3 | Diagnosis of Osteomyelitis
Imaging Findings in Specific Disorders Nuclear Medicine Imaging of Bone Inflammations |
Radiologic Imaging
M. Kroetz, U. Linsenmaier, M. Reiser
Imaging Procedures
Conventional Radiography
Principle
Radiation from a point source passes through an object according to the principle of central projection. The x-rays are attenuated according to the properties of the irradiated material. A radiograph is created when the emerging x-rays strike a detector, which is usually a film-screen combination, digital storage screen or, more recently, a solid-state detector (Laubenberger and Laubenberger 1999).
Indications
Conventional radiographs have numerous indications, including the detection of osteolysis, osteonecrosis, and fractures; the identification of intra-articular loose bodies; the assessment of joint instabilities, dislocations, nonunions, degenerative changes, and reflex sympathetic dystrophy; and for evaluating fracture reduction and the placement of internal fixation devices (Reiser and Peters 1995, Freyschmidt 1997, Bohndorf and Imhof 2001).
Contraindications
There are no absolute contraindications to radiography, but the potential benefit should always be weighed against the risks of radiation exposure.
Pregnancy is a relative contraindication, and very rigorous criteria should be applied to patient selection. Magnetic resonance imaging (MRI) may be performed initially as an alternative to radiography.
Other relative contraindications are limited storage capacity and superimposed shadows from dressing materials, splints, fixation devices, or oral contrast medium following abdominal computed tomography (CT) or gastrointestinal radiography (Kauffmann and Rau 2001).
Indications for conventional radiography:
• Standard procedure for bone imaging when trauma, inflammation, or tumors are suspected
• Visualization of osteolysis, osteonecrosis, and demineralization
• Assessment of joint instabilities and dislocations and evaluation of reduced fractures
• Evaluation of bone healing, placement of internal fixation devices, and nonunions
• Detection of intra-articular loose bodies, reflex sympathetic dystrophy, and primary and secondary osteoarthritis
Contraindications to conventional radiography:
No absolute contraindications exist.
• Pregnancy (very strict selection criteria, especially in the first trimester)
• Children and adolescents (primary MRI may be considered as an alternative)
• Superimposed shadows from dressings, splints, fixation devices, and oral contrast medium
Quality Control
The German Medical Association has published quality control guidelines for conventional radiography (German Medical Association 1995). They include recommendations on exposure technique, film-screen systems, and radiation safety. Gridless projections without automatic exposure control, preferably with a 200-speed film-screen system, should be used for imaging the hand, ankle, and foot. Radiographs of the knee, hip, and axial skeleton should be performed on a Bucky table with grid using a 400-speed film-screen system. A leaded rubber shield should cover adjacent body regions while the films are exposed.
In pediatric examinations a 1-mm aluminum and 0.1-mm copper filter should additionally be used. Knee radiographs and other selected views may be taken without a grid, depending on the age of the child. Film-screen systems with a sensitivity of 400 or 800 are recommended for children. It should be noted that changing from 200 to 400 speed, for example, reduces the necessary radiation dose by half (German Medical Association 1995, Laubenberger and Laubenberger 1999).
Long Tubular Bones
Technique
Standard radiographic films of the long bones are taken in two mutually perpendicular planes. Images should include the adjacent joint to permit the accurate localization of abnormalities. Spot radiographs or fluoroscopy may be necessary for special investigations (Linsenmaier et al. 2000).
Interpretation
Surrounding soft tissues should always be evaluated along with the bony skeleton. Pay close attention to the contours and structure of the bones. Associated findings such as calcifications (e.g., vascular) and superimposed densities should also be assessed.
Interference Factors
Interpretation may be hampered due to difficulties of patient positioning or superimposed shadows from dressings, splints, or internal fixation devices.
Quality Control
Quality control includes checking the indication for the examination, the selection of a suitable imaging procedure, accurate collimation, and the centering of all films.
Use lead aprons to shield the rest of the body from exposure. This may include gonadal protection with a testis capsule in males and a lead shield over the ovaries in females (German Medical Association 1995).
Whenever possible remove dressings, splints and the like to eliminate superimposed shadows. It is best to take radiographs before dressing changes or before the application of a new splint or cast. If uncertainties arise, consult the person who ordered the radiograph prior to the examination (Linsenmaier et al. 2000).
Joints
The wrist, shoulder, elbow, hip, knee, and ankle joints are examined as described below.
Technique
As a rule, the joints should be imaged in two mutually perpendicular projections. If this is not possible due to adjacent body parts, as in the hip or shoulder region, the limb should be rotated 90° so that at least a portion of the joint—the femoral head or humeral head—will be at right angles to the initial plane (Linsenmaier et al. 2000, Bohndorf and Imhof 2001).
Interpretation
The articular surfaces, cortical and cancellous bone, marginal osteophytes, joint-space width, intra-articular calcifications, joint capsule, and tendons should all be evaluated (Dihlmann and Bandick 1995, Reiser and Peters 1995, Freyschmidt 1997, Bohndort and Imhof 1998, Greenspan 1999).
Interference Factors
Interpretation may be hampered due to difficulties of patient positioning or superimposed shadows from dressings, splints, or internal fixation devices
Quality Control
Key aspects in quality control are precise beam collimation and centering, plus radiation shielding for the rest of the body (see Long Tubular Bones, p. 16).
If interpretation is hampered by superimposed shadows or artifacts from internal fixation devices, spiral CT (SCT) or multidetector-row spiral CT (MSCT) may be considered. The image data can be processed to generate nonsuperimposed multiplanar reconstructions (MPRs) in any desired plane (Linsenmaier et al. 2000).
Cervical Spine
Technique
Biplane radiographs are obtained. To obtain nonsuperimposed views of the upper cervical spine in the anteroposterior (AP) projection, instruct the patient to move his or her lower jaw during the scan so that superimposed bone structures are blurred. Oblique views display the intervertebral foramina when the longitudinal body axis is rotated approximately 45 ° to the left and right. Here it is important to rotate the whole patient, not only the head, to enable examination of the entire cervical spine and intervertebral foramina. For functional imaging of the cervical spine, lateral flexion/extension views are obtained with the patient in the sitting or standing position.
A dens view is obtained by taking an AP projection through the open mouth.
Radiographs of the cervical spine are used to evaluate for degenerative and inflammatory changes; to evaluate the intervertebral foramina in neurologic disorders involving the upper half of the body, shoulders, and arms; and to check for segmental laxity and functional disorders of the cervical spine (Bohndort and Imhof 1998, Linsenmaier et al. 2000).
Interpretation
Pay close attention to the position of the cervical spine, the width of the intervertebral disk spaces, and the shape of the vertebral bodies. Degenerative changes are often manifested by thickening of the uncinate processes in uncovertebral arthrosis, marginal spurring in ankylosing spondylitis, intervertebral disk-space narrowing in osteochondrosis, and irregularities with increased facet-joint sclerosis in spondylarthrosis.
Inflammatory changes in rheumatic diseases are often manifested by alterations in the joints between the dens and atlas, the facet joints at the craniocervical junction, and the facet joints between the atlas and axis.
Malalignments and functional changes can be appreciated in functional views, paying particular attention to a reference line connecting the posterior margins of the vertebral bodies (Bohndorf and Imhof 2001, Dihlmann and Bandick 1995, Freyschmitt 1997, Greenspan 1999, Reiser and Peters 1995).
Interference Factors
If the patient is of heavy build or has restricted mobility, the lower segments of the cervical spine may be poorly visualized in the lateral projection because the shoulders are superimposed. This problem can be solved by drawing the shoulders downward and forward when the radiograph is taken (e.g., by placing weights in both hands).
Quality Control
Check the indication and necessary views (oblique views, functional views, special dens views). Protect the rest of the body from radiation exposure with a lead shield. Accurate beam collimation reduces scattered radiation and overall exposure (German Medical Association 1995).
Thoracic Spine
Technique
Biplane radiographs can be obtained with the patient lying down or standing upright in front of a wall cassette holder. Special oblique projections for defining the cervicothoracic junction (swimmer’s views) are rarely necessary.
Indications are degenerative and inflammatory diseases of the spine (Bohndorf and Imhof 1998, Linsenmaier et al. 2000).
Interpretation
Pay particular attention to the position, shape, and structure of the vertebral bodies and to the intervertebral disk spaces. Evaluate the facet joints with rib attachments, the pedicles, and the spinous processes.
Also take note of any calcification or ossification at the ligamentous attachments of individual vertebral bodies: spondylophytes, parasyndesmophytes, and bamboo spine in ankylosing spondylitis (Bandick 1995, Reiser and Peters 1995, Dihlmann and Freyschmidt 1997, Greenspan 1999, Bohndorf and Imhof 2001).
Interference Factors
See Cervical Spine, page 17.
Quality Control
Accurate beam collimation eliminates scattered radiation and reduces the radiation dose. Use a flexible compensating filter (German Medical Association 1995).
Lumbar Spine
Technique
Radiographs of the lumbar spine are taken in the recumbent position. Oblique views can also be obtained with the patient rotated approximately 45° to the right and left to assess the foramina and vertebral arches in spondylolisthesis and check for vertebral arch defects. Standing and functional flexion/extension views are added when evaluating patients for spondylolisthesis and segmental instabilities (Linsenmaier et al. 2000, Bohndorf and Imhof 2001).
Interpretation
See Thoracic Spine above. For functional imaging, also check for laxity and increased motility of individual vertebral segments that would indicate spondylolisthesis. The height of the intervertebral disk space provides evidence of disk pathology (Dihlmann and Bandick 1995, Reiser and Peters 1995, Freyschmidt 1997, Greenspan 1999, Bohndorf and Imhof 2001).
Interference Factors
Lateral projections of the lumbar spine require a high radiation dose due to the thickness of the interposed soft tissues. Thus, accurate positioning and collimation are particularly important for lateral lumbar views.
Quality Control
Accurate beam collimation is essential. Views at lower levels should include only a small portion of the sacrum. Recumbent views require the use of lead shielding for gonadal protection (German Medical Association 1995).
Standard Pelvic Radiograph
The standard pelvic radiograph consists of an AP projection in the supine patient.
Technique
The AP view should cover the entire pelvis. In examinations of the sacroiliac joint, the sacrum is imaged with the beam collimated on both sacroiliac joint lines (Lin-senmaier et al. 2000, Bohndorf and Imhof 2001).
Indications are degenerative and inflammatory diseases of the sacroiliac and hip joints. Pelvic radiographs are also used to screen for metastases and bone tumors.
Interpretation
Pay particular attention to bony contours and osseous structure. Other findings such as calcifications and super-imposed densities should also be evaluated (e. g., calcification of the iliac vessels (Dihlmann and Bandick 1995, Reiser and Peters 1995, Freyschmidt 1997, Greenspan 1999, Bohndorf and Imhof 2001).
Interference Factors
Image interpretation may be hampered by overlying bowel gas, which can mimic osteolytic lesions, and by superimposed oral contrast medium in the bowel.
Quality Control
Key aspects are accurate beam collimation and gonadal shielding (testis capsule for males and lead shield over the ovaries for females) (German Medical Association 1995).
Ultrasound
Principle
Ultrasound waves propagate through tissue by causing the tissue molecules to vibrate. The waves are partially or completely reflected at interfaces between different organs. The degree of reflection depends on the wave resistance or impedance of the particular organ (acoustic impedance = product of density times sound velocity). The propagation velocity of sound waves in tissue is relatively low, thus the different organs can be localized in relation to one another by measuring the transit time of the sound waves based on the time-distance principle (Laubenberger and Laubenberger 1999).
Technique
In the pulse-echo technique, ultrasound waves are transmitted into the tissue, and the time taken for the echo to return from an interface is measured (topographic mapping). This is achieved technically by utilizing the piezoelectric effect. When an alternating voltage is applied to ceramics or certain crystals, it alters the shape of the material at a microscopic level, giving rise to ultrasound waves. These piezoelectric materials can also receive reflected acoustic pressure waves and transform them back into electric energy. The frequency range of diagnostic ultrasound is between 2 and 30 MHz. The shorter the wave frequency, the higher the resolution and the smaller the depth of sound penetration. As the frequency increases, the waves penetrate more deeply but provide less resolution.
The basic principle of ultrasound imaging is to detect the sound waves reflected from interfaces (impedance) and process them into an ultrasound image corresponding to the structures encountered by the beam. Very dense tissue like bone reflects all of the incident sound. It appears hyperechoic (white) on the image display and obscures underlying structures. Less dense tissue appears hypoechoic (black) on the display. Sound waves pass completely through water and other fluids (transmission), which then appear hypoechoic (black) on the display while underlying structures appear hyperechoic in relation to the surrounding tissue.
Not all transmitted sound waves are reflected because they are subject to physical effects such as scatter, refraction, and absorption.
The returning sound waves detected by the transducer are electronically amplified. The echo return from a particular depth can be selectively amplified (time-gain compensation, TGC), or the entire image can be uniformly amplified (gain) (Laubenberger and Laubenberger 1999).
Indications
The main indication for ultrasound scanning in osteomyelitis is to detect and evaluate associated soft-tissue changes. A diffuse, edematous imbibition of the soft tissues surrounding the bone can be reliably diagnosed sonographically by comparing the left and right sides. Circumscribed fluid collections such as abscesses, hematomas, lymphoceles, or joint effusions can be clearly and completely visualized with ultrasound. A subperiosteal fluid collection may be detectable in acute osteomyelities, especially in children and adolescents (Heuck 1997, Bohndorf and Imhof 2001, Resnick 2002).
Indications for ultrasound:
• Visualization of soft-tissue edema
• Visualization of circumscribed fluid collections and abscesses
• Visualization of joint effusion
• Visualization of subperiosteal fluid
Contraindications
According to the findings of a World Health Organisation (WHO) Commission, the acoustic intensity of low-energy diagnostic ultrasound (10 mW/cm2) is harmless to biological tissues. Hence there are no contraindications to ultrasonography.
Interpretation
Ultrasound provides good soft-tissue contrast. Its spatial resolution is better than that of SCT. Another advantage is the ability to perform dynamic imaging. Muscles and tendons can be examined during motion, especially near joints. Cooperation from the patient, for example by indicating the most painful point in the region of interest (ROI) when transducer pressure is applied, can aid in the detection of pathology and shorten the examination time (Bohndorf and Imhof 2001, Resnick 2002).
Computed Tomography
Principle
Detectors measure the intensity loss in focused x-rays that pass through the patient from a radiograph tube rotating around the longitudinal body axis. Complex calculations yield a raw dataset, which is then computer processed to produce 2D sectional images (Laubenberger and Laubenberger 1999, Reiser et al. 2001).
Technique
Today only SCT should be used in the diagnosis of axial and peripheral skeletal changes. Thin slices (0.5-1.0 mm) should be used for the examination of joints, especially small ones. MPRs should be routinely obtained by reformatting the image date in sagittal and coronal planes. The original dataset is available for evaluating the axial plane. If necessary, sophisticated secondary reconstruction techniques can also be performed for more specialized studies.
The introduction of multidetector-row SCT technology has further advanced the diagnostic capabilities of CT. Since the scan time can be shortened to approximately one-eighth (4-slice CT) with otherwise equal scan parameters, motion artifacts are largely eliminated and slice thicknesses of 0.5-1.0 mm can be achieved. This generates near-isotropic volume elements (voxels) that greatly improve resolution along the longitudinal body axis (Z axis), while multiplanar (MPR) and 3D reconstructions (shaded surface display, virtual reality) can be produced with excellent image quality (Reiser et al. 2001).
IV contrast administration is recommended for the investigation of inflammatory soft-tissue processes, abscesses, and tumors
Interpretation
CT provides exceptionally high contrast resolution of bony structures and calcifications. Soft-tissue contrast is considerably higher than on conventional radiographs but lower than on MR images. CT can easily provide nonsuperimposed views of bones and joints, osteolytic lesions, bone fragments, sequestra, and fracture fragments.
Indication
The indications for skeletal imaging by CT include the visualization of bone fragments and sequestra; the determination of their number, size, and location; the evaluation of complex fractures; follow-up (especially of fracture consolidation); the detection of nonunions; and the detection or exclusion of osteonecrosis. All these indications coincide with the traditional indications for conventional tomography. In our view, conventional tomography should be performed only if a modern CT system is not available (Linsenmaier et al. 2000, Bohndorf and Imhof 2001).
Because MRI is more sensitive than CT, it is better for the detection of occult fractures and bone contusions and for assessing the viability of bone. CT is also becoming increasingly important in preoperative planning, especially for the acquisition of preoperative datasets for image-guided navigation and for postoperative follow-up (Linsenmaier et al. 2000, Bohndorf and Imhof 2001).
Indications for computed tomography:
• Visualization of bone fragments, sequestra, and intraarticular loose bodies
• Evaluation and follow-up of complex fractures (bone healing, fracture consolidation, nonunion, etc.)
• Preoperative planning, including determination of the number, size, and position of bone fragments
• Postoperative follow-up, including the position of fragments and internal fixation device
Contraindications
There are no absolute contraindications to CT, but the therapeutic benefit should always be weighed against the risks of radiation exposure. Pregnancy is a relative contraindication that calls for rigorous selection criteria. If necessary, primary investigation by MRI should be considered.
Quality Control
Positioning constraints are a limitation, especially if the patient cannot be positioned at the center of the gantry. Resulting image deficiencies can be partially offset by obtaining MPRs. Key elements are careful planning of the examination, careful patient positioning, and the possible need for limb fixation and padding.
Image quality may be degraded by metal implants. The more metal there is in the beam path, the greater the image distortion. Beam hardening, scattered radiation, absorption, and the resulting photon deficit can produce characteristic streak artifacts, registration errors, and deletions. Implants made of metal with a low atomic number, such as titanium and carbon, cause fewer artifacts than metals with a higher atomic number (steel). Various strategies are available for reducing metallic artifacts. Good practical solutions are to increase the window width (>3000 HU) and process very thin primary slices into secondary MPRs. Increasing the tube voltage or tube current is of no advantage (Link et al. 2000). Metal external fixation rods also cause artifacts. These devices may be removed before the examination or replaced by carbon rods. Plaster casts do not degrade image quality.
The examination protocol and scan volume should be based on clinical findings and conventional radiographic findings. As a general rule, CT and MRI should not be performed without first obtaining conventional radiographic films.
Magnetic Resonance Imaging
Principle
MRI generates sectional images of the body by utilizing the principle of nuclear magnetic resonance (Purcell and Bloch 1946). Thus this modality does not involve the use of ionizing radiation. The MRI of biological tissue was first performed in 1973 (Lauterbur, Mansfield, Grannell).
Technique
Atomic nuclei with an odd number of protons or neutrons have an angular momentum (nuclear spin) that is analogous to a spinning top. The angular momentum generates a magnetic moment that is directed along the rotational axis (dipole). This property is possessed by hydrogen nuclei, which are present in the human body in very high concentrations. Normally the rotational axes of the hydrogen nuclei in the human body are randomly distributed, so that the average of all the nuclear moments is equal to zero. But when the hydrogen nuclei are acted upon by a constant, strong external magnetic field, they will align themselves along the magnetic field lines because of their magnetic impulse and will acquire a measurable net magnetic moment. The aligned hydrogen nuclei “precess” in a spinning motion about the external magnetic field. The frequency of this spinning motion, called the Larmor frequency, is proportional to the strength of the external magnetic field and a nucleus-specific constant (gamma). When a high-frequency (HF) magnetic field is now applied perpendicular to the first magnetic field, the hydrogen nuclei will align perpendicular to the second HF field in an attempt to escape the applied force, and their rotational axes will move on a conical surface around the field lines of the initial magnetic field. All the hydrogen nuclei will undergo a synchronous precession immediately after the excitation pulse is applied, that is, they are in phase (phase coherence). If the frequency of the second HF magnetic field is equal to the specific precession frequency (resonance) induced by the initial constant magnetic field, the rotational axes of the hydrogen atoms can be deflected from their alignment by the low HF field strengths. (The resonance is specific for each type of nucleus.) If the second HF magnetic field is then turned off, the angle between the field lines of the outer magnetic field and the rotational axes will decrease, the longitudinal magnetization along the outer magnetic field increasing with the time constant T1 (longitudinal relaxation, spin-grid alternation), and energy will be emitted to the surroundings. At the same time, the phase coherence of the hydrogen atoms will decline steadily after the second magnetic field has been switched off. The hydrogen atoms’ precession is out of phase (dephasing), and the transverse magnetization component decreases until the magnetic resonance signal completely disappears. This time constant, called T2 relaxation, is caused by the reciprocal action of individual magnetic hydrogen atoms on one another (spin-spin interaction). In addition, dephasing is influenced by inhomogeneities in the external magnetic field (MR scan-ner, patient body, surroundings) so that the transverse relaxation does not decrease with the actual time constant T2 but decays more rapidly with the time constant T2*. The relaxation times describe the proton spins’ return to alignment parallel to the magnetic field due to their inter-actions with one another and their surroundings.
Since the external magnetic field is shielded to varying degrees by the electron clouds of the atoms and molecules in the chemical compounds, there are slight differences in the resonant frequencies (chemical shift) which depend on the body region being examined. By analyzing the frequencies (spectroscopy), the molecular structure can be evaluated, thus providing a mechanism for tissue discrimination. The greater the magnitude of the MR signal, the more protons are present in the ROI. In addition, the atomic nuclei return to their original positions at different rates, depending on their molecular structures (relaxation time).
The images produced by MRI reflect the density of the hydrogen atoms in the body and their temporal behavior when excited by electromagnetic radiation. “Gradient fields” are used for position encoding. These are additional magnetic fields that are superimposed over the constant external magnetic field in the three cardinal spatial directions.
Owing to the amplitude and duration of their voltage pulses, the HF magnetic fields used in MRI, known as radiofrequency fields, cause the hydrogen atoms to tilt from their initial alignment by 90° or 180°. Pulse sequences commonly used in MRI are spin-echo, inversion-recovery, and saturation-recovery sequences. Sequences with smaller flip angles, such as fast low-angle shot (FLASH) sequences, shorten the acquisition time. Faster sequences simultaneously register the entire signal from a relatively large body region and use a Fourier analysis to perform separation and spatial encoding of the frequency mix (2D and 3D Fourier image acquisition; Köchli and Marincek 1998, Laubenberger and Laubenberger 1999, Vahlensieck and Reiser 2002).
Contrast agents: Complexes that contain the chemical element gadolinium (Gd) are most commonly used as MRI contrast agents. Owing to its high number (seven) of unpaired electrons, Gd has a strong paramagnetic effect. In conventional doses (standard dose = 0.1-0.3 mmol/kg body weight), tissues with increased blood flow causing increased extravasation of Gd complexes show increased signal intensity due to shortening of the T1 relaxation time in the presence of the Gd contrast agent. Because Gd is toxic in its free-ion form, it is made into a complex by chelation, which yields a nontoxic, water-soluble Gd complex. The high stability of this complex ensures that free Gd ions are not released into the body in potentially toxic amounts. Patients with severe renal failure should undergo dialysis within 24 hours to prevent dissociation of the complexes. The effect of the chelate complexes on MRI signal characteristics is determined largely by the paramagnetic Gd, while the pharmacokinetic characteristics of the complex depend on the chelate-forming agents that are used. The pharmacokinetic behavior of extracellular Gd contrast agents is characterized by a rapid rise of plasma level after IV administration followed by a distribution phase in the extracellular fluid compartment that lasts only a few minutes. The fall in the plasma level (half-life of approximately 90 minutes) is determined by the renal excretion rate. The agents are excreted by glomerular filtration in a chemically unchanged form (Kochli and Marincek 1998, Laubenberger and Laubenberger 1999, Vahlensieck and Reiser 2002).
Interpretation
MRI is distinguished by its exceptional soft-tissue contrast and its sensitive detection of inflammatory changes. Images can be acquired in arbitrary planes of section. Active inflammatory processes can be distinguished from chronic processes on images acquired after IV contrast administration.
Indications
MRI is the modality of choice for imaging inflammatory changes in bones and soft tissues. High-resolution images can define the bone marrow, cortical bone, periosteal changes, and surrounding soft tissues.
Bone-marrow and soft-tissue inflammation are most reliably detected by unenhanced, water-sensitive (T2-weighted) sequences with or without fat suppression. Inflammatory changes show definite contrast enhancement in fat-suppressed T1-weighted sequences after IV Gd administration (Bohndorf and Imhof 2001, Vahlensieck and Reiser 2002).
Indications for MRI:
• Visualization of inflammatory changes in bone marrow, cortical bone, and surrounding soft tissues
• Visualization of periosteal reactions
• Visualization of inflammatory changes in bones and soft tissues
• Detection of circumscribed abscesses
Contraindications to MRI:
• Cardiac pacemaker (strong magnetic field)
• Relative: metal implants (intramedullary nails, total replacements)
• Relative: pregnancy
• Relative: prosthetic heart valves
• Relative: claustrophobia (patient may be sedated if necessary)
• Relative: severe obesity (Köchli and Marinek 1998, Laubenberger and Laubenberger 1999)
Quality Control
MRI should employ a high-field system with a magnetic field intensity of one Tesla or more. Sequences with frequency-selective fat saturation are best for imaging inflammatory changes (German Medical Association 2000).
Special Imaging Procedures
Fistulography
Principle
A radiographic contrast medium is injected to expand the fistulous tract and check for communication with surrounding structures.
Technique
Preparation: The procedure is performed under local anesthesia. Diagnostic preparations should include conventional skeletal radiographs and CT or MRI of the fistula region.
Fistula catheterization: A catheter is introduced into the fistula under sterile conditions, and an undiluted iodinated contrast medium is injected. The flow of the contrast medium is documented by fluoroscopy, and spot radiographs are taken in several different planes, preferably perpendicular to one another. The lumen of an adjacent hollow viscus can also be opacified with contrast medium if desired (Kauffmann and Rau 2001).
Interpretation
The entire fistulous tract should be evaluated from skin level to its termination. In certain cases, interpretation of the fistulograms should be aided by CT or MRI.
Indications
Preoperative fistulography is used for the investigation of inflammatory processes involving bones, joints, and soft tissues.
Contraindications
• Immunocompromised patient
• No therapeutic implications
• Contrast extravasation into blood vessels
• Contrast allergy
Interference Factors
Problems may arise due to superficial contrast leakage from the fistula opening, incomplete contrast filling of the fistulous tract, or the inoculation of infectious organisms into deeper tissues.
Quality Control
Mark the fistula opening with a safety pin. At least two radiographs are taken in mutually perpendicular projections. Superficial contrast leakage may lead to errors of interpretation.
Diagnostic and Interventional Angiography
Principle
The current preferred technique of diagnostic catheter-based angiography is intra-arterial digital subtraction angiography (DSA). Radiographic contrast medium is injected into the vascular ROI by selective or nonselective catheterization, depending on the indication.
Technique
Preparations include taking a history geared toward the planned intervention, securing informed consent, and excluding possible contraindications. Preliminary tests depend on the ROI and may include Doppler pressure measurement, duplex scans of the limbs, and CT or MR angiography (CTA, MRA).
Antegrade puncture of the common femoral artery is performed if a simultaneous interventional procedure is planned. This requires initial MRA or CTA evaluation of the corresponding vascular region. If a stenosis is found that can be treated interventionally, this can be done in the same sitting (Kandarpa 1996, Castaneda-Zuniga 1997).
Indications
Angiography is particularly rewarding in patients with delayed wound or bone healing that have been selected for plastic reconstructive surgery. The vascular status of the affected region should first be investigated to confirm adequate blood flow.
Indications for DSA include primary vascular diseases such as arterial occlusive disease, arteriovenous malformations, aneurysms, arteriovenous fistulas, traumatic vascular injuries, vasospasm, and segmental vascular diseases. Other indications are the diagnosis of secondary vascular diseases such as vascular compression or infiltration, postoperative or postinterventional complications, and the assessment of preoperative or preinterventional vascular anatomy. If a vascular stenosis is suspected or has been confirmed, preparations should be made for an interventional procedure and DSA should be combined with percutaneous transluminal angioplasty (PTA), stent insertion, and thrombolysis.
Contraindications
Absolute contraindications to DSA include unstable patients who require preliminary treatment elsewhere, uncorrected organ failure, and indeterminate tumor masses before exclusion of an iodine-storing thyroid carcinoma.
Relative contraindications include severe previous contrast reactions, impaired renal function, equivocal thyroid function, recent myocardial infarction, severe arrhythmia, severe heart failure, coagulopathy with a markedly increased bleeding risk, limited ability to assume a recumbent position, and cardiopulmonary diseases.
General contraindications to radiographic examinations include pregnancy, superimposed oral barium contrast medium, and previous abdominal CT, upper GI series, or contrast enema (Günther and Thelen 1996, Kandarpa 1996).
Precautions in Specific Diseases
Possible precautionary measures include hydration, thyroid blockade, and sedation.
Diabetes: In consultation with the physician in charge, the insulin dose can be reduced by 50% and the examination scheduled for late morning. Antidiabetic medication with metformin should be discontinued 2–3 days before the administration of iodinated contrast media. The patient should be adequately hydrated due to the increased risk of tubular necrosis.
Thrombocytopenia: The current value should exceed 75 000 platelets/mL Check blood count and coagulation status before the intervention.
Anticoagulated patients: Coumarins should be discontinued several days before the examination (Quick > 50%), and heparin may be substituted as required. In an emergency, give the patient fresh-frozen plasma or vitamin K (25-50 mg) at least 4 hours before the procedure.
Heparinized patients: Discontinue heparin at least 3-4 hours before the examination (PTT values < 1.25-1.5 times control values). Heparin can be resumed 6-8 hours after the examination or earlier in selected cases, although this will increase the bleeding risk.
Impaired renal function: Known risk factors for developing renal failure after contrast administration are patient age, hypertension, administered contrast dose, and preexisting proteinemia. Serum creatinine levels generally return to normal within 5-10 days after contrast administration.
Pheochromocytoma: Up to 8% of pheochromocytoma patients experience a hypertensive crisis during the procedure.
Polycytemia vera and sickle-cell anemia: Thromboembolic complications may occur after contrast administration.
Agitation and nervousness: Sedate unusually anxious patients for the examination (Günther and Thelen 1996, Kandarpa 1996).
Quality Control
On completion of the examination, place compression on the arterial puncture site for 8-10 minutes. Then apply a pressure dressing and place a sandbag over the groin for at least 2 hours. If intravascular heparin has been administered, it may be necessary to remove the sheath in two stages, and bed rest should be maintained.
Angiographic examinations via a femoral approach have a reported complication rate of 1.73 %; 0.4% of which are vascular injuries and 0.26% are postprocedure bleeding (Kandarpa 1996).
Ambulatory Angiography
Ambulatory examinations can be scheduled for all cooperative patients who are able to recognize complications after completion of the procedure. Prerequisite: Another adult must be available to watch the patient.
Exclusion criteria: a history of severe allergies or a severe prior contrast reaction, heart failure, renal failure, poorly controlled hypertension, anticoagulant therapy in patients with a coagulopathy or electrolyte disorder.
The patient should be monitored for at least 2 hours after the examination, and fluid intake should be sufficient to ensure rapid elimination of the contrast medium (Günther and Thelen 1996, Kandarpa 1996).
Percutaneous Drainage of Joints, Bones, and Abscesses
Principle
Percutaneous aspiration of fluid collections, tumors, or abscesses is performed under CT or ultrasound guidance for diagnosis (e.g., bacteriology, histopathology) or treatment (external drainage).
Technique
Preparations: The procedure is performed under local anesthesia or under IV sedation if required. Diagnostic preparations include ultrasound, CT, or MRI of the affected skeletal region for accurate localization.
Puncture: Deep local anesthesia may be administered along the proposed needle track, depending on the location. Special coaxial needles or other biopsy needles can be used to retrieve tissue samples. Stellate biopsies can be repeatedly taken through an outer guide sheath to avoid cell dissemination along the puncture track. When dealing with an abscess or predominantly fluid lesion, first take material for bacteriologic or cytologic analysis and then introduce a 5F to 8F ring or pigtail catheter over a guidewire to drain the contents.
Interpretation
An adequate amount of biopsy material can be sampled in 80%-95% of cases. It is best to biopsy the periphery of large lesions because central portions may already be necrotic. The percutaneous drainage of abdominal abscesses is successful in 70%-90% of cases, eliminating the need for operative treatment. Comparable data are not available for skeletal interventions (Günther and Thelen 1996, Kandarpa 1996).
Indications
Indications include the drainage of uninfected fluid collections such as pseudocysts and lymphoceles, the drainage of abscesses, the sampling of bacteriologic material, and biopsies to confirm the diagnosis of primary or secondary tumors.
Contraindications
Untreated coagulopathy and technical obstacles in cases where the tumor is not accessible.
Interference Factors
Complications arise in 5%-10% of all imaging-guided biopsies and abscess-drainage procedures, depending on the institution. Complications may include infection, sepsis, bleeding, pneumothorax, and local infection at the puncture site.
Less than 2% of diagnostic aspirations and biopsies are complicated by infection, bleeding, organ injury, pneumothorax, or pancreatitis. The seeding of malignant cells along the needle track has been described in case reports but is generally regarded as extremely rare (<0.01%; Günther and Thelen 1996).
Quality Control
Laboratory values: platelets > 75 000, Quick > 60 %, PTT at least 1.5 times the control value. Supplement local anesthesia by analgesia in certain procedures, especially when the efficacy of local anesthesia is compromised in the infected area. Rigorous asepsis should be practiced during biopsy or drainage. An adequate diagnostic work up (MRI, CT, ultrasound) is essential. Easily accessible lesions can be biopsied or drained at bedside under ultrasound guidance. Dressings should be regularly changed and drains irrigated with 3 × 5-10 mL of sterile saline solution. Drains may be left indwelling for 2-7 days. If drainage suddenly stops, check the drain placement and take samples for bacteriologic testing before removing the drain.
Imaging Findings in Specific Disorders
M. Kroetz, U. Linsenmaier, M. Reiser
Acute and Chronic Bone Infections
Classification of Osteomyelitis
Acute Hematogenous Osteomyelitis
Sites of Occurrence and Clinical Features
Acute hematogenous osteomyelitis occurs predominantly in early childhood (see Table 3.1 for classification of different forms of osteomyelitis). The incidence rises again after 50 years of age. Infantile hematogenous osteomyelitis is distinguished from acute juvenile osteomyelitis and acute adult osteomyelitis (Heuck 1997, Bohndorf and Imhof 2001, Resnick 2002, Vahlensieck and Reiser 2002).
Infantile osteomyelitis (up to age 12 months) is an acutely progressive disease that often shows multicentric and articular involvement. Sites of predilection are the femoral metaphyses, hips, and humerus. The diaphyseal, metaphyseal and epiphyseal blood vessels create potential pathways for epiphyseal and subperiosteal spread (Fig. 3.1). The main causative organisms are streptococci. Males and females are affected equally (Cohen et al. 1990, Heuck 1997, Resnick 2002).
Table 3.1 Classification of different forms of osteomyelitis (modified from Bohndorf)
Acute osteomyelitis | Chronic osteomyelitis | Special forms |
---|---|---|
Hematogenous | Endogenous/primary hematogenous | Brodie abscess Tuberculous osteomyelitis |
Recurrent multifocal | Plasma cell osteomyelitis | |
Salmonella, fungal infections | ||
Exogenous/secondary | Others |
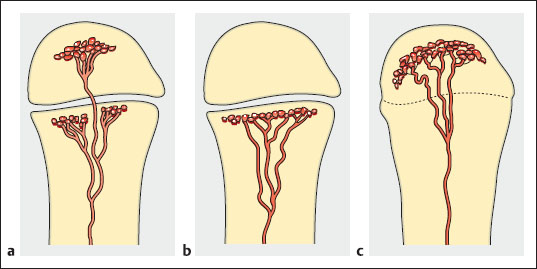
Fig. 3.1a-c Metaphyseal and epiphyseal blood supply. During the first year of life, the vessels cross the epiphyseal plate (a). From approximately 2 to 16 years of age, the vessels become obliterated and the epiphysis forms a barrier to hematogenous spread (b). By skeletal maturity, however, blood vessels again establish a connection between the epiphysis and metaphysis (c).
Juvenile osteomyelitis (12 months or older) is characterized by an acute onset of symptoms that are poorly localized initially. The metaphyses of the long tubular bones are most commonly affected. Usually the infection does not spread to the epiphysis due to physiologic occlusion of the meta- and epiphyseal vessels. Intra-articular spread may occur only if the joint capsule encloses portions of the metaphysis (hip, knee). Subperiosteal spread may also occur. The main causative organisms are staphylococci, and males are predominantly affected (Cohen et al. 1990, Heuck 1997, Resnick 2002).
Acute adult osteomyelitis: This is a rare disease that often takes an insidious clinical course. Sites of predilection are the axial skeleton and long tubular bones, and the diaphysis, metaphysis, and epiphysis may be involved. Articular involvement is common. Subperiosteal involvement is rare due to the thick cortex and firm periosteal attachment in adults. If subperiosteal spread does occur, it often leads to fistula formation and soft-tissue involvement. Males are predominantly affected (Heuck 1997, Bohndorf and Imhof 2001, Resnick 2002, Vahlensieck and Reiser 2002).
Chronic Hematogenous Osteomyelitis
If acute hematogenous osteomyelitis does not resolve, it may pass through a subacute stage before progressing to chronic hematogenous osteomyelitis, which is sometimes multifocal (Resnick and Petterson 1992, Heuck 1997, Bohndorf and Imhof 2001, Resnick 2002). Key imaging goals are the early detection of a reactivated, florid inflammatory process and the detection or exclusion of new inflammatory foci. This is most effectively accomplished by MRI.
Differential diagnosis of hematogenous osteomyelitis:
• Eosinophilic granuloma
• Malignant tumors: Ewing sarcoma, osteosarcoma, reticular cell sarcoma
• Necrotic tumors, posttraumatic seromas
• Abscesses due to other causes
• Periostitis due to other causes
• Noninfectious osteomyelitis (psoriasis, Reiter syndrome)
Imaging of Acute and Chronic Hematogenous Osteomyelitis
Projection Radiography
Hematogenous osteomyelitis produces radiographically detectable bone changes by 7-14 days at the earliest. Trabecular bones at that time may show ill-defined margins with a geographic, moth-eaten or permeative pattern of bone destruction. The lesion margins are indistinct. The cortex is initially eroded from the inside before varying degrees of cortical destruction can be seen. Note that destructive changes are more difficult to detect in trabecular bone than in compact bone. The infection may spread outward through the cortical bone, elevating the periosteum and causing radiographically detectable periosteal new bone formation. If the spread of infection deprives bony areas of their blood supply, these areas will become necrotic and will eventually appear on radiographs as sequestra. Reactive new bone formation may completely isolate the sequestrum from surrounding bone (involucrum). Marked associated soft-tissue swelling, especially about joints, will often develop within 3-5 days in infants and small children.
NOTE
Conventional radiographs initially show obliteration of fat planes and increased soft-tissue density due to edema of the paraosseous soft tissues.
The bony changes that occur during the reparative stage and in chronic hematogenous osteomyelitis depend on the virulence of the causative organism, host immune status, and treatment. Endosteal, cortical, and periosteal new bone formation produce a mixed lytic and sclerotic pattern. The initial sign of healing is centripetal sclerosis (Gold et al. 1991, Heuck 1997, Bohndorf and Imhof 2001, Resnick 2002).
NOTE
Chronic hematogenous osteomyelitis in children may resolve completely, or there may be persistent sequelae with partial destruction of the metaphysis and, less commonly, of the epiphysis (growth disturbance). Complete resolution does not occur in adults, and it is likely that a sequestrum will be formed.
Key radiographic features of hematogenous osteomyelitis:
• Detectable soft-tissue swelling during the first 3 days
• Bony changes no earlier than 7-14 days
• Trabecular bone destruction (geographic, moth-eaten, permeative)
• Ill-defined lesion margins with convex cortical thinning
• Periosteal new bone formation
• Detectable sequestrum or involucrum (rare)
• Mixed lytic and sclerotic changes in the reparative stage
Ultrasound
The value of ultrasound in acute osteomyelitis is inversely proportional to patient age. In infants, the early phase of osteomyelitis is initially marked by edematous swelling of the deeper soft tissues. This is followed later by the appearance of a thin, echo-free subperiosteal fluid collection that visibly elevates the periosteum. The significance of ultrasound findings should not be overstated, however. The changes described above are nonspecific, and there is a substantial incidence of false-positive findings and (in up to 40% of cases) false-negative findings (Kaiser and Rosenborg 1994).
Ultrasound scans in adults can detect only associated soft-tissue involvement. Abscesses and hematomas appear as echo-free or hypoechoic masses. Diffuse soft-tissue edema leads to inhomogeneous, hyperechoic thickening of the subcutaneous tissue. Ultrasound is very accurate in the detection of joint effusions (anechoic intraarticular fluid). Usually it cannot be determined whether a bland or suppurative effusion is present.
Computed Tomography
MRI is superior to CT owing to its good soft-tissue contrast and high accuracy, especially in infants and children due to the scant fat planes in that population.
NOTE
CT has a minor role in pediatric cases due to its high radiation exposure.
CT scanning in adults can accurately detect the destruction of trabecular and cortical bone, periosteal ossification, sclerosis, and sequestration. Bone density is increased, the density difference being most pronounced in the diaphysis (fat marrow). Gas inclusions and fat-fluid levels in the medullary cavity are relatively specific for osteomyelitis. Paraosseous abscesses can be identified by their fluid attenuation and enhancing abscess membrane. Surrounding soft tissues show an obliteration of interposed fat planes due to edema. CT findings and conventional x-ray findings coincide during the repair stage and in chronic hematogenous osteomyelitis. Both modalities show osteolytic lesions coexisting with sclerotic changes (Gold et al. 1991, Bohndorf and Imhof 2001, Reiser et al. 2001, Resnick 2002).
Magnetic Resonance Imaging
NOTE
MRI permits the early diagnosis of inflammatory bone and soft-tissue changes owing to its high tissue contrast.
Frequency-selective and T-weighted MRI pulse sequences (STIR = short true inversion recovery) combined with fat suppression can detect inflammatory changes with high sensitivity, although they tend to overestimate the spatial extent of the inflammation. Decreased signal intensity is seen in the bone marrow and fatty tissue in T1-weighted sequences. This technique also provides detailed anatomical visualization. T2-weighted images without fat saturation show little or no contrast between inflammatory changes and normal bone marrow or fat, especially when fast (turbo) spin-echo sequences are used. Inflammation and edema in muscles appear hyperintense in T2-weighted images. The same is true for contrast-enhanced T1-weighted sequences. Contrast-enhanced T1-weighted sequences with fat suppression are particularly rewarding. Inflammatory areas in the bone marrow and in paraosseous soft tissues are delineated by their high signal intensity. An abscess membrane surrounding a fluid-filled abscess cavity can easily be identified. Sequestra are also nonenhancing but, like abscesses, are hypointense in the STIR sequence and may show little if any peripheral enhancement. If the inflammatory process spreads to the cortex, increased signal intensity is visible in the compact bone, which is normally devoid of signal. Periosteal reactions can also be identified. Fistulous tracts have fluid-equivalent signal intensity in T2-weighted and STIR sequences, and the tract walls show definite contrast enhancement in T1-weighted sequences.
The healing stage of acute osteomyelitis is marked by a regression of edematous changes (decreasing signal intensity in the STIR sequence) and soft-tissue swelling. A fibrovascular halo forms, which enhances initially and then shows a steady decline in contrast uptake.
In chronic hematogenous osteomyelitis, MRI is the imaging modality of choice for detecting new inflammatory foci, which show the same signal and enhancement characteristics as acute hematogenous osteomyelitic lesions. Sequestra are devitalized bone fragments that appear as signal voids surrounded by granulation tissue or pus (Quinn et al. 1988, Ungeretal. 1988, Goldetal. 1991, Zynamon et al. 1991, Jones et al. 1992, Morrison et al. 1993, Vahlensieck and Reiser 2002).
NOTE
MRI has significantly higher specificity than scintigraphy and equivalent sensitivity. However, it is difficult for MRI to distinguish between active inflammatory tissue and reactive edema, between active and inactive inflammation in chronic osteomyelitis, and among periostitis, osteomyelitis, and reactive bone edema associated with a primary soft-tissue inflammation.
Key MRI findings in hematogenous osteomyelitis:
• Early detection of inflammatory changes in bone marrow edema (STIR, T1-weighted sequences)
• Better differentiation of tumors after contrast administration
• Better differentiation from abscess, abscess membrane, and perifocal inflammatory reaction after contrast administration
• Visualization of fistulous tracts and inflammatory soft-tissue involvement
• Detection of sequestra
• The activity of a lesion during the repair phase can be evaluated based on edema resolution and enhancement characteristics
• Equivalent sensitivity and higher specificity than scintigraphy (Figs. 3.2 and 3.3)
Complications of acute hematogenous osteomyelitis:
• Recurrences, especially in juveniles and even after several years
• Multifocal occurrence, especially in infants
• Bone necrosis, sequestrum, involucrum
• Subperiosteal abscess, cellulitis
• Fistula formation
• Longitudinal growth disturbance due to epiphyseal involvement (infantile osteomyelitis)
• Pathologic fractures
Chronic Recurrent Multifocal Osteomyelitis
Etiology, Sites of Occurrence, and Clinical Features
Chronic recurrent multifocal osteomyelitis (CRMO) occurs predominantly in children, adolescents, and young adults (principal manifestations between 5 and 15 years of age), with a preponderance of females. Sites of predilection are the metaphyses of the leg bones and the middle third of the clavicle, but any bones may be affected, including vertebrae. The disease begins with a synchronous or metachronous appearance of circumscribed, multifocal bone lesions. This is accompanied by focal, painful soft-tissue swelling with no systemic manifestations. The disease takes an undulating course with exacerbations and remissions that may last for up to 15 years.
NOTE
In most cases a causative organism cannot be identified and the etiology of the disease remains unclear.
A connection with pustulotic arthro-osteomyelitis (SA-PHO [synovitis, acne, pustulosis, hyperostosis, and osteomyelitis] syndrome) has been postulated (Sundaram et al. 1996, Jurik and Egund 1997, Bohndorf and Imhof 2001, Resnick 2002).
Differential diagnosis of CRMO:
• Osteomyelitis due to other causes
• Paget disease
• Fibrous dysplasia
• Sarcoma
• Bone infarction
Imaging of Chronic Recurrent Multifocal Osteomyelitis
Projection Radiography
CRMO is characterized by pronounced bone scleroses with associated periostitis. If the course has been short (<3 weeks), osteolytic lesions may predominate. With a protracted course, radiographs may show osteolytic lesions with sclerotic margins, lytic-blastic lesions, and areas of pure osteosclerosis. Even untreated cases show a progression of sclerosis with eventual resolution (Sundaram et al. 1996, Heuck 1997, Bohndorf and Imhof 2001, Resnick 2002).
Ultrasound
Ultrasound may demonstrate a hypoechoic, subperiosteal fluid collection in cases with concomitant periostitis.
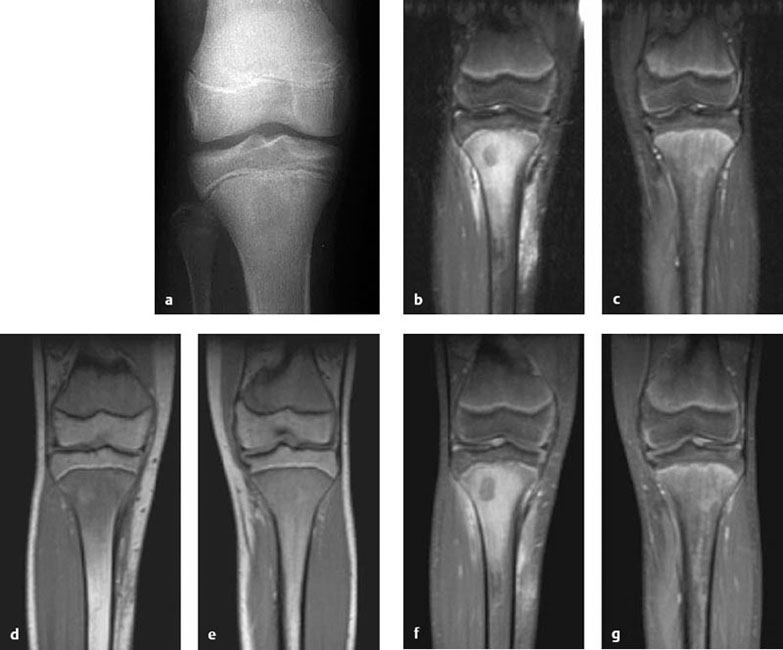
Fig. 3.2a-g A 14-year-old boy with unexplained right knee pain. Radiographs show a circumscribed zone of increased lucency in the bone marrow of the central metaphysis extending to the epiphyseal plate (a). MRI, coronal STIR (b = right leg, c = healthy left leg for comparison) and unenhanced T1-weighted images (d = right leg, e = healthy left leg) show diaphyseal and metaphyseal bone marrow edema extending to the epiphyseal plate. This area shows intense enhancement in fat-saturated T1-weighted images after IV contrast administration (f = right leg, g = healthy left leg). These findings are consistent with acute juvenile osteomyelitis.
Magnetic Resonance Imaging
The MRI findings in CRMO resemble those of acute hemogenous osteomyelitis, with osteosclerosis predominating in the long term. An important task of MRI is to detect new lesions, which are predominantly osteolytic initially (Sundaram et al. 1996, Heuck 1997, Jurik and Egund 1997, Bohndorf and Imhof 2001, Resnick 2002).
Key findings in CRMO:
• Osteolytic lesions coexisting with pronounced bone scleroses and periostitis
• MRI findings as in hematogenous osteomyelitis
• Detection of new lesions with MRI (Fig. 3.4)
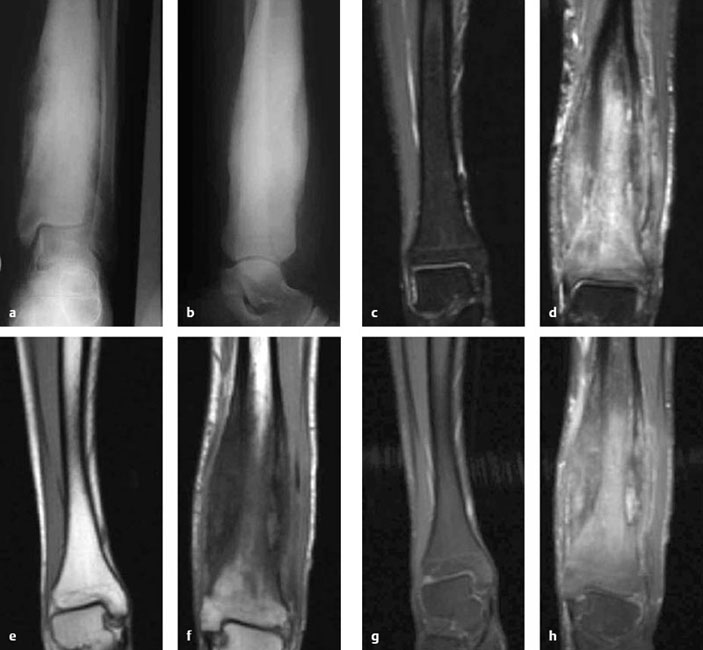
Fig. 3.3a-h Chronic osteomyelitis and hyperostosis in the left tibia of a 19-year-old male. Radiographs show massive cortical expansion in the mid-and distal tibia with increased sclerosis of the bone marrow (a, b). MRI (d, f, h left distal tibia; c, e, g healthy distal right tibia for comparison) shows definite fusiform cortical expansion with marked edematous imbibation of the bone marrow and surrounding muscles with inflammatory enhancement of the entire bone and the soft tissues in this region (c, d = STIR sequence, e, f = unenhanced Tl-weighted image, g, h = fat-saturated Tl-weighted image after contrast administration). Note the inclusion of the epiphysis in the meta- and epiphyseal blood supply.
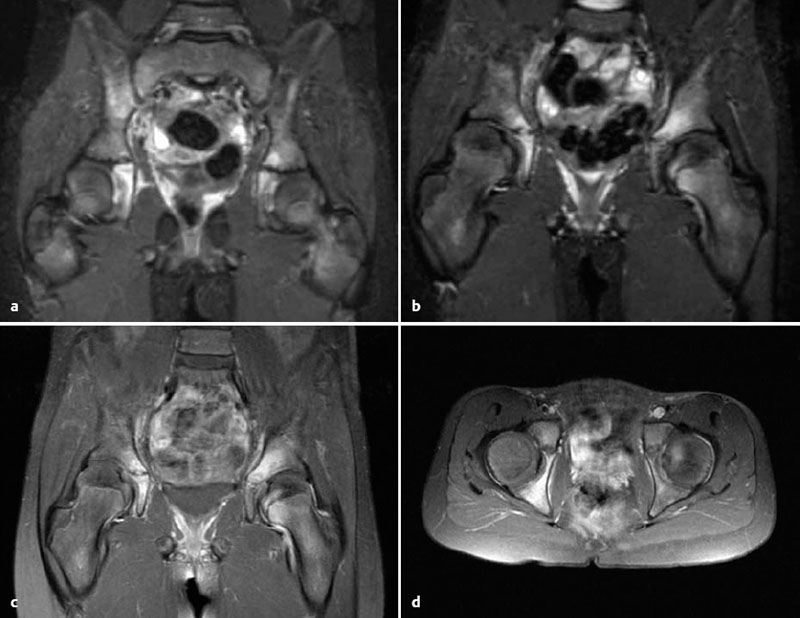
Fig. 3.4a-d CRMO in a 10-year-old girl. MRI shows involvement of the right ilium, both acetabula, and the left femoral head with pronounced bone marrow edema in STIR sequences (a, b). The cortex is still intact, and the synovium shows edematous imbibition. Fat-saturated Tl-weighted sequences after contrast administration (c, d) show inflammatory enhancement of the bone mar-row and synovium.
Chronic Posttraumatic Exogenous Osteomyelitis
Etiology, Sites of Occurrence, and Clinical Features
This is a primarily local bone inflammation (osteomyelitis), whose spread depends on the host immune status, the virulence of the causative organism, the extent of soft-tissue damage, the introduction of foreign tissue, the degree of circulatory compromise in the bone, and the stage of fracture healing. The soft-tissue infection spreads through the adjacent periosteum and compact bone into the medullary cavity. Causes are posttraumatic and postoperative infections and chronic ulcers, as in diabetes mellitus. Various organisms, often including a combination of aerobes and anaerobes, may be causative. Patients present clinically with increasing local pain and signs of inflammation. Draining sinus tracts may develop in cases that take a protracted course. Sepsis or septic arthritis may develop in untreated or poorly managed cases as a result of lymphogenous or hematogenous spread (Gold et al. 1991, Greenspan 1999, Bohndorf and Imhof 2001, Resnick 2002).
Imaging of Chronic Posttraumatic Exogenous Osteomyelitis
Projection Radiography
Soft-tissue swelling is manifested by the obliteration of fat planes in the surrounding soft tissues. Periosteal spread of the disease leads to elevation of the periosteum and incipient periosteal new bone formation (often the earliest radiographic sign). The further course is marked by subperiosteal bone resorption and cortical destruction. If the inflammation spreads to the medullary cavity, radiographs show evidence of trabecular destruction. The further course of the disease is the same as in hematogenous osteomyelitis (Resnick and Petterson 1992, Greenspan 1999, Bohndorf and Imhof 2001, Resnick 2002).
NOTE
When postoperative infection develops after the internal fixation of a fracture, radiographs are difficult to interpret for some time. It is important to compare the radiographs with immediate postoperative films. Bony consolidation of the fracture site is delayed, and osteolytic lesions coexist with areas of new bone formation. Callus formation may be the dominant finding in more protracted cases.
Key radiographic findings in chronic posttraumatic osteomyelitis:
• Soft-tissue swelling, obliterated fat planes
• Subperiosteal bone resorption and new bone formation
• Osteolytic lesions in the medullary cavity
• Reparative stage as in hematogenous osteomyelitis
• Postoperative radiographs are difficult to interpret and should be compared with preoperative films
Fistulography
When a draining fistula is catheterized and radiographic contrast medium is injected, spot radiographs can demonstrate the communication between the bone and fistula. This will also reveal the presence of any sequestrum (Fig. 3.5).
Ultrasound
Ultrasound can detect inflammatory soft-tissue edema indicating an infectious focus and can identify circumscribed fluid collections due to abscess formation.
Computed Tomography
The main role of CT in chronic posttraumatic osteomyelitis is to detect a devitalized sequestrum that has become separated from the surrounding bone. The fragment is hyperdense and rimmed by fluid (Heuck 1997, Bohndorf and Imhof 2001, Reiser et al. 2001, Resnick 2002).
Magnetic Resonance Imaging
The MR signal and enhancement characteristics of the lesions in chronic exogenous osteomyelitis closely resemble those in hematogenous osteomyelitis.
NOTE
MRI during the first 3-6 postoperative months cannot positively distinguish surgery-related bone marrow edema from inflammatory involvement of the medullary cavity.
Fluid-sensitive sequences (STIR, T2-weighted) show greatly increased signal intensity in the bone marrow, which also shows contrast enhancement in fat-suppressed T1-weighted sequences.
NOTE
Abscess formation in the medullary cavity or paraosseous soft tissues, showing central fluid-equivalent signal intensity and peripheral enhancement of the abscess membrane (best appreciated in fat-saturated Tl-weighted sequences), confirms the presence of a postoperative infection.
Circumscribed postoperative fluid collections, unlike inflammatory fluid collections, do not exhibit perifocal edema. MRI in chronic cases shows an inhomogeneous pattern with a combination of endosteal and periosteal new bone formation and osteolytic changes. Fistulas are best demonstrated by unenhanced fat-suppressed, fluid-sensitive sequences (STIR) and by thin-slice, fat-suppressed T1-weighted sequences after IV contrast administration (Gold et al. 1991, Vahlensieck and Reiser 1992, Bohndorf and Imhof 2001, Resnick 2002, Vahensieck and Reiser 2002; Fig. 3.6).
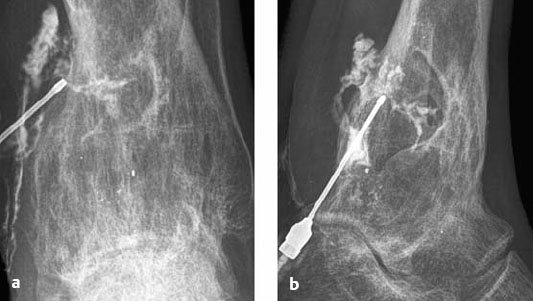
Fig. 3.5a, b Fistulograms in chronic posttraumatic osteomyelitis. Inverted fluoroscopic images in the AP (a) and lateral projection (b) show an arborizing system of fistulous tracts in the soft tissue extending into a large, trough-shaped defect in the distal tibia. Note the pronounced, inhomogeneous bone marrow sclerosis at the site of the tibial defect.
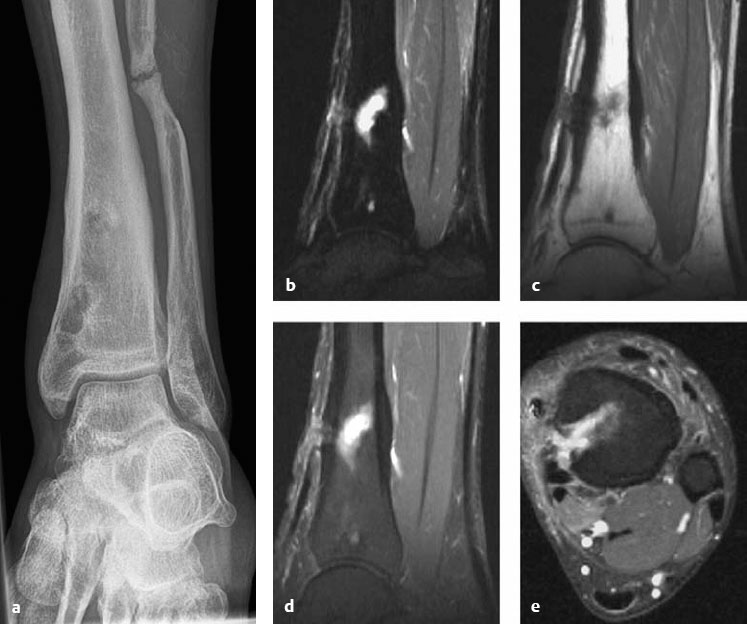
Fig. 3.6a-e Chronic posttraumatic osteomyelitis in a 22-year-old man following an open tibial fracture. Radiograph shows eccentric, irregular dia-, meta-, and epiphyseal areas of increased lucency with marginal sclerosis and cortical thickening in the distal tibia (a). MRI of this region (b-e) shows a fluid-filled defect in the bone marrow with spindle-shaped cortical expansion and fistulas tracking anteriorly (b-d) and medially (e) into the surrounding soft tissue.
Infectious Spondylitis and Spondylodiskitis
Etiology, Sites of Occurrence, and Clinical Features
Males are predominantly affected in approximately 2%-4% of all cases. Incidence shows an initial peak in infancy and childhood and a second peak in the fifth and sixth decades. Previous infections and interventional procedures or operations are predisposing factors. The infectious organisms mainly spread hematogenously via the vertebral arteries to the anterior portions of the vertebral bodies bordering the disk space (spondylitis). From there the inflammation spreads secondarily across the vertebral end plate into the disk space and avascular disk (spondylodiskitis). Generally this is followed by extension to adjacent vertebral bodies. Primary infection of the vascularized disk may occur in children. Only one vertebral segment is affected in most cases, but involvement of multiple segments may occur. The lumbar spine is most commonly affected followed by the lower thoracic spine. Involvement of the entire spinal column may occur.
The acute form of the disease is characterized by dull, localized spinal pain (occurring mainly at night), antalgic posturing, fever, and general malaise. Inflammatory markers are elevated when these symptoms are present. The chronic form causes little or no local pain, and inflammatory markers are normal or only slightly elevated, often causing a delay in diagnosis. The main causative organisms are staphylococci, Gram-negative bacteria, and Mycobacterium tuberculosis (Dihlmann 1987, Freyschmidt 1997, Heuck 1997, Bohndorf and Imhof 2001, Resnick 2002).
Differential diagnosis of infectious spondylitis:
• Erosive osteochondrosis
• Rheumatoid arthritis
• Tuberculous spondylitis
• Seronegative spondylarthritis
• Pseudogout
• Sarcoidosis
• Tumor, metastasis
Imaging of Infectious Spondylitis
Projection Radiography
Radiographic abnormalities first appear from 1 to 3 weeks after the onset of clinical symptoms. Soft-tissue swelling may be noted during the first 2-3 weeks (psoas shadow, shifting of the paravertebral line). This is followed by a loss of intervertebral disk height and blurring of adjacent vertebral body end plates. Further progression is marked by increasing unsharpness, irregular margins, and defects in adjacent end plates ranging to marked destruction of the vertebral bodies and disk (kyphoscoliosis, gibbus). The reparative phase is characterized by a smoothing of contour defects in the vertebral body end plates, focal sclerosis of the cancellous bone, osteophyte formation, and even partial interbody fusion (Dihlmann 1987, Heuck 1997, Bohndorf and Imhof 2001, Stäbler and Reiser 2001, Resnick 2002).
Key radiographic findings in infectious spondylitis:
• Soft-tissue swelling
• Decreased height of the intervertebral disk space
• Indistinct vertebral body end plates, contour defects
• Destruction of the vertebral bodies and disk, kyphoscoliosis
• Reparative phase: smoothing of contour defects, sclerosis, osteophytes, block vertebrae
Fistulography
Fistulography can define the communication between the fistula and bone or may reveal a sequestrum or abscess cavity. MRI can also detect fistulas and define their extent after the injection of water or Gd into the fistulous opening.
Ultrasound
Paravertebral fluid collections and abscesses can be identified with ultrasound. If necessary, they can be aspirated under ultrasound guidance to obtain samples for bacteriologic analysis.
Computed Tomography
CT shows the same changes as conventional radiographs. Acquiring thin slices ensures that high-quality MPRs can be performed. Soft-tissue processes lead to the obliteration of normal fat planes, decreased density in relation to normal muscles, and occasionally to increased contrast enhancement. Abscesses have central fluid-equivalent attenuation, although somewhat higher densities may be seen depending on the blood, cellular and protein content of the abscess. Usually the hypervascularized abscess membrane is clearly delineated on postcontrast images owing to its increased contrast uptake. Infection of the bone marrow leads to increased density even in the absence of visible bone destruction. The higher the fat content of the bone marrow, the greater the density increase in the medullary cavity. Rarely, fat–fluid levels or gas inclusions may be found in the medullary cavity. Defects in the vertebral end plates are seen more clearly in CT scans than in plain radiographs—especially when only a small portion of the end plate is affected. The junction of the vertebral end plate and disk can be more precisely evaluated in sagittal and coronal reconstructions than in primary axial sections. Trabecular and cortical bone destruction, sclerosis, and sequestra are demonstrated better by CT than conventional radiographs. If epidural spread of spondylitis is suspected, CT myelography will provide a more detailed view than standard contrast-enhanced CT.
NOTE
CT is better than any other modality for directing percutaneous biopsies and drainage.
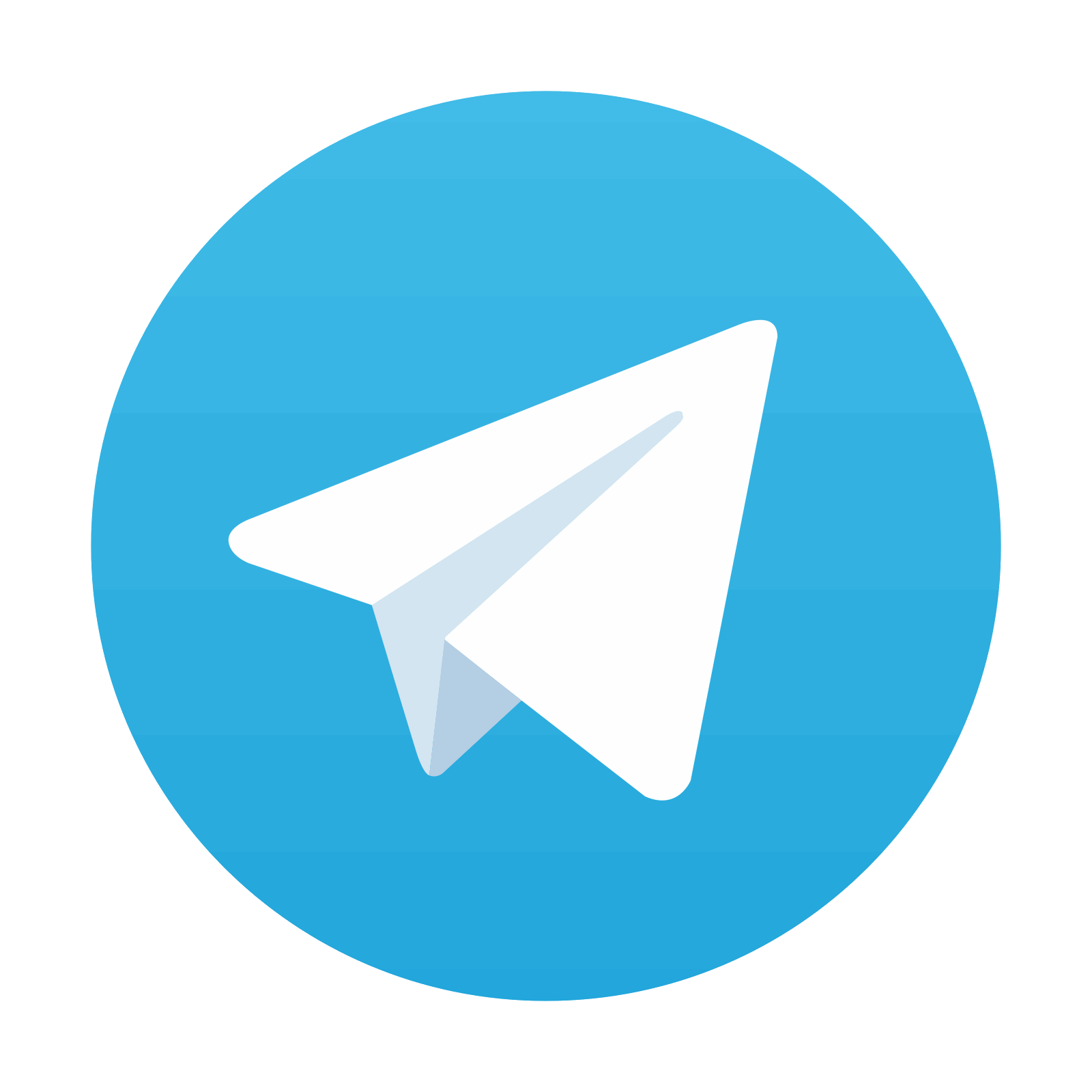