Introduction
This study aimed to investigate the surface free energy and surface roughness (SR) of metallic alloys under the influence of acid solutions.
Methods
The experiment involved the use of 270 rectangular wire samples measuring 0.019 × 0.025-in. These samples were sourced from 3 different commercial brands: Dentsply GAC, American Orthodontics, and Orthoclassic. This in vitro study categorized the samples into 3 groups based on the solutions employed: deionized water, citric acid, and phosphoric acid. Each group consisted of 90 samples, with 30 samples representing each type of alloy—stainless steel, nickel-titanium, and titanium molybdenum alloy (TMA). The wire segments were immersed in their respective solutions for 72 hours at a controlled temperature of 37°C, with continuous orbital agitation at 130 rpm. After the immersion period, the study analyzed both surface free energy and SR. The mean values obtained were subjected to an analysis of variance at a significance level of 5%.
Results
All alloys displayed hydrophobic behavior, as indicated by interaction free energy values <0. In acidic environments (phosphoric acid and citric acid), significant differences were observed among different brands and alloys, affecting surface energy and interaction free energy. Variations in SR among metallic alloys included steel with the lowest SR variations, followed by nickel-titanium and TMA. Notably, the TMA alloy stood out with significantly higher surface energy compared with that of the other alloys ( P <0.001).
Conclusions
In this study, all examined alloys demonstrated a hydrophobic nature, suggesting a limited attraction to water. Notably, TMA exhibited the least hydrophobic behavior among the alloys studied. However, when exposed to citric acid, TMA displayed the most substantial alterations in its surface properties. These results underscored the significance of accounting for the distinctive properties of each alloy and their responses to diverse challenges, such as exposure to acidic solutions, during the selection of orthodontic wires for orthodontics treatment.
Graphical abstract

Highlights
- •
Titanium molybdenum displayed lower hydrophobic behavior than stainless steel and nickel-titanium.
- •
Titanium molybdenum had greater changes to its surface characteristics in response to citric acid.
- •
All the alloys showed hydrophobic behavior.
Orthodontic tooth movement occurs because of a cascade of biologic events triggered by the stimulus generated by different components of the orthodontic appliance, such as elastics, springs, and wires. , Orthodontic wires enable the orthodontist to achieve tooth alignment and leveling according to their physics and chemical properties, such as flexibility, modulus of elasticity, etc. In addition, these wires are capable of performing tooth retractions and closing spaces, for which the properties of rigidity and surface polishing become paramount.
To select the most appropriate wire for each phase of orthodontic treatment, the orthodontist considers several factors, including treatment objectives, wire flexibility or rigidity, and wire formability or weldability, among other properties extensively documented in the literature. , In the final phase, particularly for leveling and anterior retraction, rectangular wires are recommended because of their ability to fit nearly entirely within the bracket slot, among other advantageous properties. During anterior retraction, the contact between the wire and the groove creates a phenomenon known as friction. ,
Friction is an inherent factor in orthodontic mechanics, and its level of expression directly correlates with the requirement for forces greater than its resistance. Consequently, this can lead to adverse effects on both orthodontic mechanics and the associated biologic responses. , One approach to mitigating the effects of friction is to ensure perfect alignment among all bracket slots, coupled with the use of high-quality polishing wires. Wire manufacturers recognize that achieving optimal polishing is an ongoing challenge, but significant progress has been made in this field. ,
The surface properties of biomaterials exert a notable influence on bacterial adhesion when studied in a laboratory setting. Surface roughness (SR) and surface free energy (SFE) exert significant control over the process of bacterial adhesion. , A rough surface enhances bacterial colonization by providing more adhesion sites and hindering the detachment of bacterial colonies. , Conversely, materials with high SFE tend to attract a greater number of bacteria to their surfaces than those with low SFE, and this phenomenon is guided by fundamental thermodynamic principles. Understanding the surface characteristics of orthodontic materials can yield valuable insights into bacterial adhesion.
One of the techniques employed for evaluating the surface properties of solid bodies when exposed to acidic solutions is SFE analysis, which provides insights into the interplay between cohesive and adhesive forces and determines the wetting ability of a solid. It is quantified by measuring the contact angle formed between the solid and the liquid. It is important to note that SFE cannot be directly measured; the measurement of the liquid contact angle alone merely indicates the wetting behavior of the solid. In contrast, SFE quantitatively assesses the intermolecular forces at the surface and remains independent of the specific liquid being used.
The impact of acidic drinks on the surface of rectangular wires, as well as the potential reduction in surface polishing caused by these beverages, remains a relatively unexplored area in scientific studies. , This aspect is particularly relevant in the context of patients undergoing orthodontic treatment who regularly consume acidic drinks in their daily routines.
Therefore, this study aimed to assess the surface properties of orthodontic wires when exposed to acidic drinks. The evaluation focused on changes in SFE and SR as key parameters for analysis. By examining these characteristics, we aimed to gain insights into the potential effects of acidic beverages on the surface of orthodontic wires and their implications for surface quality. Our null hypothesis posited that there is no discernible difference in the surface of orthodontic wires after induced corrosion treatment in a controlled in vitro setting.
Material and methods
For this study, straight rectangular orthodontic wires with a cross-section of 0.019 × 0.025-in were employed. The wires were made from 3 different alloys: stainless steel (SS), nickel-titanium (NiTi), and titanium molybdenum alloy (TMA). The specific alloys and brands of wires used are detailed in Table I .
Alloys | Type | Code |
---|---|---|
GAC (Bohemia, NY) | ||
SS | StraightWire | 03-925-68 |
NiTi | Bio Force Nickel Titanium | 02-528-061 |
TMA | Resolve Beta Titanium | 03-925-99 |
OC (McMinnville, Ore) | ||
SS | StraightWire | 40.40.880.01925 |
NiTi | Nickel Titanium Archwire | 61.40.720.19250 |
TMA | Beta Titanium StraightWire | 40.40.870.01925 |
AO (Sheboygan, Wis) | ||
SS | StraightWire | 856-011 |
NiTi | NiTi Memory Wire | 858-546 |
TMA | TitaniumStraightWire | 857-699SP |
For the analysis of SFE and SR, 2 of the most common acids in beverages were used to simulate the effect of acidic drinks on the surface of the wires. Citric acid solution 0.05 mol/L (Sigma-Aldrich Brazil Ltda, São Paulo, São Paulo, Brazil) with a pH of 3.2 , ; and phosphoric acid solution 0.05 mol/L (Sigma-Aldrich Brazil Ltda) with a pH of 2.5 were prepared. The pH of the acidic solutions was adjusted using 0.5 mol/L sodium hydroxide (Sigma-Aldrich Brazil Ltda) and a pH electrode (Pencil-Thin Epox Body Gel-filled combination electrode; Accumet, Fisher Scientific, Waltham, Mass) calibrated with standard pH levels of 7.0 and 4.0.
The experimental procedure involved the preparation of a total of 270 samples, with each sample consisting of a 10-mm long wire segment from each alloy and brand. To ensure a clean surface, the wire segments underwent an initial cleaning process using deionized water in an ultrasound device (Unique USC 1400; Indaiatuba, São Paulo, Brazil) operating at a frequency of 40 Hz. After cleaning, the wire segments were placed in a drying chamber for 24 hours to remove any residual moisture. After the drying step, the wire segments were submerged in the respective acidic preparations for 1 week. The acidic preparations included a citric acid solution (pH 3.2) and a phosphoric acid solution (pH 2.5). After the immersion period, the wire segments were once again dried to remove any excess solution. Polymerase chain reaction microtubes (MCT-200-C; Axygen Union City, Calif) were used to accommodate each wire segment during the experimental procedure. These microtubes provided a suitable container for holding the wire segments during the various steps of the experiment.
The wire segments were immersed in containers (PCR microtubes, MCT-200-C; Axygen, Union City, Calif) containing 1.5 mL of the respective solutions: deionized water, citric acid, and phosphoric acid. There was a total of 90 wire segments, with 30 segments for each type of alloy (SS, NiTi, and TMA) and 10 segments for each brand. These containers, containing the wire segments, were placed in an incubator with orbital agitation (Tecnal, TE-420; Piracicaba, São Paulo, Brazil) set at 130 rpm and a temperature of 37°C. The wire segments remained immersed in the solutions for 72 hours. , After the 72-hour immersion period, the samples were rinsed with deionized water for 15 seconds to remove any residual solution. Subsequently, the wire segments were dried using a paper towel, ensuring the removal of excess moisture.
After the drying process, each wire segment underwent SFE analysis to determine its nonpolar (γ s Lifshitz van der Waals [LW] ) and polar (γ s acid-base [AB] ) energy components. In addition, the acid (γ s + ) and base (γ s − ) components of the SFE (γ s ) were calculated, as well as the interaction free energy (ΔG sws ), according to the van Oss, Chaudhery, and Good model. The ΔG sws value indicates the hydrophobicity or hydrophilicity of the surface, with negative values indicating hydrophobic behavior and positive values indicating hydrophilic behavior. To calculate these values, the contact angle of a sessile drop of liquid (0.3 μL) in contact with the wire was measured. This was done using an automatic goniometer (DSA100S; KRÜSS GmbH, Hamburg, Germany) and 3 liquid probes with known surface energy: deionized water (polar), di-iodomethane (nonpolar), and ethylene glycol (polar with acid and base components). , The contact angle measurements were conducted by measuring the right and left angles of the sessile drop 5 times over 5 seconds. The measurements were taken at a temperature of 23°C and relative humidity of 48 ± 7% using the Laplace-Young shape analysis method with the Drop Shape Analysis DSA4 software (version 2.0-01; KRÜSS GmbH).
For SR analysis, the wire samples were individually placed on the object platform of the Contour GTK 3D Optical Microscope (Bruker AXS Inc; Billerica, Mass) and properly identified. The microscope was configured in automated mode, and readings were continuously taken. Four scans were performed in different quadrants of each sample using a ×20 magnification. The area of measurement for each scan was 314.3 × 235.6 μm, and the scan time was set to 20 seconds. The roughness parameter (Sa) used in this study was the absolute deviation from the mean. Sa represents the arithmetic mean (μm) of the height difference between peaks and valleys on the surface. In addition, the asymmetry coefficient (Ssk) was determined to measure the symmetry of the surface profile about the midline. The Ssk value can indicate whether the surface has an equal number of peaks and valleys (Ssk = 0), removed peaks or deep valleys (Ssk <0), or filled valleys or high peaks (Ssk >0). After the image registration, the captured images were processed and analyzed using the Vision64 Map software (Bruker AXS Inc) to extract the necessary data for SR characterization.
Statistical analysis
The collected data for SFE variables (γ s , γ s LW , γ s AB , γ s + , γ s − , and ΔG sws ) and SR (Sa and Ssk) underwent statistical analysis. First, normality tests (such as the Kolmogorov-Smirnov test) were performed to assess the distribution of the data. In addition, homogeneity tests (eg, the Cochran test) were conducted to evaluate the equality of variances across groups. For the SFE data, the analysis considered the type of alloy and the exposure medium as variation factors within each brand. In addition, the alloy and brand within each medium were analyzed. In these cases, a 2-way analysis of variance was performed, followed by the Student–Newman–Keuls test for post-hoc comparisons. Regarding the SR parameters (Sa and Ssk), the analysis considered the type of alloy and exposure medium as variation factors within each brand. Similar to the SFE data, a 2-way analysis of variance was conducted, followed by Fisher’s least significant difference multiple comparison tests for post-hoc comparisons. All statistical analyses were performed using the SigmaPlot program (version 12.0; Systat Software GmbH, Erkrath, Germany) with a significance level set at 5%.
Results
In the analysis of SFE (γ s ) and interaction free energy (Δ G sws ) with deionized water, the different brands of alloys showed significant differences in γ s values ( P <0.001; Table II ). Dentsply GAC (GAC) and OC did not differ in terms of γ s LW ( P = 0.756), but both differed compared with American Orthodontics (AO) ( P <0.001; Table III ). Orthoclassic (OC) and AO brands did not differ in terms of γ s AB ( P = 0.616), SFE donor component (γ s − ) ( P = 0.119; Table IV ), and ΔG sws ( P = 0.326), but both differed from GAC ( P <0.001). There was no difference among brands for the SFE receptor component (γ s + ) ( P = 0.303). Regarding the metallic alloys, the TMA alloy exhibited the highest values of γ s and γ s LW , followed by NiTi and SS ( P <0.001). NiTi and TMA did not differ in terms of γ s LW ( P = 0.223) and showed higher values of γ s AB compared with SS ( P <0.001; Table III ). SS and TMA did not differ ( P = 0.222) and showed higher values compared with NiTi for γ s + ( P <0.001; Table IV ). There were no significant differences in γ s − among the alloys ( P <0.050), and all alloys had ΔG sws values <0, indicating hydrophobic behavior. Strong positive correlations (Pearson) were found between ΔG sws and γ s − ( r = 0.841, P <0.001), γ s and γ s LW ( r = 0.697, P <0.001), and γ s and γ s AB ( r = 0.745, P <0.001).
Variables | GAC | OC | AO | |||
---|---|---|---|---|---|---|
γ s (mN/m) | △G sws (mN/m) | γ s (mN/m) | △G sws (mN/m) | γ s (mN/m) | △G sws (mN/m) | |
Deionized water | ||||||
SS | 26.5 ± 1.4 aA | −54.2 ± 4.7 aA | 25.7 ± 1.3 aA | −61.3 ± 9.0 aB | 25.0 ± 1.8 aA | −59.2 ±9.0 aBA |
NiTi | 29.3 ± 1.1 bA | −57.7 ± 4.1 abA | 27.0 ± 0.7 bB | −57.1 ± 5.2 aA | 30.1 ± 0.9 bA | −52.7 ± 5.9 bA |
TMA | 31.8 ± 1.4 cA | −59.4 ± 4.5 bA | 32.2 ± 1.6 cA | −77.3 ± 3.2 bB | 34.5 ± 1.2 cB | −79.0 ± 8.6 cB |
Phosphoric acid | ||||||
SS | 28.9 ± 1.6 bA | −22.5 ± 4.3 cA | 28.8 ± 0.8 dA | −72.2 ± 4.5 cB | 27.8 ± 2.0 dA | −63.1 ± 4.1 aC |
NiTi | 32.0 ± 2.0 cA | −58.5 ± 4.4 bA | 29.1 ± 1.8 dB | −12.9 ± 3.8 dB | 30.8 ± 1.3 bA | −33.2 ± 6.7 dC |
TMA | 26.6 ± 1.7 aA | −6.8 ± 2.6 dA | 38.0 ± 1.3 eB | −59.6 ± 3.5 aB | 38.7 ± 2.2 eB | −49.8 ± 3.0 bC |
Citric acid | ||||||
SS | 8.8 ± 2.3 dA | 23.5 ± 4.8 eA | 29.9 ± 0.9 dB | −73.8 ± 3.3 cB | 29.9 ± 0.5 bB | −61.6 ± 3.8 aC |
NiTi | 30.4 ± 1.6 bA | −36.2 ± 8.4 fA | 30.4 ± 0.7 fA | −51.8 ± 4.8 eB | 26.7 ± 0.6 aB | −27.8 ± 2.8 eC |
TMA | 17.0 ± 1.2 eA | 21.9 ± 1.9 eA | 34.0 ± 1.9 gB | −15.4 ± 4.0 dB | 34.5 ± 1.6 cB | 2.7 ± 4.9 fC |
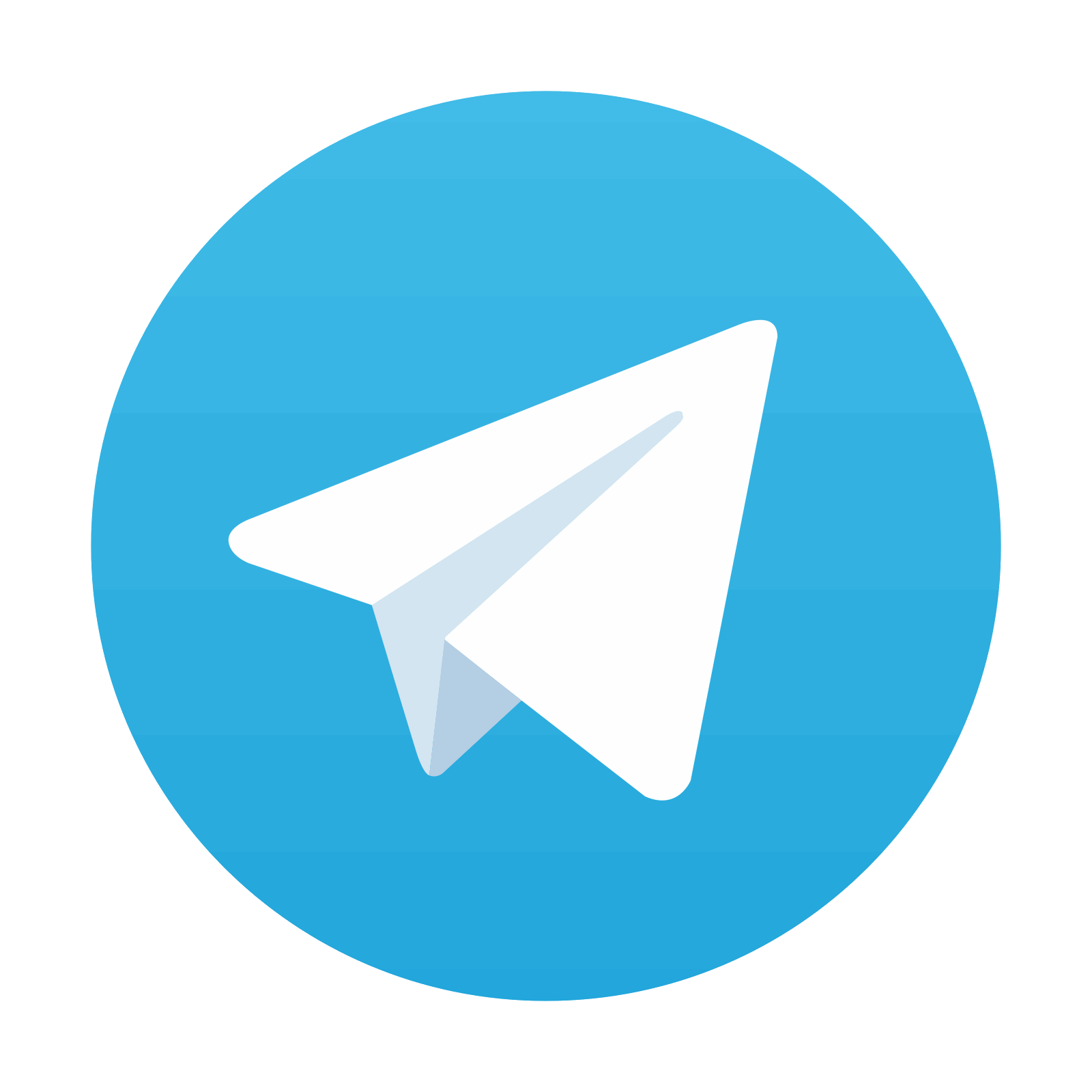
Stay updated, free articles. Join our Telegram channel

Full access? Get Clinical Tree
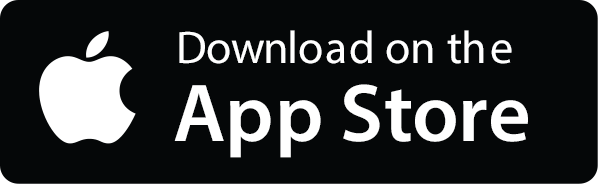
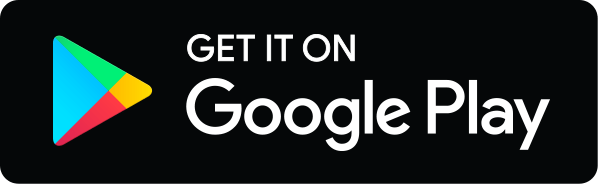
