applying of different torques and forces. The authors confirmed that coupled internal rotation and valgus torques best recreated the lateral ATT that occurs in the simulated pivot shift test [22].
40.5.2 Manual Pivot Shift Test
Many studies used the manual pivot shift to quantify the pivot shift. One of the great advantages of the manual pivot shift is the fact that no equipment or additional personal is necessary and results are immediately obtained [12]. However, one major disadvantage is the difference in technique among examiners. In a survey among 33 orthopedic surgeons, several differences in technique and reporting outcomes were detected [51]. More specific, 60 % of the surgeons performed flexion from extended position, whereas 40 % performed extension from flexed position, and 70 % applied internal rotation during the pivot shift test, while 30 % applied external rotation. In addition, the authors compared the quantified pivot shift between five examiners with an electromagnetic device. They found no differences in ATT and acceleration between the examiners but did find differences in ITR and when the pivot shift was applied in the contralateral knee [51]. Because of different surgeon preferences in the pivot shift technique, a standardized pivot shift test is suggested [33, 71]. In the first step, the leg is in extension with slight hip abduction and the leg is internally rotated. In the second step, gentle valgus stress is applied and the leg is brought into flexion. The internal rotation is maintained until 20° of flexion and is then released when the knee is further flexed. Between 20° and 40° of flexion, the reduction phase occurs, and posterior translation of the tibia along with external rotation can be measured. Hoshino and colleagues [33] compared the inter-rater variability among 12 orthopedic surgeons and found a smaller variability in the standardized technique compared to their preferred technique. However, it remains difficult to quantify the pivot shift with a manual examination since educational differences and preferences in technique exist among orthopedic surgeons.
40.5.3 Mechanized Pivot Shift
The senior author (AP) and colleagues used a mechanized pivot shifter in order to quantify the pivot shift test in human cadavers. A whole hip-to-toe cadaver is mounted to a setup, and a CPM machine is secured to the table. The foot is placed in a holder and fixed in an internal rotation moment at the knee. A valgus moment is applied with a three-degree-of-freedom arm, and the leg is moved from extension through the flexion arch (Fig. 40.7). The mechanized pivot shift test was compared to the manual pivot shift test and was found to be more accurate in measuring ATT than the manual performed pivot shift test (0.92 vs. 0.76, respectively) [72]. A second-generation mechanized tester was developed which was more accurate than the first generation (0.99 vs. 0.92, respectively) [16]. With this mechanized pivot shift test, the examiners quantified the role of the primary and secondary stabilizers on in ATT and ITR [10, 68, 70, 86, 94]. They were also able to assess the influence of different tunnel positions on knee stability [9, 93] and compared different reconstruction techniques in knee stability with the meniscus [73] and without the meniscus [69] in situ.


Fig. 40.7
A human cadaver is placed in the mechanized pivot shifter, and the examiner pushes the handle of the foot driver component while pulling the handle of the base plate. This causes flexion of the knee and will induce the pivot shift phenomenon. Please note that the foot is in external rotation in this photograph (Reprinted from Citak et al. [16] with kind permission of Springer Science and Business Media)
The mechanized pivot shift is shown to have high ICC and is able to distinguish different pivot shift grades as earlier discussed. However, the setup of this method is invasive since Steinmann pins are drilled in the distal femur and proximal tibia. Therefore, this method is not applicable in the clinical setting. As previously discussed, some studies have examined the application of noninvasive reflective markers on Steinmann pins [83] and found a high ICC for internal rotation. Further research is needed to show reliability in ATT and acceleration. Furthermore, it would be of value to further develop the mechanized pivot shifter to a device that can be easily used in the clinical setting.
40.6 Clinical Application
Several studies have reported objective measuring of the ATT, ITR, or tibial acceleration of the pivot shift, and some of these methods have potential to be of clinical value. Measuring lateral ATT or acceleration is currently the most reliable and cost-efficient in quantifying the pivot shift test [5]. The use of skin markers seems promising since the markers are easy to use, have low costs, and can reliably measure the lateral ATT [35]. Other measurement methods as electromagnetic devices, inertial sensors, and noninvasive reflective markers also have clinical potential [37, 61, 83]. However, a problem with noninvasive methods remains the skin-bone movement and marker-skin movement, and the test should be corrected for these movements [3, 13, 64]. The method of performing the pivot shift test can be manual with a standardized method or with a mechanized pivot shift test. The mechanized pivot shift test is more objective than the manual pivot shift since there are still differences in performing the pivot shift test. However, an easy, smaller, and low-cost mechanizer needs to be developed to enable clinical use as is seen with the KT-1000. Over the last years, several studies have assessed the role of the quantified pivot shift test in the clinical setting. Hopefully, this will lead to the ultimate goal of a worldwide available and affordable device that can be used to diagnose and evaluate the ACL in the clinical setting.
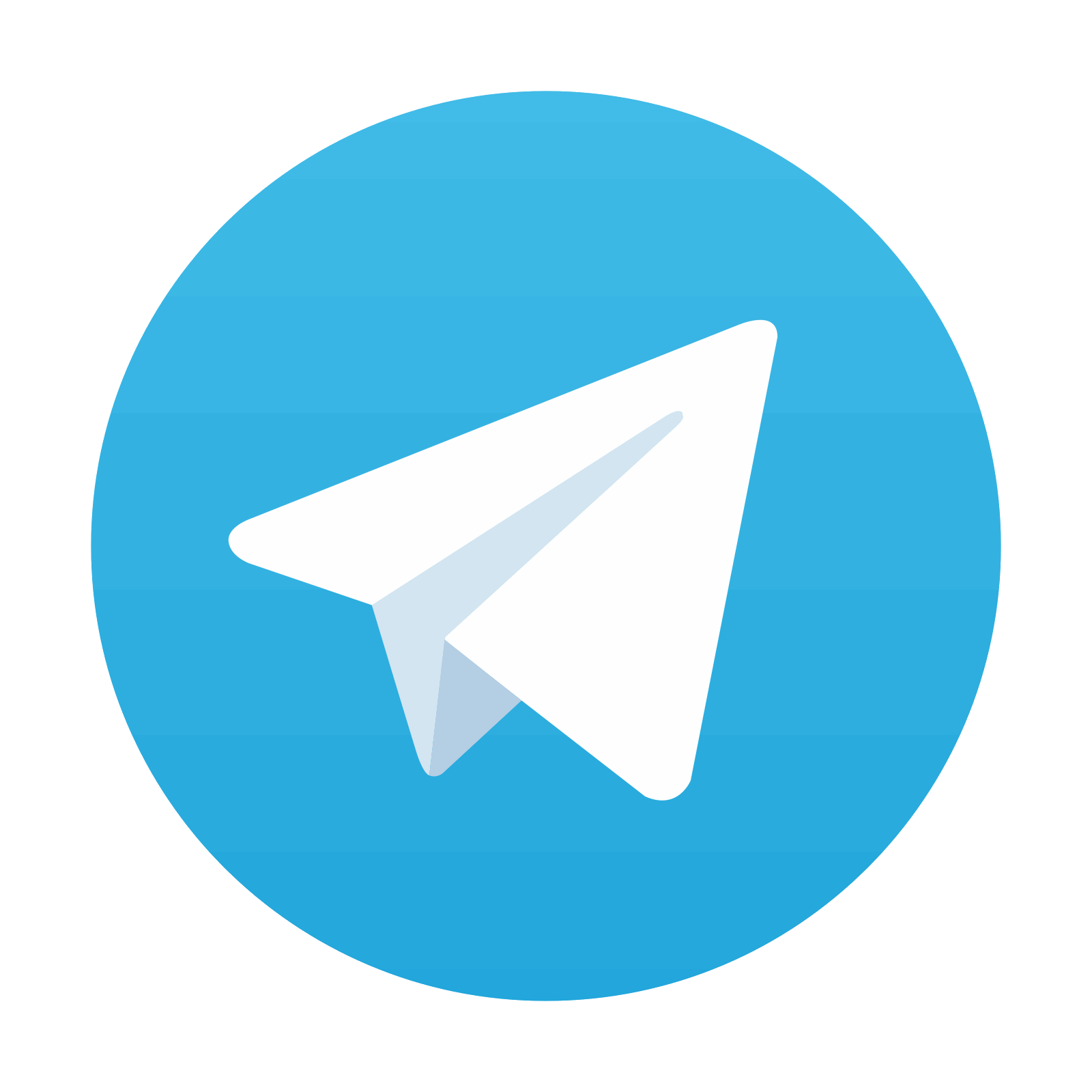
Stay updated, free articles. Join our Telegram channel

Full access? Get Clinical Tree
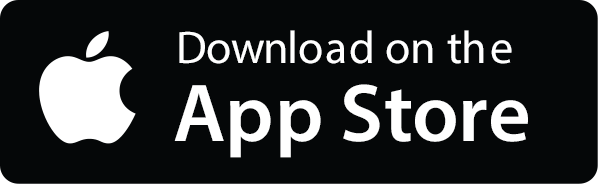
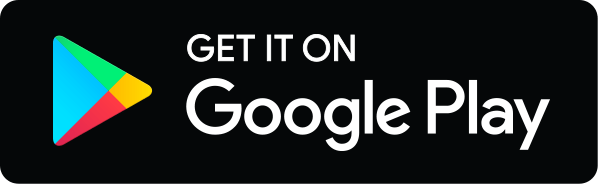