Disorders of Mitochondrial Fatty Acid Oxidation
Bryan E. Hainline
Rebecca S. Wappner
FATTY ACID METABOLISM
One of the primary functions of mitochondria, the aerobic production of energy from fatty acids, is discussed in this chapter. Other biochemical reactions taking place within these organelles are discussed in Chapters 384, 385, 386, 387 and 388 and 409. Mitochondria assume a wide variety of sizes and shapes that depend on the metabolic and physiologic needs of the tissue in which they are located. A prototypic mitochondrion is 1 to 2 μm long, is roughly cylindric, and has rounded ends. The outer surface is bounded by the outer membrane, which is permeable to low-molecular-weight compounds. The inner membrane is much more restrictive to biomolecules, however, and most substances cannot enter or leave the mitochondrial matrix unless a specific transport system is available. The inner membrane is folded narrowly into cristae at somewhat regular intervals, which project more or less deeply into the matrix. The narrow space between the outer and inner membranes (the intermembrane space) is continuous with the narrow space within the crista. In general, the areas at which the inner membrane is contiguous with the outer membrane primarily have transport functions, whereas those inner membrane areas that form the cristae have primarily electron transport–oxidative phosphorylation functions. The matrix has its own complement of enzymes that are involved with various metabolic functions and contains the mitochondrial DNA, whose genes encode RNA and additional molecules needed for protein synthesis and mitochondrial oxidative phosphorylation.
Mitochondrial beta-oxidation of fatty acids is an important source of energy for metabolic processes including normal fasting, muscular activity, illness with stress (e.g., infection), and nonshivering thermogenesis. After long-chain fatty acids are mobilized from adipose tissue, they are transported, bound to albumin, to the liver and other tissues, where plasma membrane uptake is mediated by fatty acid–binding proteins. Long-chain fatty acids are “activated” to their coenzyme A (CoA) esters by long-chain acyl-CoA synthase, are transported across the mitochondrial membrane by a carnitine-mediated system, and are oxidized to ketone bodies in the mitochondrial matrix. Medium-chain fatty acids are transported across the mitochondrial membranes in a noncarnitine-dependent process, activated by a corresponding matrix medium-chain acyl-CoA synthase, and they are then oxidized in a manner similar to the long-chain molecules. Disorders involving mitochondrial beta-oxidation of fatty acids are characterized by faulty ketone body formation, impaired energy production, and the accumulation of partially oxidized fatty acid metabolites during periods of stress and fasting. All are inherited as autosomal recessive traits. Biochemical aspects of these disorders, including the importance of carnitine, are described in Box 386.1. The disorders have widely varying clinical manifestations.
Dietary sources of carnitine include meats and dairy products. Endogenous carnitine may be synthesized from methylated lysine, which can be derived from such muscle proteins as myosin. Usually, secondary carnitine deficiency states are associated with organic acidemias or faulty beta-oxidation. Deficiencies may be seen also in patients with renal tubular disorders, vitamin C and pyridoxine deficiencies, strict vegetarian diets, total parenteral nutrition, hemodialysis, and valproate anticonvulsant therapy.
Systemic carnitine deficiency, regardless of its cause, results in faulty fatty acid oxidation and has been associated with poor tolerance of fasting, hypoketotic hypoglycemia, liver dysfunction, hypotonia, myopathies, cardiomyopathies, and recurrent myoglobinuria and myalgias.
DISORDERS OF FATTY ACID OXIDATION
The clinical features of disorders of fatty acid oxidation are summarized in Table 386.1. Additional details are provided in the following sections. It should be noted that the introduction of expanded newborn screening using tandem mass spectrometry will affect the identification and management of
these disorders. Although screening of asymptomatic newborns will identify most individuals with severe disorders, especially medium-chain acyl-CoA dehydrogenase (MCAD) deficiency, the remaining disorders may not be recognized early. Clinical suspicion should remain high when children or older patients present with symptoms described below, despite a history of a negative newborn acyl-carnitine screening test.
these disorders. Although screening of asymptomatic newborns will identify most individuals with severe disorders, especially medium-chain acyl-CoA dehydrogenase (MCAD) deficiency, the remaining disorders may not be recognized early. Clinical suspicion should remain high when children or older patients present with symptoms described below, despite a history of a negative newborn acyl-carnitine screening test.
BOX 386.1 Biochemical Aspects of Fatty Acid Metabolism
As shown in Figure 386.1, long-chain (C10 to C25) fatty acids are activated by long-chain acyl-CoA synthase (located in the outer mitochondrial membrane) to form long-chain acyl-CoA esters. Then the esters are transesterified with carnitine by carnitine palmitoyl transferase (CPT) I, which resides on the inner aspect of the outer mitochondrial membrane. The long-chain acyl-carnitines (and nonesterified carnitine) cross the inner mitochondrial membrane by a process mediated by carnitine-acyl-carnitine translocase. On the matrix side of the inner mitochondrial membrane, the long-chain acyl-carnitines are reesterified to long-chain acyl-CoA esters by a separate transferase, CPT II. The “activated” long-chain acyl-CoA esters then enter the beta-oxidation pathway. Medium-chain (C6 to C12) and short-chain (C4 and C6) fatty acids do not need carnitine-mediated transport to transverse the membranes. They are “activated” to their respective acyl-CoA esters by mediumor short-chain acyl-CoA synthases (synthetases) located in the mitochondrial matrix before entering the beta-oxidation pathway. With each cycle through the beta-oxidation pathway, the fatty acid–CoA ester is reduced in length by two carbons to form an acetyl-CoA group that can be metabolized further to ketone bodies in the liver and kidneys or can enter the tricarboxylic acid cycle in heart and skeletal muscle.
The beta-oxidation pathway includes a series of chain length–specific acyl-CoA dehydrogenases, enoyl-CoA hydratases, 3-hydroxyacyl-CoA dehydrogenases, and 3-oxoacyl-CoA thiolases. The four acyl-CoA dehydrogenases are flavoproteins (derived from riboflavin) that transfer electrons from acyl-CoA esters to electron transfer flavoprotein (ETF) and subsequently to the mitochondrial electron transport chain to form adenosine triphosphate. Very long-chain acyl-CoA dehydrogenase (VLCAD) is a membrane-bound protein that catalyzes the oxidation of fatty acids with chain lengths of 16 to 24 carbons; long-chain acyl-CoA dehydrogenase (LCAD) catalyzes the reaction for 12to 18-carbon fatty acid esters; medium-chain acyl-CoA dehydrogenase (MCAD) catalyzes the reaction for chain lengths of 4 to 14 carbons; and short-chain acyl-CoA dehydrogenase (SCAD) catalyzes the reaction for chain lengths of 4 or 6 carbons. Fatty acid acyl-CoA esters with odd chain lengths are oxidized similarly until a three-carbon propionyl-CoA is formed. Unsaturated fatty acids require additional enzymes, longand short-chain 3-cis, 2-trans-enoyl-CoA isomerase and 2,4-dienoyl-CoA reductase, for complete beta-oxidation of these compounds. An enzyme related to SCAD may be responsible for oxidation of branched-chain substrates. The long-chain activities for enoyl-CoA hydratase, 3-hydroxyacyl-CoA dehydrogenase, and 3-oxoacyl-CoA thiolase exist together in a trifunctional protein (TFP) complex. Individual soluble enzymes with short-chain enoyl-CoA hydratase, short-chain 3-hydroxyacyl-CoA dehydrogenase, and 3-oxoacyl-CoA thiolase activities have been found in the mitochondrial matrix.
The acetyl-CoA and acyl-CoA esters formed as a result of beta-oxidation exit the mitochondrial matrix by carnitine-mediated transport similar to that for the entrance of long-chain acyl-CoA esters. Acetyl-carnitine is formed by carnitine acetyltransferase and is transported by the translocase to the cytosol.
ETF and ETF:ubiquinone oxidoreductase (ETF:QO) are proteins that mediate the transfer of electrons from flavin-containing acyl-CoA dehydrogenases (i.e., VLCAD, LCAD, MCAD, SCAD, and others) to the main respiratory chain at the level of ubiquinone (coenzyme Q). The energy of these electrons is used for the formation of adenosine triphosphate and the ultimate reduction of molecular oxygen to form water.
L-Carnitine (gamma-trimethyl-beta-hydroxy-butyrobetaine) is needed for transport of long-chain fatty acid acyl-CoA esters into the mitochondrial matrix and for the return of acyl-CoA intermediates to the cytosol. L-Carnitine also functions to remove the metabolites of faulty beta-oxidation and certain other abnormal organic acids from the mitochondrial matrix by forming carnitine esters with these compounds. The abnormal acyl-carnitine compounds then are transported to the cytosol, from which they exit the cell, enter the circulation, and are excreted in the urine. Usually, plasma carnitine measurements are reported as total, free, and esterified in micromolar concentrations. In some patients with disorders of beta-oxidation or organic acidemias, both the total and free carnitine levels are low. In other patients, the total plasma carnitine level is normal, but the esterified fraction is abnormally high (greater than 40% of total carnitine). This condition results in a relative deficiency of free carnitine, which is needed for appropriate fatty acid transport.
Medium-Chain Acyl-CoA Dehydrogenase Deficiency
MCAD deficiency, the most common of the disorders of fatty acid oxidation, occurs in approximately 1 in 15,000 births, varying from 1 in 6,500 to 1 in 17,000 in different populations. This disorder is seen almost exclusively in whites, especially those with Northwestern European heritage, among whom the carrier rate may be as frequent as 1 in 40 for the most common mutation, K304E. Most patients present between ages 5 and 24 months with vomiting, lethargy, and hypotonia that occur after a decreased carbohydrate intake associated with an intercurrent illness or fasting. Mild hepatomegaly and seizures may occur. Hypoglycemia, mildly elevated blood ammonia, and increased liver enzyme levels usually are noted. Any acidosis usually is mild. Urine and plasma ketones may be either absent or present in trace amounts, which has led to the term hypoketotic hypoglycemia for this group of disorders. However, some patients may make ketone bodies when severely stressed. The amount of ketones produced in these patients, as determined by plasma beta-hydroxybutyrate levels, is inappropriately low for the degree of hypoglycemia and the marked elevation of
plasma free fatty acids. The pathophysiologic reason for the hypoglycemia is understood incompletely but is believed to result from failure of the normal increase in gluconeogenesis with fasting, which usually occurs in response to increased acetyl-CoA and ketone body production.
plasma free fatty acids. The pathophysiologic reason for the hypoglycemia is understood incompletely but is believed to result from failure of the normal increase in gluconeogenesis with fasting, which usually occurs in response to increased acetyl-CoA and ketone body production.
Clinical presentation varies widely. Some patients have been identified in the newborn period due to a history of early hypoglycemia, whereas others were asymptomatic and were detected only by family studies or state-mandated newborn screening. Ill, affected patients may have a rapidly deteriorating course with an episode that progresses to coma and death from cardiorespiratory collapse or cerebral edema. The accumulation of acyl-CoA compounds, especially those with chain lengths of three carbons or more, frequently is associated with encephalopathy and may result in cerebral edema. Mortality has been reported to be highest (59%) at between ages 15 and 26 months. Approximately 25% of patients die with their first episode, which frequently is unrecognized as being a result of a disorder of fatty acid oxidation until the disorder is diagnosed in a second child in the family. Many of the deaths have been attributed to Reye syndrome or to sudden infant death syndrome. Autopsy findings include fatty infiltration of the liver, the pattern of which may be macrovesicular or microvesicular. Mitochondrial changes in the liver on electron microscopy differ from those seen in Reye syndrome. Condensed mitochondria, with increased matrix density and intracristal widening, or enlarged and abnormally shaped mitochondria, with an increase in the number of cristae and crystalloids in the matrix, may be seen.
With episodes, affected individuals accumulate metabolites of medium-chain length (C6 to C12), especially octanoic acid and 4-decanoic acid. Owing to the excessive accumulation of fatty acyl-CoA intermediates of medium-chain length in the mitochondria, alternative pathways of microsomal (omega and omega1) oxidation and peroxisomal beta-oxidation become involved and lead to excessive production of (omega1)-hydroxy acids and medium-chain dicarboxylic acids, such as adipic, suberic, and sebacic acids. Organic acid analysis using gas or liquid chromatography–mass spectrometry may detect these metabolites in the blood or urine of patients during episodes. Acyl-CoA compounds may be conjugated with glycine or with carnitine. Abnormal metabolites of medium-chain length may be detected as urinary acyl-glycine conjugates (stable isotope dilution gas chromatography–mass spectrometry) or by plasma or dried blood filter-paper sample acyl-carnitine profiles (fast atom bombardment with tandem mass spectrometry). The latter two tests are more sophisticated and sensitive than are routine organic acid measurements. Their use is indicated particularly in the evaluation of all patients suspected of having a defect in fatty acid oxidation. Between episodes, asymptomatic affected patients may have normal routine organic acid studies. However, urine acyl-glycine or dried blood filter-paper or plasma acyl-carnitine profiles usually are abnormal. Frequently, affected patients have abnormal plasma and urinary carnitine levels, with lowered total and elevated esterified fractions. Such patients should not be subjected to a provocative fast because of the possibility of inducing a fatal acute episode. The use of acyl-carnitine profiles from dried blood filter-paper samples has not only allowed identification of asymptomatic affected children, but also has led to retrospective diagnosis in children who have died, including several affected newborns. Those infants identified by newborn screening tests should have the diagnosis confirmed by repeat testing at a separate standard laboratory. Confirmation of deficient activity of MCAD may be shown in cultured skin fibroblasts by finding disease-specific labeled metabolites using tandem or electrospray mass spectrometry assay after feeding with carbon 14– or deuterium-labeled palmitate or linoleate.
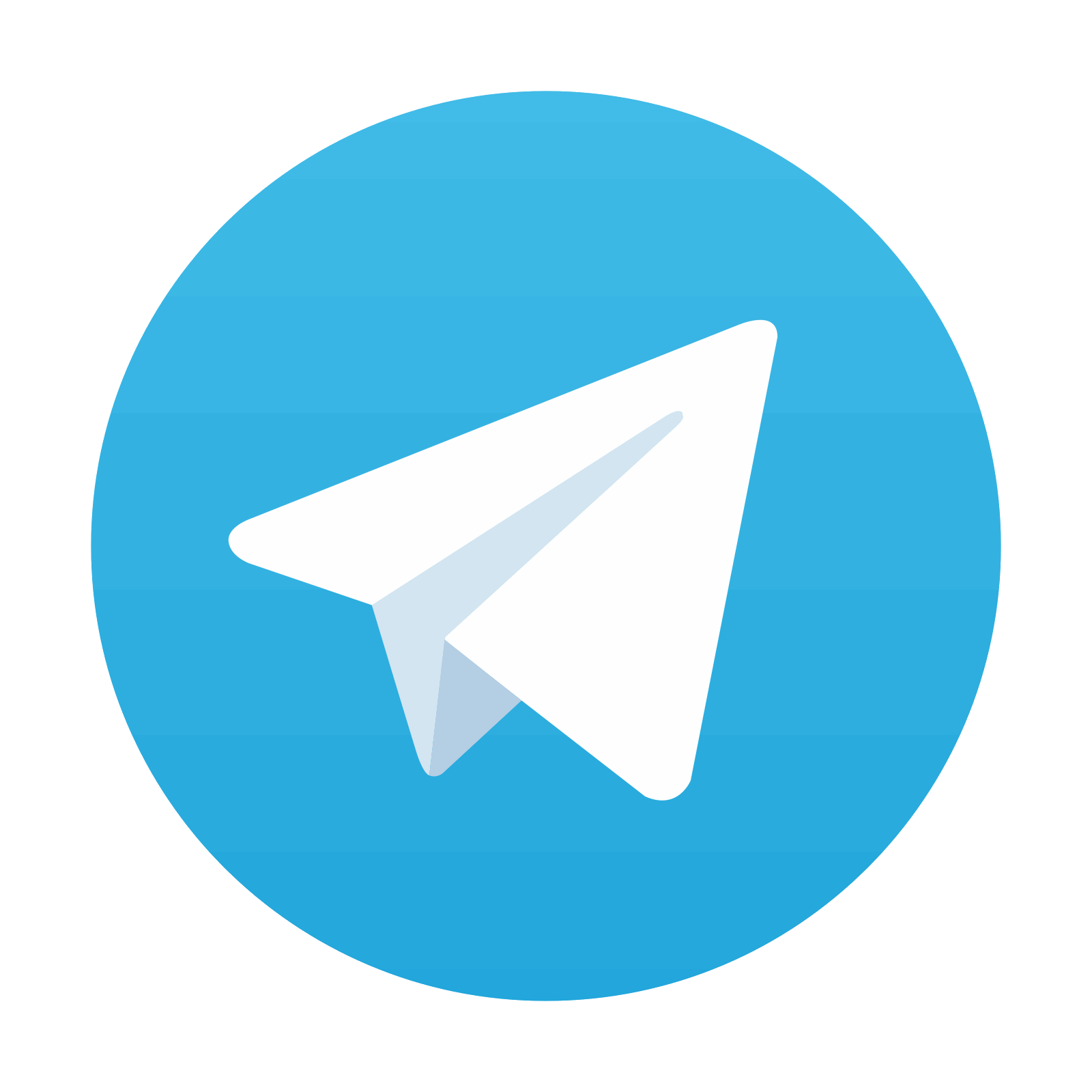
Stay updated, free articles. Join our Telegram channel

Full access? Get Clinical Tree
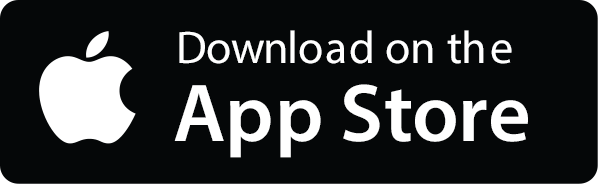
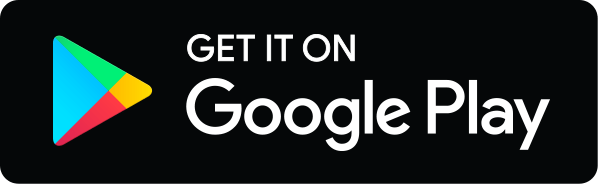