TABLE 1 Sunderland Classification of Nerve Injuries With Description of Pathoanatomy and Expected Clinical Course Without Surgical Intervention | ||||||||||||||||||
---|---|---|---|---|---|---|---|---|---|---|---|---|---|---|---|---|---|---|
|
TABLE 2 Important Growth Factors, Pathways, and Proteins Associated With Nerve Injury and Regeneration | ||||||||||||||||||||||||||||||||
---|---|---|---|---|---|---|---|---|---|---|---|---|---|---|---|---|---|---|---|---|---|---|---|---|---|---|---|---|---|---|---|---|
|
crush, gunshot wounds, blast, or closed traction injuries. Injuries can also be iatrogenic from direct laceration, compression by plates or suture, and stretch. When the injury occurred is particularly important to motor nerve injuries because distal nerve, motor end plates, and target muscle degenerate and are not reinnervatable 12 to 18 months after injury.
1. What was the mechanism of injury? Nerve deficit after sharp laceration implies high likelihood of complete neurotmesis with minimal surrounding zone of injury, and should lead to a low threshold for surgical intervention based on history alone. Open injury due to crush, gunshot, or blast mechanisms can have highly variable clinical progressions. These injuries can cause neurapraxia due to concussive trauma to the nerve, or transfer more significant amounts of energy to the nerve resulting in a degenerative pathway with a wide zone of injury. The energy imparted from the mechanism of injury is a clear prognostic factor, with worse outcomes seen in higher energy injuries.40,41
2. When did the injury occur? The timing of presentation relative to injury is key. As detailed above, there is a physiologic window with regard to potential for nerve regeneration. For motor recovery, the reinnervated nerve must reach its target before 12 to 18 months, likely closer to 12 months, especially in patients older than 40 years. However, outcomes are optimized with early intervention when possible depending on clinical circumstances.
3. What has the clinical course been since injury? A conduction block or neurapraxia should be expected to improve by 6 to 12 weeks from injury. Nerve regeneration after axonotmesis should be expected to progress along its anatomic course at approximately 1 mm/d after an initial latent period of 2 to 4 weeks. While there is likely variability in this rate between nerves, individual patients, and at different time points over the course of a single nerve’s recovery, this estimate can be useful in evaluating likelihood of recovery without further intervention.42
categories included none, nascents, increased polyphasics, and di-/triphasics. Nascent wave configurations can be thought of as immature waves with capacity to mature to di-/triphasic waves.47
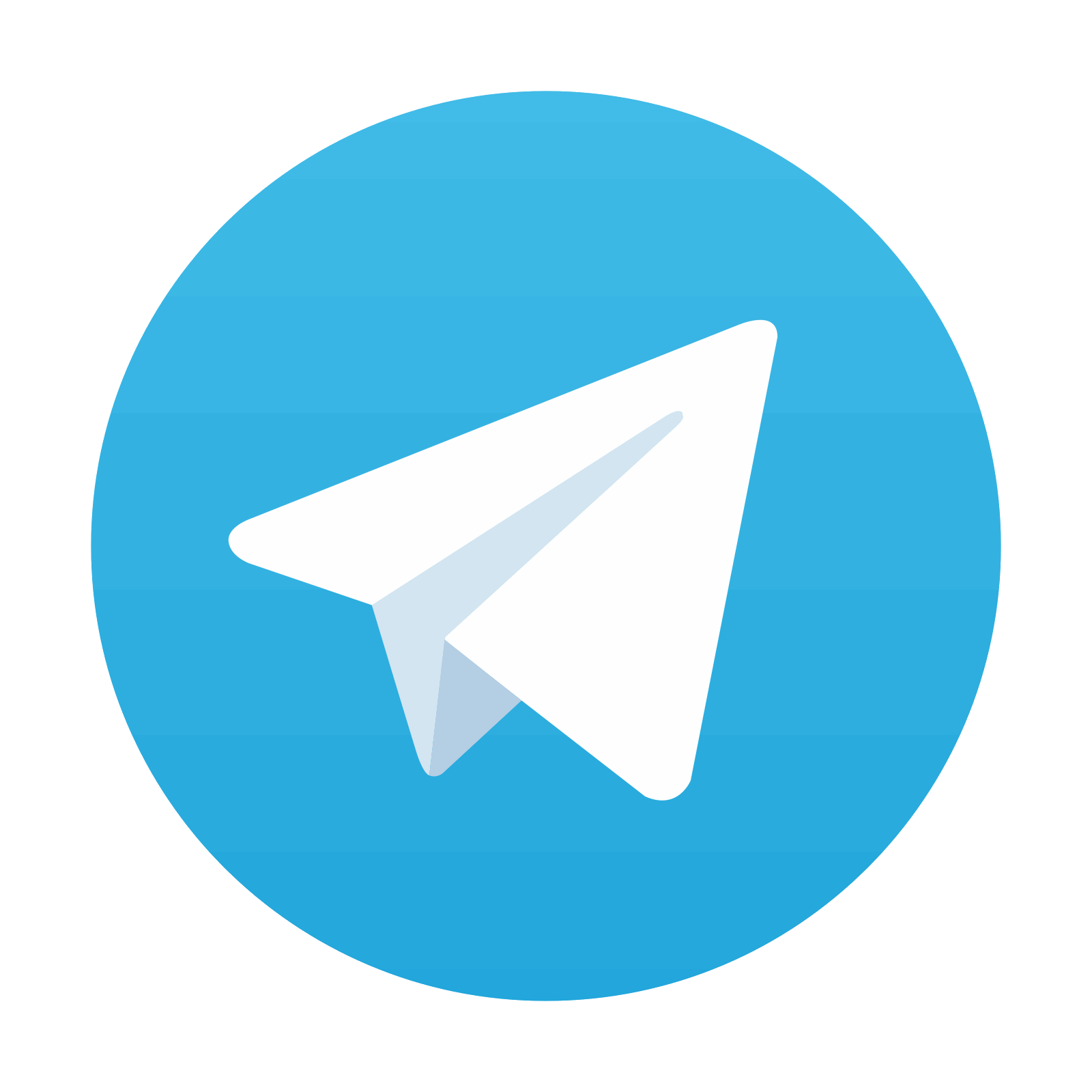
Stay updated, free articles. Join our Telegram channel

Full access? Get Clinical Tree
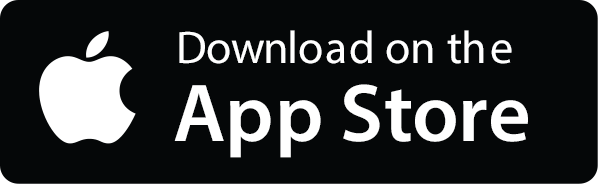
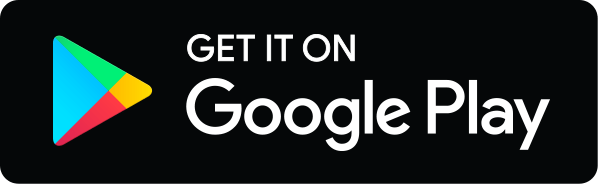
