13 Complications of Instrumentation: Is There a Higher Complication Rate in Placing Instrumentation via a Minimally Invasive Technique Compared with an Open Technique? Open exposure of the dorsal spinal elements is the gold standard for placement of posterior instrumentation, given that it facilitates identification of anatomic landmarks and trajectories for safe screw placement. However, open techniques require significant paraspinal muscle dissection, resulting in frequent postoperative pain, delayed mobilization, and muscle atrophy. Minimally invasive approaches utilizing serial dilators, tubular retractors, and image guidance have been developed to place posterior instrumentation throughout the spine. Minimally invasive surgery (MIS) techniques cause less immediate postoperative pain and allow faster recovery, but it is controversial whether they come at the cost of screw accuracy, construct strength, fusion rate, and long-term outcome. Posterior spinal instrumentation is placed to immobilize the spine and facilitate fusion during surgery for degenerative, traumatic, oncologic, rheumatologic, or structural disease. Atlantoaxial stabilization is indicated for C1–C2 instability and can be achieved through a variety of techniques including C1–C2 transarticular screws (TASs) and screw–rod constructs (C1 lateral mass screw with C2 pedicle, pars, or laminar screw). Subaxial posterior cervical fixation is most commonly achieved using lateral mass screws. Subaxial cervical pedicle screws can also be placed and have greater pullout strength, but placement is technically demanding with a higher risk of vertebral artery or nerve root injury. Subaxial cervical fixation using percutaneous transfacet screws has also been described. In the present era, stabilization of the thoracic and lumbar spine is typically achieved with pedicle screw–rod constructs, although pedicle or laminar hooks and wiring techniques may be used in cases with poor pedicle anatomy or as salvage strategies. Pedicle screws may be placed using a traditional open approach or percutaneously with anteroposterior (AP)–lateral fluoroscopy or computer-aided navigation. Lumbar translaminar facet screws and transfacet-transpedicular screws may also be placed percutaneously, often to supplement an anterior interbody fusion. Open placement of posterior instrumentation requires significant paraspinal muscle dissection, leading to frequent postoperative pain, delayed mobilization, blood loss, and muscle atrophy. Less soft-tissue trauma occurs when instrumentation is placed with serial dilators and tubular retractors. As a result, MIS techniques are associated with less postoperative pain, shorter hospital stays, and faster return to activities of daily living. Percutaneous screw placement also spares the mid-line fascia and ligaments, and the preservation of this posterior tension band may potentially lower the risk of adjacent segment disease. Percutaneous pedicle screws, when properly placed, minimize dissection and exposure of the facet joint and could reduce the risk of adjacent segment disease. The advantages of open surgery are primarily its familiarity and versatility. Virtually all contemporary spine surgeons are intimately familiar with the anatomy of open posterior spinal exposures and the techniques of posteriorly placed spinal instrumentation. Utilization of anatomic landmarks can help guide implant placement, particularly in cases with limited fluoroscopic visualization. Combining anatomic cues with limited fluoroscopy also fosters a balanced approach to patient safety and radiation exposure to the surgical team. Given that most of these techniques have been commonplace for up to two decades, the nuances and pitfalls have become well understood and reasonably documented. As such, the risks and expectations associated with these procedures can be communicated to patients with a fair amount of confidence. In addition to their familiarity, open techniques are versatile. Standard midline approaches permit bilateral exposure to the lateral masses and pedicle entry sites. Levels can be easily added if planned fixation sites are compromised due to screw cutout or poor purchase. Alternative midline fixation techniques, such as C1–C2 sublaminar cables or C2/C7 translaminar screws, can also be utilized for bailout or augmentation. If mid-line laminectomy is not necessary, then the available bony surfaces for fusion are substantially greater. Conversely, because many patients undergoing posterior instrumented fusion also require decompression, the open midline approach is often selected anyway, and as a consequence will be utilized more frequently throughout most surgeons’ careers. Volume fosters familiarity and familiarity has the advantages noted above. A 35-year-old woman presents with chronic progressive neck pain and 6 months of left hand weakness and paresthesias. The patient has mild left hand intrinsic weakness with a positive left Hoffman’s sign. Computed tomography (CT) demonstrates a smooth dens separated from the body of the axis, consistent with os odontoideum ( Fig. 13.1 (a) Sagittal CT and (b) T2-weighted MRI demonstrate os odontoideum associated with T2 signal abnormality. Lateral cervical spine X-rays demonstrate increased atlantodental interval on (c) flexion but not on (d) extension. The patient is positioned prone in a Mayfield head holder with the neck extended to bring the atlas and axis in normal alignment.1 A 2.5-cm paramedian incision is made overlying the C2 spinous process 2 cm lateral to midline. The fascia is incised, and under fluoroscopic guidance serial dilators are docked onto the lamina of C2. An expandable tubular retractor is placed over the largest dilator and fixed to the bed ( The patient is positioned similarly as described above. The shoulders can be taped to the table to eliminate redundant skin folds in the area of the exposure, if necessary. A midline skin incision is made from just above the foramen magnum to just below the tip of the C2 spinous process. Verification of the mid-line can be done by finger palpation before incising the nuchal fascia, as this minimizes bleeding. Initial exposure proceeds along the top of the C2 spinous process and lamina bilaterally, extending distally toward the C2–C3 facet joint and cephalad to expose the medial boarder of the C2 pedicle. Exposure of the bottom of the occiput in the midline can help establish the ventral depth of the exposure, as the tubercle of the atlas is not as easily palpated initially. Once the tubercle of the atlas can be palpated, subperiosteal dissection can proceed laterally to each side, up to 1.5 cm without risk to the vertebral artery. Gelfoam and bipolar electrocautery can be used to help control bleeding from the epidural venous plexus while exposing the C1 lateral mass and C1–C2 facet joint. The C2 nerve root can be retracted caudally with a Penfield or cut to aid with this exposure. C1 lateral mass and C2 pedicle screws are then placed with standard entry points and trajectories with the aid of fluoroscopy and direct visualization (i.e., a Penfield or blunt nerve hook on the superomedial boarder of the C2 pedicle). If necessary, sublaminar wiring under the atlas can aid in the reduction of an anteriorly displaced dens fragment. A variety of C1–C2 wiring constructs or C2 translaminar screws can also be utilized if one of the originally selected fixation points becomes compromised intraoperatively. Decortication of the C1–C2 facets and, in selected cases, the posterior arch of C1 and C2 lamina provides adequate surface area for bony fusion. Copious irrigation of the wound, interval removal of the retractors, use of vancomycin powder in the wound bed, and meticulous closure all contribute to minimizing risk of surgical site infection. Fig. 13.2 Intraoperative photographs show placement of (a) expandable tubular retractor allowing exposure of (b) C1–C3 posterior spinal elements. (c) C1 lateral mass and C2 pedicle screws are placed through the retractor using standard starting points, trajectories, and fluoroscopic guidance. (d) The screws are secured to a rod, and (e) appropriate hardware placement is confirmed on postoperative imaging. Posterior segmental instrumentation may be placed throughout the spine using percutaneous or tubular retractor–based techniques. Atlantoaxial fixation may be achieved using C1 lateral mass and C2 pedicle/pars screws placed through tubular retractors. Subaxial cervical stabilization may performed using lateral mass screws through tubular retractors or using percutaneous transfacet screws. Cervical pedicle screws may also be placed percutaneously. For thoracic and lumbar posterior stabilization, percutaneous pedicle screws may be placed using standard AP– lateral fluoroscopy or computer-guided stereotactic navigation. Lumbar translaminar facet and transfacet-transpedicular screws can be placed percutaneously or with a small mini-open exposure. There are many single-cohort studies reporting the feasibility and safety of these techniques. Several retrospective studies assess the complications and outcomes of MIS approaches compared to open stabilization. There are few prospective studies comparing MIS and open techniques ( There are no level I studies available. There are no level II studies available. Joseffer et al1 demonstrated that atlantoaxial fixation was technically feasible using a tubular retractor–based approach. C1 lateral mass and C2 pedicle screws were placed in one patient without complication, and early fusion was evident by 3 months. Taghva et al2 reported two patients undergoing C1 lateral mass and C2 pedicle screw placement with expandable tubular retractors. Neither patient had any complication from surgery, and no breaches were noted on postoperative CT. Both patients had significant improvement in pain scores and had good fusion at 2-year follow-up. The advantages of this approach over open atlantoaxial fixation include preservation of the posterior tension band, less blood loss, and less postoperative pain. Holly et al3 reported six patients undergoing C1–C2 fusion using tubular retractors (five for type II dens fractures, one for os odontoideum). No patients suffered any complication, and solid fusion occurred in all patients (average follow-up time: 32 months). There was no hardware malposition found in the four of six patients undergoing postoperative CT. The authors noted that the techniques for placing instrumentation (entry points, trajectories) and the method of obtaining fusion do not substantially differ between open and tubular retractor techniques. As such, the long-term results would be expected to be similar, although the study did not have a comparison group of open patients. Wei at el4 performed a comparison study of MIS versus open C1–C2 fusion, with 22 atlantoaxial instability patients undergoing an MIS endoscopic approach and 22 undergoing open treatment. The two groups were similar in baseline characteristics, but the MIS group had lower blood loss, operation time, and incision length. There was no difference in foramen transversarium violation between the two groups. The patients were followed for a mean 25 months, and bony fusion without screw loosening or breakage was achieved in all cases. There was no significant difference in JOA (Japanese Orthopaedic Association) scores between the two groups at last follow-up. The authors concluded that MIS atlantoaxial fusion is equally effective as an open approach, with no increased risk of complications and less soft-tissue injury. There are no level I studies available. There are no level II studies available. Percutaneous screws can be placed through the facet joints of the cervical spine to achieve stabilization without rod placement. Ahmad et al5 reported three patients undergoing percutaneous cervical transfacet fixation without complication following multilevel anterior fusion. This study had no comparison group or long-term follow-up, and the authors noted that this technique is limited to supporting an anterior fusion until percutaneous bony fusion methods are developed. Miyanji et al6 did a biomechanical study of human cervical spines, comparing transfacet versus lateral mass fixation (n = 8 in each group) in flexion/extension, lateral bending, and axial torsion. The transfacet group (with or without connecting rods) had similar biomechanical stability compared to the lateral mass group. On the other hand, some biomechanical data suggest that facet screws provide inferior stabilization once the spine has been destabilized by corpectomy. Lee et al7 assessed the strength of transfacet versus lateral mass fixation following multilevel corpectomy. Corpectomy was performed in 16 cadaver cervical spines randomized to lateral mass or transfacet screw constructs. Transfacet screws were found to provide inferior spinal immobilization and were associated with increased graft loading. Subaxial lateral mass screws may also be placed with MIS technique. Mikhael et al8 wrote a technique article describing lateral mass screw placement through tubular retractors. There is no report of complications, fusion rate, or patient outcomes. Fong and Duplessis9 described the feasibility of a tubular retractor system to achieve lateral mass fixation in two trauma patients. There were no reported complications. However, the screws were placed to supplement an anterior fusion, and no long-term follow-up was reported. Wang and Levi10 reviewed 18 patients treated with lateral mass fixation using a tubular retractor. Postoperative CT showed no bony violations except for screws with bicortical purchase. All cases achieved fusion. Pedicle screws may be inserted into the subaxial cervical spine using open or percutaneous approaches. Schaefer et al11 demonstrated the feasibility of percutaneous cervical pedicle screw placement but revealed its limited accuracy. In total, 72 percutaneous cervical pedicle screws were placed, but only 76% had no bony violation on postoperative imaging. Still, there were no complications of posterior instrumentation, and no screw revision was required. There was no comparison to open pedicle screw placement. Jiang et al12 performed a randomized controlled trial comparing percutaneous pedicle screw fixation (n = 31) with an open paraspinal muscle-splitting approach (n = 30) in the management of neurologically intact patients with thoracolumbar burst fractures. The percutaneous group had significantly less blood loss, operative time, and length of hospital stay, as well as superior pain control and function at 3 months. No screws required revision, and there was no difference in complications between the two groups. On the other hand, open surgery was associated with superior correction of kyphosis and restoration of vertebral body height. There were no significant differences in long-term clinical outcome. Studies of paraspinal muscle anatomy and function have demonstrated less muscle injury with percutaneous compared to open techniques. Li et al13 assessed paraspinal muscle injury via electromyogram (EMG) and CT among 33 patients divided into percutaneous and open pedicle screw groups. Both procedures caused damage to paraspinal muscle, but less muscle injury was noted in the percutaneous group, mostly in the short term. King14 prospectively followed 19 pedicle screw patients (11 open, 8 percutaneous) for a mean 21 months, assessing trunk extension strength and multifidus muscle cross-sectional area on axial imaging. The percutaneous patients had lower blood loss, less postoperative pain, and superior trunk extension strength (although the latter was not statistically significant). Postoperative versus preoperative multifidus muscle cross-sectional area significantly decreased in the open group (p = 0.003) but not in the percutaneous group (p > 0.05). Grossbach et al15 prospectively followed 38 patients undergoing surgery for flexion-distraction injury (27 with open pedicle screws, 11 with percutaneous screws). There was no significant difference in presenting ASIA (American Spinal Injury Association) scores or alignment. The MIS group had a shorter operative time and lower blood loss. There was no difference in perioperative complications between the groups. There were no cases of construct failure, although the follow-up period was short. In a similar report, Huang et al16 compared percutaneous with traditional open pedicle screw fixation in neurologically intact patients with thoracolumbar burst fractures (n = 30 patients in each group). The percutaneous group had a significantly lower blood loss, length of hospital stay, and immediate postoperative pain (within 3 months of surgery). At last follow-up (average 2 years), there was no significant difference in kyphosis angle, vertebral height, pain as measured by VAS (visual analog scale), or disability as measured by ODI (Oswestry Disability Index). Percutaneous pedicle screws are traditionally placed with AP–lateral fluoroscopy, but computer-assisted navigation techniques have also been developed. Yang et al17 did a multicenter prospective study comparing 42 patients undergoing navigated percutaneous pedicle screw placement with 34 patients undergoing percutaneous screw placement with conventional fluoroscopic technique. Fluoroscopy time, time to place guidewire, and pedicle breach rate (3.0 vs. 7.2%) were superior in the guidance group. There was no comparison with open screw placement. Several retrospective studies suggest that percutaneous pedicle screw fixation achieves similar long-term outcomes when compared to open screw placement, with less blood loss and postoperative pain and no increase in complications. Lee et al18 compared 32 patients undergoing percutaneous fixation with 27 patients undergoing open fixation for thoracolumbar burst fractures. The percutaneous group had significantly less blood loss and operative time, as well as a significantly lower VAS score and superior LBOS (low back outcome score) at 3 and 6 months. At last follow-up, there was no significant difference in correction of kyphosis or clinical outcome. Kotani et al19 did a retrospective review of 80 patients undergoing single-level posterolateral fusion for degenerative spondylolisthesis. Compared to open patients, the percutaneous group had significantly less blood loss and postoperative pain. The ODI and Roland–Morris Questionnaire (RMQ) scores were lower in the percutaneous group at 3, 6, 12, and 24 months postoperatively. The fusion rate was similar between the two groups (98 vs. 100%). There were no major complications of screw placement. A few reports have associated percutaneous pedicle screw placement with certain complications. Mobbs and Raley20 retrospectively assessed for anterior breaches with the K-wire during placement of 525 percutaneous screws. There were seven anterior breaches of the K-wire; two of these patients developed a retroperitoneal hematoma with ileus but neither required blood transfusion or surgical intervention. The authors concluded that the anterior breach with the K-wire is rare and generally of no long-term consequence, but surgeons must take care to avoid this event because catastrophic vascular or bowel injury are potential complications. Another complication that some reports have associated with percutaneous pedicle screw fixation is violation of the adjacent unfused facet joint by the superior screw of the construct. Jones-Quaidoo et al21 compared the incidence of superior facet disruption in 66 patients undergoing percutaneous fixation versus 66 undergoing open fixation. The percutaneous cohort was younger than the open group but otherwise well-matched. A significantly higher percentage of screws placed in the percutaneous group were in the facet joint compared to the open group (13.6 vs. 6%). Babu et al22 also compared rates of superior facet violation during 126 open versus 153 percutaneous lumbar pedicle screw cases for degenerative disease. On postoperative CT, the percutaneous screws had a significantly higher rate of superior facet violation. Other reports refute the claim that percutaneous pedicle screws are less accurate than open screws. Yson et al23 compared the rate of superior facet violation among 245 open lumbar pedicle screws versus 125 percutaneous screws placed with three-dimensional CT-based guidance (O-arm). Facet violation by the cranial-most screws occurred significantly more frequently in the open group compared to the percutaneous group (26.5 vs. 4%). Oh et al24 also did a retrospective cohort study assessing the accuracy of percutaneous versus open pedicle screw fixation in lumbosacral fusion (126 open patients, 111 percutaneous patients). Postoperative CTs were reviewed to assess the incidence and severity of breach. The incidence and severity of pedicle perforation were not significantly different between the two groups. Lau et al25 compared the incidence of superior facet violation in percutaneous (142 patients) versus open (140 patients) pedicle screw placement during transforaminal lumbar interbody fusion. The rate of superior facet violation was similar in both groups. There is some evidence that accuracy of percutaneous pedicle screws can be improved when intraoperative computer-based navigation is used instead of conventional fluoroscopic technique. Houten et al26 retrospectively compared 141 percutaneous screws placed using standard fluoroscopic technique with 205 screws placed using O-arm computer-based navigation. The rate of pedicle perforation was 12.8% in the fluoroscopy group versus 3% in the O-arm group (p < 0.001). Screw placement was also faster in the O-arm group. Fraser et al27 compared 29 patients undergoing percutaneous screw placement using three-dimensional navigation, with 13 undergoing screw placement using standard fluoroscopy. There was a significantly lower rate of pedicle perforation (p = 0.04) in the navigated group. Nakashima et al28 retrospectively assessed percutaneous screw accuracy with conventional fluoroscopic technique (n = 150) versus three-dimensional fluoroscopy-based image guidance (Iso-C) (n = 150). On postoperative CT, 7.3% of the screws placed with Iso-C were outside the pedicle in comparison to 15.3% placed with fluoroscopy alone (p < 0.05). There are no level I studies available. Tuli et al29 did a prospective cohort study comparing long-term outcomes in patients treated with translaminar facet screw fixation (n = 40) versus open pedicle screw fixation (n = 37) for degenerative lumbar disease. The translaminar facet screw group had a shorter length of stay, blood loss, and fewer perioperative complications. Nevertheless, facet screw patients had a greater need for reoperation for nonunion (7 of 40 vs. 1 of 37). Biomechanical studies suggest that translaminar facet fixation provides comparable strength to pedicle screw fixation following interbody fusion. Hou et al30 compared the stiffness of translaminar facet versus pedicle screw constructs following two-level anterior lumbar interbody fusion (ALIF). A two-level ALIF was performed in 12 fresh human lumbar spines followed by placement of either pedicle screws or translaminar facet screws. There was no significant difference in stiffness during flexion, extension, lateral bending, or rotation. Burton et al31 also compared translaminar facet versus pedicle screw fixation in 10 cadaveric specimens with an interbody graft. Translaminar facet screw fixation was equivalent to bilateral pedicle screw placement in compressive loading, flexion–extension, lateral bending, and axial torque. Transfacet screw constructs have been shown to provide some degree of stabilization but are not as rigid as pedicle screw constructs. Wang et al32 performed flexibility testing in fresh-frozen calf spines following two-level ALIF with either pedicle screws, translaminar facet screws, or transfacet screws. In lateral bending, pedicle and translaminar facet screw constructs had similar strength and were superior to transfacet screw constructs. In extension, pedicle screw constructs were more rigid than the other two. These results suggest that pedicle screws provide stronger fixation than translaminar facet screws, which in turn provide stronger fixation than transfacet screws. Similarly, Agarwala et al33 studied two groups of seven human cadaver spines instrumented with either pedicle screws or transfacet screws, and examined strength in primary and circumferential fixation. Transfacet screws provided similar fixation in flexion–extension but inferior stiffness in lateral bending and rotation. These differences were minimized when anterior instrumentation was included. While not providing the three-column fixation of pedicle screws, several studies have shown that translaminar facet screws and transfacet screws provide sufficient immobilization to facilitate successful ALIF. Amoretti et al34 retrospectively assessed 182 percutaneous transfacet and 56 translaminar facet screws. All were placed with fluoroscopic guidance with one attempt following ALIF. Radiographic fusion occurred within 1 year in all patients despite one translaminar facet screw failure. Shim et al35
13.1 Introduction
13.2 Indications of Posterior Instrumentation
13.3 Advantages of Minimally Invasive Surgery
13.4 Advantages of Open Surgery
13.5 Case Illustration
Fig. 13.1a). No cord compression is seen on magnetic resonance imaging (MRI) with the neck in neutral position, but subtle T2 signal abnormality is present at C2 (
Fig. 13.1b). On lateral X-ray, an increased atlantodental interval is present on flexion but not on extension (
Fig. 13.1c,d).
13.6 Surgical Technique in Minimally Invasive Surgery
Fig. 13.2a). The retractor is then expanded, with the cephalad blade exposing the C1 lateral mass and the caudal blade exposing the C3 lateral mass. The C2 and C3 lateral masses are dissected with monopolar cautery (
Fig. 13.2b). The C2 nerve root is coagulated with bipolar cautery and cut to expose the C1–C2 facet joint and C1 lateral mass. C1 lateral mass and C2 pedicle screws are placed using standard entry points and trajectories with fluoroscopic guidance (
Fig. 13.2c,d). The C1–C2 joint is decorticated and filled with allograft chips and demineralized bone matrix. The screws are secured to a rod, and the procedure is repeated contralaterally (
Fig. 13.2e).
13.7 Surgical Technique in Open Surgery
13.8 Discussion of Minimally Invasive Surgery
Table 13.1;
Table 13.2;
Table 13.3).
13.8.1 Level I Evidence in Minimally Invasive Atlantoaxial Stabilization
13.8.2 Level II Evidence in Minimally Invasive Atlantoaxial Stabilization
13.8.3 Level III and IV Evidence in Minimally Invasive Atlantoaxial Stabilization
13.8.4 Level I Evidence in Minimally Invasive Subaxial Cervical Stabilization
13.8.5 Level II Evidence in Minimally Invasive Subaxial Cervical Stabilization
13.8.6 Level III and IV Evidence in Minimally Invasive Subaxial Cervical Stabilization
13.8.7 Level I Evidence in Minimally Invasive Thoracolumbar Pedicle Screw Fixation
13.8.8 Level II Evidence in Minimally Invasive Thoracolumbar Pedicle Screw Fixation
13.8.9 Level III and IV Evidence in Minimally Invasive Thoracolumbar Pedicle Screw Fixation
13.8.10 Level I Evidence in Minimally Invasive Lumbar Translaminar Facet/Transfacet Fixation
13.8.11 Level II Evidence in Minimally Invasive Lumbar Translaminar Facet/Transfacet Fixation
13.8.12 Level III and IV Evidence in Minimally Invasive Lumbar Translaminar Facet/Transfacet Fixation
Stay updated, free articles. Join our Telegram channel

Full access? Get Clinical Tree
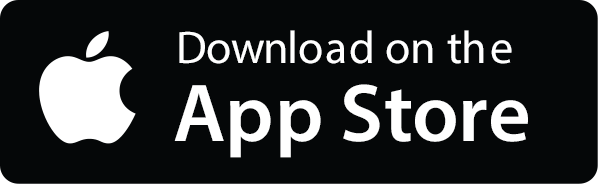
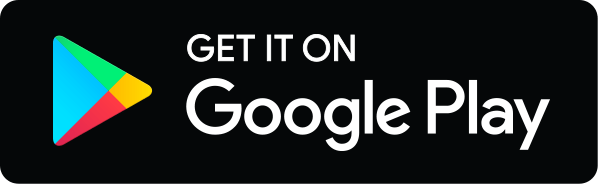