Hypertension
Horner’s syndrome
Tachycardia or bradycardia
Muscle spasm
Profuse sweating
Restlessness
Flushing and skin warmth above the level of SCI
Seizure
Cold and pale skin below the level of SCI
Headache
Piloerection below the level of SCI
Altered consciousness
Bronchospasm
The underlying pathophysiology is thought to be the activation of the sympathetic nervous system by the triggering stimulus, leading to vasoconstriction below the level of SCI, leading to increased BP and the other symptoms/signs below the level of the lesion. Parasympathetic activity is triggered by the baroreceptors sensing the hypertension. Descending supraspinal inhibitory signals are blocked at the level of the SCI.
Management of AD involves sitting the individual upright, loosening/removing any restrictive clothing, and addressing the underlying trigger. Rapidly acting antihypertensive medications may be needed if BP remains elevated despite addressing the possible trigger. Monitoring should be continued to ensure that the episode has resolved.
Effect of Autonomic Dysfunction on Exercise Performance
In order to understand why athletes with SCI may seek to deliberately induce such a dangerous phenomenon, it is important to consider the physiological response to exercise in such athletes. Much is already known about the impairment in motor and sensory function that occurs as a result of SCI, but the impairments in autonomic function are not fully understood. Just as the motor and sensory impairments present in SCI vary according to the neurologic level of injury, the autonomic dysfunction also varies according to the neurologic level of SCI [6]. In the last few years, the international SCI community has developed an assessment tool to document remaining autonomic function after SCI [7]. It is worthwhile to review autonomic control of the cardiovascular system in able-bodied individuals and compare it with that in those with SCI.
The cardiovascular control center is in the medulla oblongata and receives projections from the cerebral cortex and hypothalamus. This system provides central autonomic control of cardiovascular function. The heart is under control of both the parasympathetic and sympathetic nervous systems, while the vasculature is primarily controlled by the sympathetic nervous system. Parasympathetic control of the heart is provided by the vagus nerve whose preganglionic fibers synapse with postganglionic parasympathetic neurons in ganglia on or near the heart. Supraspinal pathways provide descending sympathetic input to spinal preganglionic neurons mainly found within the lateral horn of the spinal cord at T1–L2. These neurons synapse with postganglionic neurons in the paravertebral sympathetic chain ganglia and these in turn synapse with the heart (T1–T5 segment) and blood vessels (T1–L2). Therefore, after any neurologic level of SCI, the cranial parasympathetic nervous system will remain intact, and the sacral system will always be involved. The sympathetic nervous system’s involvement will depend on the neurologic level of injury and the autonomic completeness of the injury.
Cardiac output is the product of HR and stroke volume and is controlled by a complex array of mechanisms. In highly trained able-bodied athletes, cardiac output increases up to sevenfold during maximal exercise. Studies investigating the cardiac output response to maximal exercise in SCI found a less than twofold increase during maximal aerobic arm exercise in cervical SCI patients [8], and similar findings were found for submaximal functional electrical stimulation cycling exercise in cervical SCI [9].
The decreased cardiac output in cervical SCI is due to impairments in both stroke volume and heart rate (HR). In cervical SCI, there is no change in stroke volume compared to the resting state with either submaximal or maximal arm exercise [8]. This is attributed to reduced venous return caused by the inability to cause vasoconstriction of the splanchnic vasculature. In high-thoracic SCI, the stroke volume response to exercise is lower than in able-bodied individuals but not significantly so [9]; in low-thoracic or lumbar SCI, the stroke volume response is similar to that of able-bodied individuals [10].
Traditionally it has been thought that individuals with cervical SCI exhibit a maximum HR between 100 and 120 beats per minute (bpm), while those with thoracic or lumbar SCI exhibit a relatively normal maximal HR. The reduced HR response to maximal exercise in cervical SCI is attributed to diminished sympathetic activity to the myocardium and reduced circulating catecholamines [11]. However, it has been observed that some elite cervical SCI athletes have almost normal age-predicted maximal HR. A study found partial to fully functioning sympathetic pathways in some patients with neurologically complete cervical SCI, and there was a strong association between the degree of autonomic function and the maximum HR obtained during exercise [12]. Therefore, it appears that the response to exercise in SCI is dependent on the integrity of autonomic pathways and not just the level of injury.
Blood pressure (BP) is the product of cardiac output and peripheral resistance and is regulated at rests via sympathetic activity on the vasculature which controls the peripheral resistance. As exercise intensity is increased, cardiac output gradually contributes more to BP regulation. The balance between the two contributors to BP is controlled through hormonal and autonomic influences on the heart, vasodilatory substances released from exercising muscles and sympathetically mediated vasoconstriction [6]. In SCI, the BP response to exercise is compromised both due to loss of central cardiovascular control and loss of reflexive vasoconstriction. One study showed that individuals with cervical SCI were unable to maintain their BP during functional electrical stimulation (FES) cycling, while those with thoracic SCI could do so [9]. In another study, persons with low thoracic SCI showed increased BP in response to exercise, while those with cervical SCI exhibited decreased BP [13].
In able-bodied persons, elevated sympathetic activity constricts the splanchnic vasculature to redistribute blood to the exercising muscles [14]. In individuals with cervical SCI, loss of abdominal muscle tone increases abdominal compliance [15] and predisposes to splanchnic blood pooling. This combined with the lack of descending sympathetic activity leads to compromised blood volume redistribution during exercise. Portal vein flow during incremental arm exercise was shown to be unchanged in cervical and high-thoracic SCI and reduced in low (below T7) SCI and able-bodied controls, implying that those with high SCI are unable to redistribute blood during exercise [16].
Several studies have examined whether increasing BP affects exercise capacity and/or performance. One study used a 10 mg dose of and all participants had increased BP during exercise, but only 50% exhibited increased maximal oxygen uptake [17]. The same group of athletes were studied, while intentional induction of AD was done in the laboratory setting. Significantly increased peak HR, BP, circulating norepinephrine (NE) levels, oxygen uptake, and power output during exercise, along with 10% improvement in time trial performance, were found [18]. A more recent study of six elite athletes with tetraplegia demonstrated significantly increased peak HR and BP, circulating NE levels, maximal oxygen uptake, and peak power [19].
How Widespread Is the Boosting Phenomenon?
The obvious benefits to performance of competing in a dysreflexic state have led some athletes to use this during competition. Self-induced injury may be caused in several ways such as excessive tightening of leg straps, administering electrical shocks to the muscles, constricting the lower extremities or scrotum, fracturing toes, or clamping the urinary catheter to cause overfilling of the bladder [20]. A study investigated the incidence of boosting in Paralympic athletes as well as their knowledge regarding the practice. It was found that 56% of participant knew about boosting and 16.7% reported having boosted in the past. However, 48.9% of athletes considered it dangerous, 21.3% felt it was dangerous, and 25.5% thought it was very dangerous [21]. Although the number of athletes who admitted boosting was relatively small, there was a great need for more education about the practice and the dangers of it.
Short-term dangers of AD include increased BP, cardiac arrhythmias, pulmonary edema, cerebral hemorrhage, seizures, retinal hemorrhage, and metabolic problems such as hyponatremia, cardiac arrest, and death. Some of the stimuli used to induce this phenomenon can cause problems such as hydronephrosis, pyelonephritis, and skin infections. In terms of long term issues caused by AD, it is thought that elevated BP could accelerate atherosclerosis [20].
The Positions of the Governing Bodies of Adaptive Sports
Boosting is not prohibited by WADA although it is a method of performance enhancement comparable to other methods, which are on the prohibited. However, it has been prohibited by the International Paralympic Committee (IPC) since the 1990s, initially because of ethical concerns and the performance enhancement of the process. Since then, the IPC has recognized that it can be harmful to athletes and prohibits any athlete from competing while in a dysreflexic state, whether or not it was intentionally induced [22]. The IPC’s Position Statement on AD and Boosting states that “A hazardous dysreflexic state is considered to be present when the systolic blood pressure is 180 mm Hg or above” (IPC Handbook 2009, Chap. 4.3). The current approach is to test an athlete’s BP prior to competition and recheck 10 min later if the systolic BP ≥ 180 mmHg. If it measures ≥180 mm Hg at the recheck, the athlete will be withdrawn from competition. No athlete has so far tested positive for boosting at an IPC sanctioned event [3].
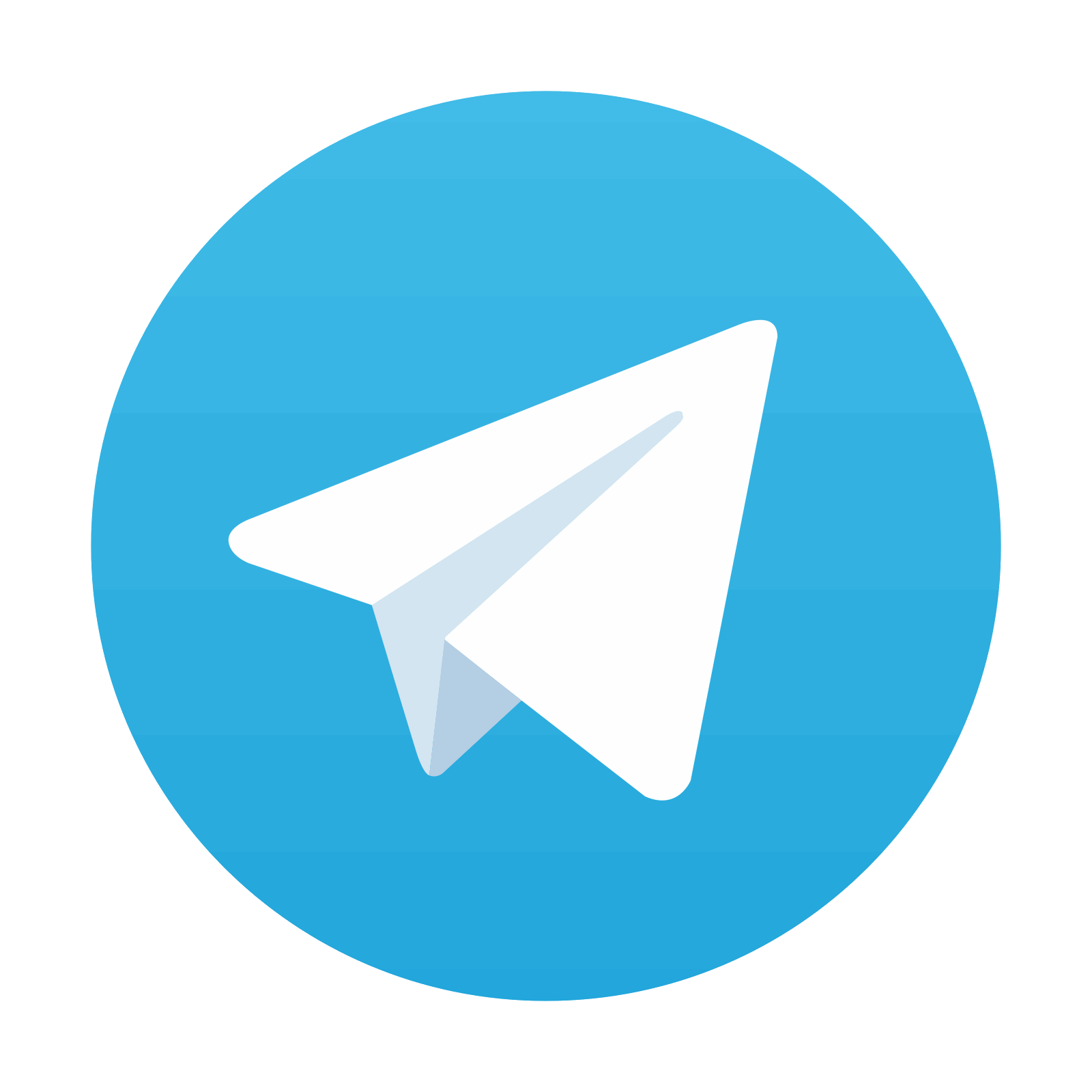
Stay updated, free articles. Join our Telegram channel

Full access? Get Clinical Tree
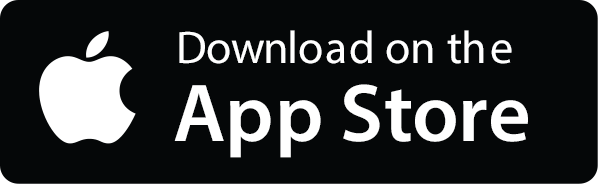
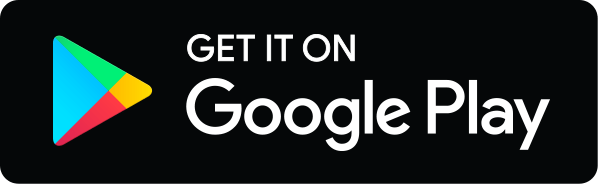