Fig. 25.1
(a) Mean hip flexion and knee flexion angles during sprinting. (b) Typical example of normalized EMGs for the biceps femoris long head (BFlh) and semitendinosus (ST) muscles during sprinting. The hamstring muscles were activated during the stance, mid-swing, and late-swing phases: the early-stance phase, beginning with the foot strike and ending with the maximum knee flexion during ground contact; late-stance phase, beginning with maximum knee flexion during the ground contact and ending with the toe-off; early-swing phase, beginning with toe off and ending with maximum knee flexion; mid-swing phase, beginning with maximum knee flexion and ending with maximal hip flexion; late-swing phase, beginning with maximum hip flexion and ending with foot strike. (c) Mean and standard deviation of the peak time, at which each RMS of the BFlh and ST reached their maximum value during the stance and swing phases. Statistically significant peak time differences between these two muscles were found: *, p < 0.05; **, p < 0.01
In a recent study we investigated the activation patterns of the lateral and medial hamstring muscles durning overground sprinting (Higashihara et al. 2013). The EMG activation of the ST muscle during the mid-swing phase was greater than that of the BFlh muscle (Fig. 25.2). In addition, the peak activation of the ST muscle occurred significantly earlier than that of the BFlh muscle during the swing phase (Fig. 25.1c). During the mid-swing phase, the biarticular hamstring muscles are stretched due to hip flexion and simultaneous knee extension. Studies using musculoskeletal simulation have shown that the biarticular hamstring muscles underwent lengthening contraction in this phase of sprinting and the ST muscle displayed the greatest musculotendon lengthening velocity among the hamstring muscles (Schache et al. 2012; Thelen et al. 2005; Yu et al. 2008). The ST muscle has long fibres that contain many sarcomeres in series, illustrating its potential to contract quickly over long distances (Heron and Richmond 1993). Therefore, the ST muscle is selectively recruited for controlling simultaneous knee extension and hip flexion because of its morphological ability to effectively handle strain during lengthening contractions. Furthermore, the activity of the ST muscle was significantly greater than that of the BFlh muscle during the late stance phase (Fig. 25.2), and the peak activation of the ST muscle occurrs significantly later than that of the BFlh muscle during the stance phase (Fig. 25.1c). An earlier study reported that an eccentric contraction of the hamstrings occurs during the late-stance phase of overground sprinting (Yu et al. 2008). Therefore, the ST muscle is selectively recruited for eccentric knee flexion during the late stance phase.
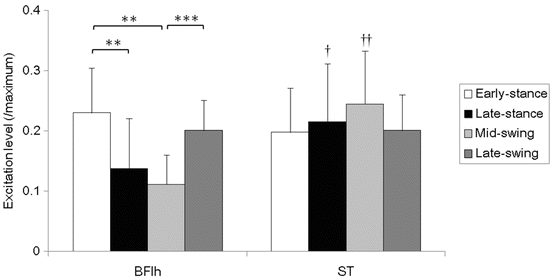
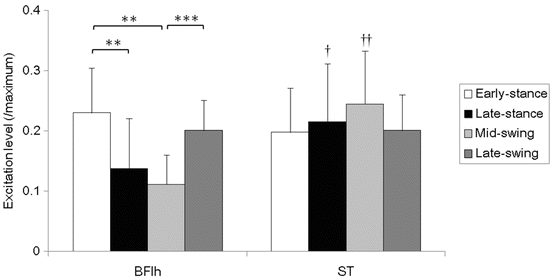
Fig. 25.2
Mean and standard deviation of the normalized EMG values during the early-stance, late-stance, mid-swing, and late-swing phases of sprinting. The two-way ANOVA indicated statistically significant interaction effects (muscle × phase; p < 0.001). The activation of the biceps femoris long head (BFlh) muscle during the early-stance and late-swing phases was significantly greater than during the mid-swing phase. The activation of the BFlh muscle during the early-stance phase was significantly greater than during the late-stance phase. The activity of the semitendinosus (ST) muscle was significantly greater than that of the BFlh muscle during the late-stance and mid-swing phases: **, p < 0.01; ***, p < 0.001; †, p < 0.05; ††, p < 0.01 vs. BFlh (Modified image from Conference Proceedings (Higashihara et al. 2013))
Activation of the BFlh muscle is greater during the early-stance and late-swing phases than during the late-stance and mid-swing phases, whereas the activity of the ST muscle exhibits no differences between each phase (Fig. 25.2) (Higashihara et al. 2013). In addition, peak activation of the BFlh muscle occurrs significantly closer to the point of foot contact compared to that of the ST muscle (Fig. 25.1c). Therefore, the activation demand of the BFlh muscle is high before and after foot contact. The hamstring muscles function as hip extensors that concentrically act to quickly swing the thigh back during the late-swing phase (Sugiura et al. 2008), and to provide the activation necessary for the generation of a forward propulsive force during the stance phase (Simonsen et al. 1985). In addition, the maximum torques for hip extension and knee flexion occur during foot contact in overground sprinting (Mann 1981). Therefore, during these phases of sprinting, the hamstring muscles primarily act as strong hip extensors (Novacheck 1998). One study that compared the recruitment patterns of the various hamstring muscles demonstrated that the BF muscle is selectively recruited during hip extension exercises because of the morphological potential for large torque production (Ono et al. 2011). In light of these previous investigations, the results of the present study indicate that the BF muscle is selectively recruited primarily as a strong hip extensor during the terminal swing and early stance phases.
25.2.3 Changes in the Activity of the Hamstring Muscles with Increasing Running Speed
Previous studies (Higashihara et al. 2010; Kyrolainen et al. 2005; Mero and Komi 1987; Schache et al. 2013) have examined activity of the lower extremity muscles during running at different speeds. Kyrolainen et al. (2005) found that the greatest change in the BF muscle activity pattern occurred as the speed increased from that of a slow jog to the maximum and indicated that increasing the running speed effectively requires increased EMG activity in the two-joint muscles during the entire running cycle.
We previously investigated activation patterns of the lateral and medial hamstring muscles during treadmill sprinting at different speeds and observed changes in activity patterns of the BFlh and ST muscles as the speed increased (Higashihara et al. 2010). There were no significant differences in the timing of peak activation of the BFlh and ST muscles at speeds below 95 % max. In contrast, at near maximum sprinting speed, peak activation of the BFlh muscle occurrs significantly closer to the point of foot contact as compared to that of the ST muscle. These findings indicate that a complex neuromuscular coordination pattern occurs during the running gait cycle at near maximum sprinting speeds. The increase to maximum speed is accomplished by utilizing each muscle according to its specific function.
When running at near maximum sprinting speed, the BFlh muscle is selectively recruited primarily as strong hip extensor because of the morphological potential for large torque production (see 25.2.2). The ground reaction forces at foot strike increase with increasing running speed (Kyrolainen et al. 2005; Mero and Komi 1987). Additionally, as running speed is increased, peak knee flexion torque during the terminal swing and peak hip extension torque during the terminal swing and initial stance are increased (Schache et al. 2011). Therefore, when running speed is increased toward the maximal level, the BFlh muscle strongly activates before and after foot strike to prepare for the intensive impact of the ground reaction force at foot strike, and also to play an important role in the generation of the forward propulsive force.
In contrast to the EMG activation of the BFlh muscle, peak activation of the ST muscle occurred significantly earlier than that of the BFlh muscle during the swing phase. During the mid-swing phase, the biarticular hamstring muscles are stretched, due to hip flexion along with simultaneous knee extension. Peak musculotendon lengthening velocities of the hamstrings occur at this phase and increase significantly with running speed (Schache et al. 2013; Thelen et al. 2005). In addition, lengthening velocity of the ST muscle is greater than that of the BFlh and SM muscles (Schache et al. 2012; Thelen et al. 2005; Yu et al. 2008). The ST muscle is therefore selectively recruited for simultaneously controlling knee extension and hip flexion because of its morphological property of effectively dealing with strain.
25.3 Insight into the Mechanism of Hamstring Strain Injuries During Sprinting
25.3.1 Mechanism of Hamstring Strain Injuries During Sprinting
It is well recognised that the hamstring muscles are susceptible to acute strain injury during high-speed sprinting (Askling et al. 2007). Several factors have been hypothesised to increase the risk for hamstring strains (Agre 1985; Hoskins and Pollard 2005). Commonly studied factors include hamstring muscle weakness (Sugiura et al. 2008), thigh muscle strength inbalance (Croisier et al. 2008; Sugiura et al. 2008), flexibility (Arnason et al. 2004; Heiderscheit et al. 2010), fatigue (Woods et al. 2004), and a history of previous injury (Croisier 2004). During sprinting, a complex neuromuscular coordination pattern in the hamstring muscles is accomplished according to each muscle’s specific functional characteristics. We, therefore, hypothesise that the disruption of the functional balance of the hamstring muscles, which may occur because of interactions between several of the risk factors, may lead to hamstring muscle strain (Fig. 25.3).
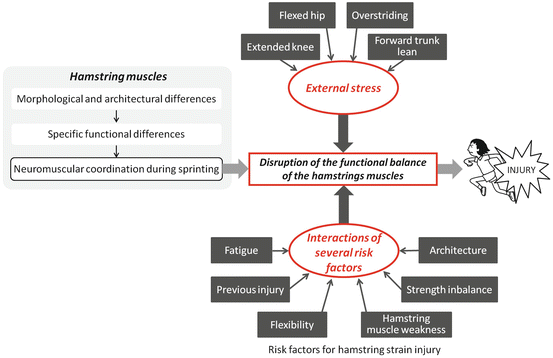
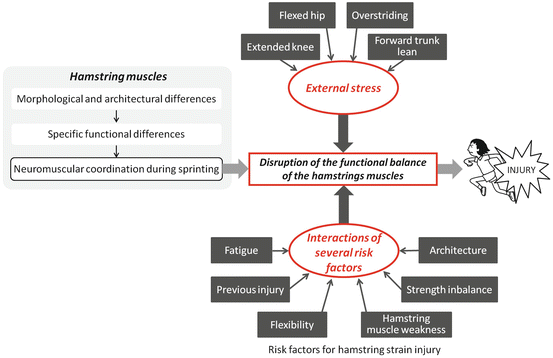
Fig. 25.3
One of the possible mechanisms of the hamstring strain injury which occurs during sprinting. During sprinting, a complex neuromuscular coordination pattern in the hamstring muscles would be accomplished by each muscle’s specific functional differences due to their morphological and architectural differences. When the hamstring strain injury occurs, the disruption of the functional balance of the hamstring muscles, which may occur because of interactions between several risk factors and/or the external stress, may lead to hamstring muscle strain
Furthermore, a hamstring strain is likely to occur during overstriding, i.e. when the foot lands too far in front of the centre of mass when running at close to the maximum speed and trying to maintain or achieve even greater speed (Orchard 2002). The BFlh muscle is selectively recruited during the late-swing and early-stance phases during which the hamstring muscles contract concentrically to produce hip extension torque. Therefore, we hypothesize that the risk of acute hamstring strain injury that involve the BFlh increases when the athletes have more forward trunk lean or flexed hip during these phases. On the other hand, the ST muscle is selectively recruited when the hamstring muscles contract eccentrically to control knee extension. Therefore, the risk of the injuries that involve the ST will increase when athletes have more knee extension during swing phase. These external stresses on hamstring muscles which are due to rapid intensive changes in movement also contribute to an increased risk of injury (Fig. 25.3).
25.3.2 Relation to the Location of the Hamstring Strain Injury
Previous magnetic resonance imaging studies of hamstring strain injuries (De Smet and Best 2000; Gibbs et al. 2004; Slavotinek et al. 2002) suggest that the BFlh muscle is the most likely to be injured; these injuries occur more commonly at the proximal end of the BFlh muscle and in combination with the adjacent ST. These results can be explained anatomically by the fact that in the proximal hamstring, the BFlh and ST muscles have a combined tendon origin and proximal part of their muscle (Gibbs et al. 2004). Considering the relations between the anatomical features of the proximal hamstrings and injury location, differences in the activation patterns between the BFlh and ST muscles, which were found in our previous studies (Higashihara et al. 2010; Higashihara et al. 2013), might have significant effects on the mechanism of hamstring strain. During sprinting at near maximum speed, a complex neuromuscular coordination pattern is accomplished according to each hamstring muscle’s specific functional differences. However, when the disruption of the functional balance of the lateral and medial hamstring muscles occurs due to the risk factors mentioned above, this may lead to an increase in load as well as force concentration. This combination could, result in increased risk for a hamstring strain injury. Future studies need to address interactions between the risk factors for hamstring strain injury (such as fatigue), as well as activation patterns of the specific involved muscles, to further explicate injury mechanisms.
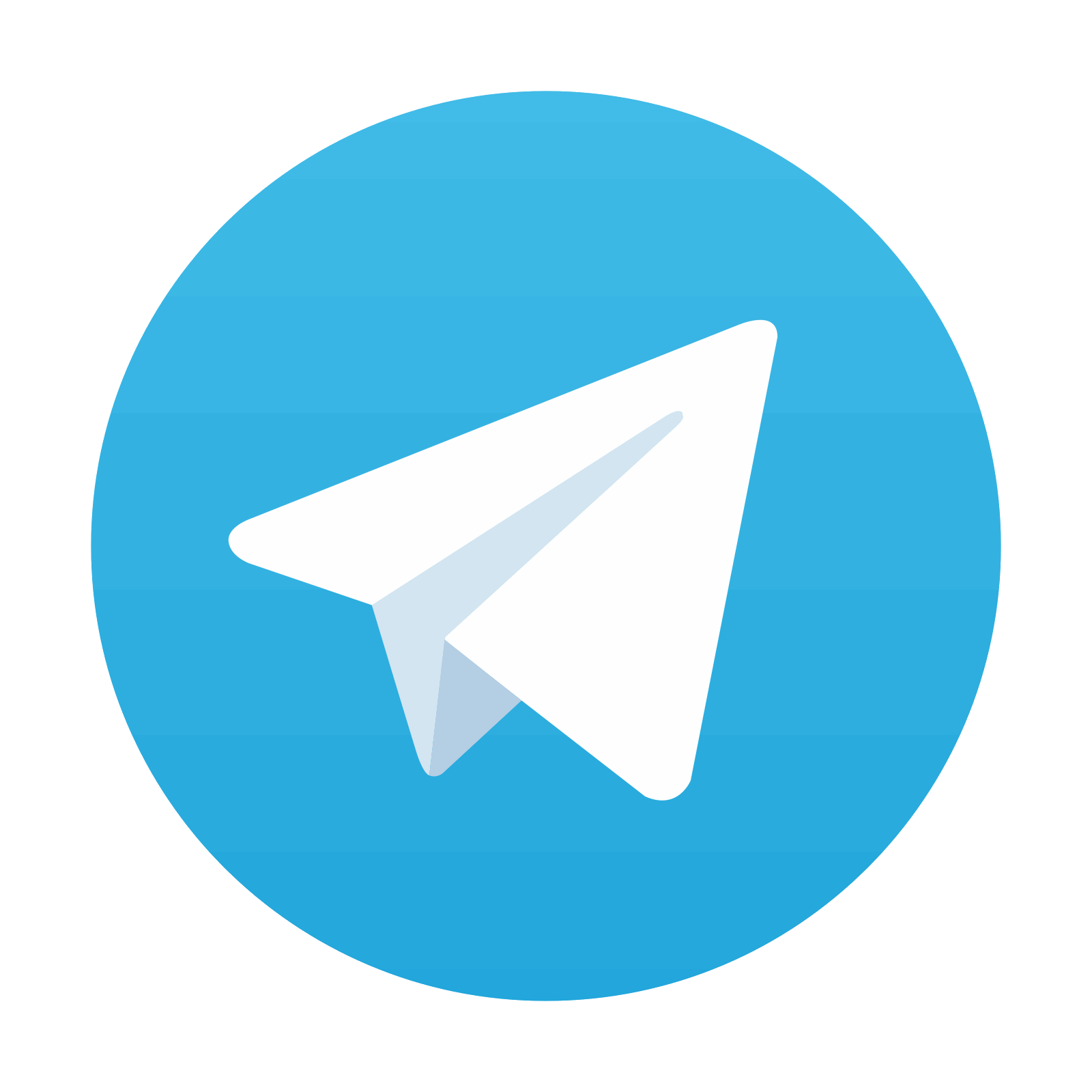
Stay updated, free articles. Join our Telegram channel

Full access? Get Clinical Tree
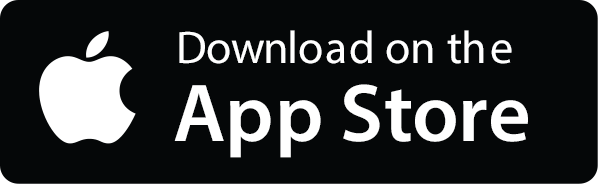
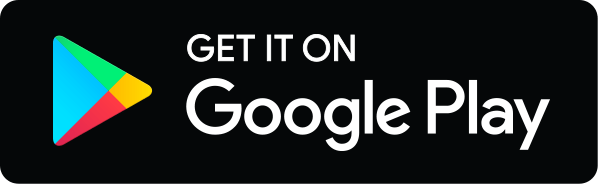