Fig. 27.1
Planning of the acetabular socket using CT image
According Position of the Femur
The future position of the femur (as determined for instance by the location of the greater trochanter) is determined on the frontal view based on the position of the acetabular socket, on the desired lengthening as determined from the scanogram, and on the neck lever arm (femoral offset). This position will determine the level of the femoral cut and assess the correct neck lever arm on the frontal view. However, osteotomy of the greater trochanter may be necessary in cases where extensive lengthening is required, associated to a wrong anteroposterior position of the greater trochanter due to excessive anteversion.
The anteversion angle of the prosthesis neck must be set such that normal gait anatomy can be restored. The normal gait anatomy requires three conditions: (1) foot axis showing 10–20° of external rotation, (2) posterior bicondylar axis perpendicular to the gait direction, (3) anteversion of the femoral neck between 15 and 20° with respect to the bicondylar axis. It has been shown that in most cases of congenital dysmorphism, the upper femur axis, also called helitorsion axis and defined as the axis passing across the longer diameter at the level of osteotomy, is not aligned with the neck axis [30]. This phenomenon is usually not taken into account in standard prostheses. This results in such cases most frequently in an over- or under-correction of the prosthetic anteversion angle, thus preventing from full restoration of the normal gait. By superimposing the three CT views of the osteotomy level (usually above the lesser trochanter), and of the knee and foot levels, it is possible to calculate the correction angle to add (or subtract) to the helitorsion angle such that a final prosthetic anteversion angle of 15–20° is achieved (Fig. 27.2).


Fig. 27.2
Restoration of normal gait anatomy based on the correction of the helitorsion angle (H); AV desired prosthetic neck anteversion, BPA bicondylar posterior axis, alpha correction made in the prosthetic neck between H and AV
The Computer Assisted Design of Custom Hip Stem
The Design of the Intramedullary Section
Contouring
Upon their reception, raw CT data is processed by numerical thresholding such that non bony structures are excluded from the images. Following this “image filtering” step, the design engineer runs an image analysis program to select both the internal and external contours of the bone section on each femoral CT slice. This contouring process is normally performed fully automatically, except in the area of the femoral neck and in cases of important artefacts on CT images for which manual intervention is needed.
Matching CT and X-Ray Data
Anatomical landmarks on the diseased joint must be first registered. These landmarks will be used later for the definition of the osteotomy. The summits of the greater and lesser trochanters and the femoral head summit are localized and indicated on the x-ray frontal view.
The next step in the design process consists in superimposing the CT and x-ray data on the same image file. For this, frontal and lateral radiographic views of the diseased hip are first digitalized using an x-ray compatible image scanner. The contouring data obtained during the previous step is numerically added to the digitalized x-ray views. A manual fitting of the two types of images is then performed independently on the frontal and lateral view.
Definition of Osteotomy Orientation
Once merging of CT and x-ray data is completed, osteotomy directions are calculated and added to the image file. The level of the osteotomy is defined such that neck length, optimized stability in rotation and optimized bone stock preservation are taken into account.
Generation of the Initial Stem and Extraction
Based on the internal contouring data, the design software uses then numerical interpolation procedures to generate a first stem shape limited to the intramedullary zone. However, the very precise reproduction of the femoral internal contour on this first draft makes it most often useless without modifications, as local protrusions and depressions at the bone surface would prevent any movement of the stem within the femur. It is therefore necessary to simulate numerically the extraction of the stem from the femur. This is done by successive iteration steps during which the stem is extracted incrementally by rotations and translations in the three main orthogonal axes. During each iterative step, incremental stem shape modifications are performed by the software in order to allow the extraction while maintaining the contact zones necessary for an optimized mechanical support of the stem in the femur. Optimized support is sought in medial, lateral, and anterior metaphyseal areas. At the end of the simulation, a new, modified version of the stem is obtained that can be implanted into the femur with a very restricted degree of freedom for the insertion path.
Final Adaptations
At the end of the extraction process, numerical integration of the new stem shape in the CT data is performed. It enables the design engineer to view each CT section together with the corresponding stem section (“composite” view, Fig. 27.3). By switching to the editor mode of the software, the engineer can also perform a final design “tune up”, during which he can still implement slight modifications on each stem section to further optimize bone-prosthesis adjustment.


Fig. 27.3
Composite CT views with integration of stem sections
Stem Insertion and Resistance Simulation
The final step in the design of the intramedullary part of the femoral stem consists in simulating a subsidence of the stem in the femoral canal in order to be sure that the stem is at worst in contact with the cortical bone in this shifted position.
A numerical 3-point bending simulation test is then performed to validate the mechanical resistance of the stem.
The Design of the Extramedullary Section
The design of the extramedullary part of the stem is performed as well in the frontal and lateral as in the sagittal plane. The determination of the anteversion angle of the prosthesis neck taking into account the correction for helitorsion, has already been explained earlier. With the intramedullary stem integrated in the x-ray frontal view, the design engineer calculates the optimized combination of CCD angle, neck length and head offset such that the planned rotation center and lever arm are respected (Fig. 27.4).


Fig. 27.4
Composite x-ray frontal view with integration of intra- and extramedullary stem sections
The Prosthesis Validation
The pre-operative planning of a custom made prosthesis is performed together by the surgeon and the design engineer at Symbios. Following the planning, the design of the stem is done entirely by the design engineer. Therefore the final design must be validated by the surgeon before the fabrication of the prosthesis can be launched. A complete patient file is provided to the surgeon including the CT composite view, the normal gait restoration scheme, and the x-ray frontal (with osteotomy parameters, Fig. 27.5) and lateral view with the designed stem.


Fig. 27.5
The x-ray frontal view with osteotomy parameters
The Stem Manufacturing
Stem Machining
Upon validation of the stem design and pre-operative planning by the surgeon, the fabrication of the prosthesis can proceed. For this, the stem CAD data is transferred into a Computer Assisted Machining (CAM) software that pilots a 5-axis milling machine. In parallel, a compactor with a smooth surface is machined with the same design as the stem itself. It is used for compaction of the cancellous bone before the stem itself is introduced (Fig. 27.6).


Fig. 27.6
Example of porous coated custom made prosthesis together with the corresponding “rasp” for compaction of cancellous bone and implant preparation
Materials and Coatings
Wrought Ti6Al4V titanium alloy is used most of the time for the fabrication of the stem. In very few cases, stainless steel stems are produced upon request of the surgeon. The rasp itself is made out of wrought stainless steel. After machining, the prosthesis stem undergoes a surface plasma spray coating procedure which can vary from one stem to the other, depending again on the surgeon’s request. In most cases a first layer of ~300 μm of porous titanium followed by a ~80 μm layer of porous hydroyapatite (HA) are coated on the intramedullary section of the stem, from the osteotomy level down to the distal level at which the transition from an elliptic to a circular section takes place.
Sterilization and Packaging
The final steps in the production of the prosthesis are the gamma sterilization and the final packaging procedure which is performed in clean room conditions.
The Twenty-Years Clinical Use of Individual Cad for Cementless Hip Arthroplasty
The Rationale for the Use of Custom CAD for Hip Arthroplasty
The self-preservation of the dense cancellous bone compacted towards the inner cortical femur is obtained by the use of a smooth compactor of identical intra and extramedullary shape than the final prostheses. The preservation of this cancellous bone is mandatory for secondary biologic fixation to the hydroxyapatite (HA) covering the final prosthesis. Both the clinical and the radiological evaluation of different stem coatings lead us to move from a proximal HA to a full HA coating [31].
The solution provided by three-dimensional designed custom neck to face excessevely anteverted upper femur often encountered in dysmorphic or dysplasic hips was a retroversion of the custom neck to restore an appropriate anteversion of 15–20° on the knee condylar plane [32, 33]. In such situations some authors have described the association of a derotational osteotomy to a conventional stem or the use of modular necks [13].
We studied by X-rays and CT the morphology of 83 hips from 69 European adults with osteoarthritis following developmental dysplasia of the hip (DDH) [17]. A cohort of 310 primary osteoarthritic hips was used as a control group. According to the classification [34] of dysplastic hips were graded as class I, 27 as class II and 23 as class III or IV. The intramedullary femoral canal had reduced mediolateral and anteroposterior dimensions for all groups compared to primary osteoarthritis control group. The individual variability was important when measuring the CT-scan canal flare index, despite the subluxation class considered. The femoral neck shaft angle was increased only for femora of class II. The proximal femur had more anteversion in all the DDH groups, ranging from 2 to 80°. The mean anteroposterior diameter of the true acetabulum for classes III/IV was smaller compared to primary osteoarthritis. The severity of the dysplasia may influence the difficulty of achieving hip center location in the true acetabulum but does not correlate with the degree of individual femoral anteversion.
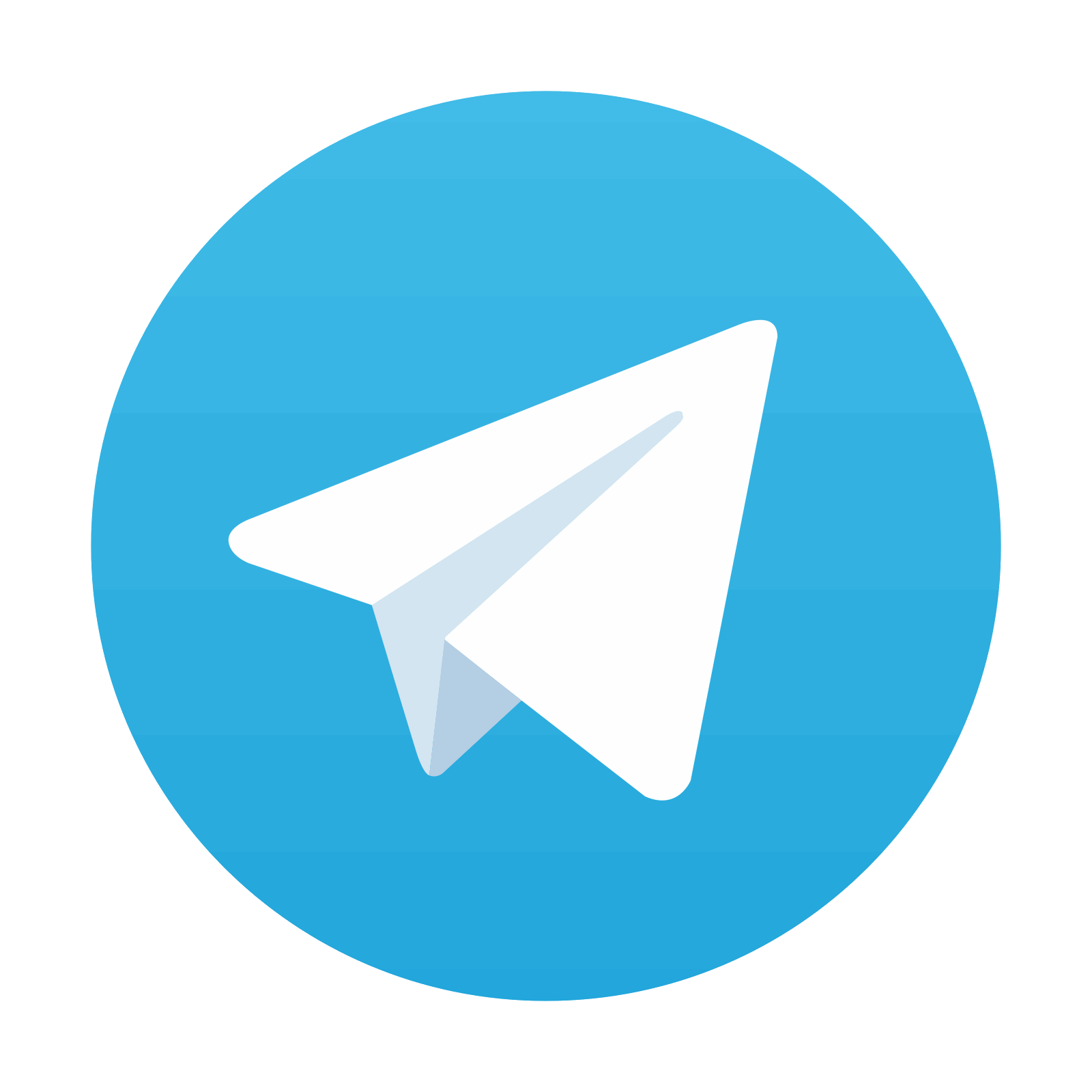
Stay updated, free articles. Join our Telegram channel

Full access? Get Clinical Tree
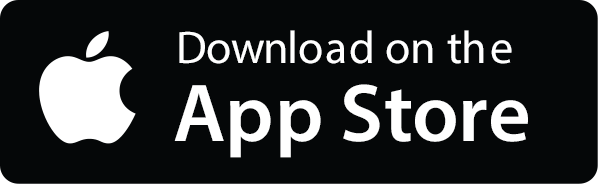
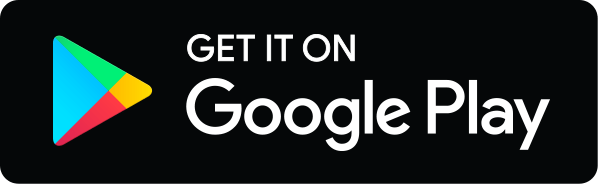