Crystal arthropathies are among the most common causes of painful inflammatory arthritis. Gout, the most common example, has been associated with cardiovascular and renal disease. In recent years, evidence for these associations and those involving other comorbidities, such as the metabolic syndrome, have emerged, and the importance of asymptomatic hyperuricemia has been established. In this review, an update on evidence, both experimental and clinical, is presented, and associations between hyperuricemia, gout, and several comorbidities are described. Causality regarding calcium pyrophosphate arthropathy and associated comorbidities is also reviewed.
Key points
- •
Recent evidence has shown that asymptomatic hyperuricemia, as well as hyperuricemia in patients with gout, plays a significant role in the development of cardiovascular comorbidities.
- •
In addition to an already proven association between hypertension and hyperuricemia, interventional trials are showing a positive effect of urate-lowering therapy in early stages of hypertension in young individuals.
- •
An association between hyperuricemia and other cardiovascular diseases such as coronary heart disease, congestive heart failure, and stroke is still not clear.
- •
A link between hyperuricemia, insulin resistance, and the metabolic syndrome has been shown by fructose-fed animal models and may explain the association between 2 overlapping and increasing diseases.
- •
Hyperuricemia is associated with an increased risk of chronic kidney disease, but the use of urate-lowering therapy in these patients is still not clear.
- •
Evidence regarding calcium pyrophosphate arthropathy and associated comorbidities is still scarce and not conclusive.
Crystal arthropathies are among the most common cause of arthritis worldwide. Of these arthropathies, gout represents the highest known burden of crystal-induced arthritis and is likely the most common type of inflammatory arthritis in adults in the United States. Calcium pyrophosphate arthropathies, initially described as pseudogout, and other calcium crystal arthropathies are less commonly recognized than gout. Although initially observed only as a painful inflammatory arthropathy, in recent years, more evidence has been building up the case for an association between gout and hyperuricemia and important cardiovascular-metabolic conditions. This article presents an updated review of the evidence for these associations, as well as comorbidities associated with calcium crystal arthropathies.
Comorbidities associated with hyperuricemia and gout
Hyperuricemia, defined as a serum urate (SU) concentration higher than the point of saturation of 6.8 mg/dL or more, is the most common biochemical abnormality associated with the development of gout, but it is not a sufficient causative factor. Individuals in whom SU concentrations are increased above saturation levels but have not developed clinical manifestations of gout are considered to have asymptomatic hyperuricemia. Data from the US National Health and Nutrition Examination Survey (NHANES) 2007–2008 study estimated a gout prevalence of 3.9% (5.9% for men; 2.0% for women), but a higher hyperuricemia prevalence of 21.4% (21.2% for men; 21.6% for women). In the following sections, the experimental and epidemiologic evidence linking gout and various comorbidities and their complex interrelationships is summarized.
Cardiovascular Disease
Urate and the endothelium: laboratory and animal studies
In vitro studies that used urate concentrations similar to in vivo levels have shown several potential vascular effects. These effects include suppression of nitric oxide (NO) levels, increased platelet-derived growth factor expression, local thromboxane production, and cyclooxygenase 2 stimulation, as well as induction of endothelial proliferation, angiotensin II production, and increased markers of oxidative stress. The key role of the renin-angiotensin system (RAS) was proved by the reversibility of these effects by adding captopril or losartan. Other significant in vitro observations include the increased production of endothelin 1, a powerful vasoconstrictor, on human aortic smooth muscle cells and cardiac fibroblasts under different urate concentrations. All of these effects are facilitated by the entry of urate to vascular smooth muscle cells via the urate anion transporter 1 (URAT-1), an integral membrane protein that serves as a urate transporter and was initially described in afferent renal arterioles.
In vivo animal models have also supported data from in vitro studies. Hyperuricemia was induced in rats through the administration of oxonic acid, a uricase inhibitor, which led to renal vascular disease characterized by cortical vasoconstriction, afferent arteriolar swelling, and glomerular hypertension. Partial attenuation of these abnormalities was obtained through the administration of the nonreversible xanthine oxidase inhibitor, febuxostat. Other animal models supported these observations and have also shown that although early hypertension can be corrected with SU reduction, after prolonged hyperuricemia, urate reduction does not translate into control of blood pressure and avoidance of arteriolar thickening. Prolonged hyperuricemia results in an irreversible sodium-sensitive urate-insensitive hypertension. These observations have pointed to a 2-stage model, with an early hypertension mediated by increased renal renin activity and reduction of circulating plasma nitrates, and a later irreversible phase secondary to an altered intrarenal vascular architecture ( Fig. 1 ).
Hypertension
In 1999, a study of the Framingham cohort reported an association between hyperuricemia and hypertension, which has been confirmed by other epidemiologic studies in different populations. Recently, NHANES 2007–2008 analyzed the prevalence of gout, hyperuricemia, and comorbidities in noninstitutionalized adults. Hypertension was present in 74% with gout, and in 47% with hyperuricemia (defined as an SU >7.0 mg/dL for men and >5.7 mg/dL for women) but no history of gout, compared with a population-estimated prevalence of 24% among normouricemic patients. Prevalence among the population with gout with and without hyperuricemia was 77.7% and 70.8%, respectively, higher compared with individuals with asymptomatic hyperuricemia. The prevalence of hypertension was significantly higher among individuals in the highest SU category (SU ≥10 mg/dL) compared with those in the lowest SU category (SU <4 md/dL).
A meta-analysis that pooled 11 studies showed a significantly increased risk ratio for incident hypertension of 1.41 (95% confidence interval [CI] 1.23–1.58) among individuals with hyperuricemia, after adjusting for traditional risk factors, including age, body mass index (BMI), and alcohol and tobacco use. This risk appeared more pronounced in younger individuals and women. An increased pooled relative risk (RR) for incident hypertension of 1.13 (95% CI 1.06–1.20) per each mg/dL increase in SU was calculated from 6 studies. Another meta-analysis including 8 prospective studies also reported an increased risk, pooled RR 1.55 (95% CI 1.32–1.82), for hypertension when comparing the highest quartile with the lowest one of SU. However, the analysis presented showed significant heterogeneity ( P <.05).
Most interventional studies come from the adolescent or pediatric population. A relationship between primary hypertension and high SU levels has been observed even at concentrations less than the supersaturation level of 6.8 mg/dL, and 1 study reported increased SU levels (>5.5 mg/dL) in up to 90% in children with primary hypertension. A small randomized, controlled, crossover trial of 30 treatment-naive adolescents (11–17 years old) with stage I hypertension and hyperuricemia randomized individuals to receive oral allopurinol 200 mg daily versus placebo followed by a washout period and then the crossover intervention. After treatment, 20 of 30 participants achieved normal blood pressures compared with 1 participant taking placebo. A randomized, double-blind, placebo-controlled study compared allopurinol with probenecid in prehypertensive obese adolescents. Both treatment arms had a significant decrease in SU and led to a reduction in systolic and diastolic blood pressure of 10.2 and 9.0 mm Hg, respectively. These results suggested that reduction in blood pressure was related to the urate-lowering effect and not to decreased xanthine oxidase activity. A similar study with a small sample of hyperuricemic adults receiving allopurinol 300 mg daily also supported the blood pressure reduction effects of urate-lowering therapy (ULT). Despite these encouraging results, a recent Cochrane review on pharmacotherapy for hyperuricemia and the reduction of blood pressure concluded that evidence is still insufficient to recommend ULT.
Atherosclerosis, coronary heart disease, and peripheral arterial disease
Several mechanisms such as maintenance of a proinflammatory state, promoting a proliferative response in vascular smooth muscle cells, and alterations in the RAS and promotion of hypertensive state may explain the link between urate concentrations and cardiovascular disease. Contributing to this association, increased levels of monosodium urate have also been observed in atherosclerotic plaques. Carotid intima-media thickness (IMT), regarded as a surrogate marker for atherosclerosis, has been shown to have a significant association with SU levels in cohort of healthy postmenopausal women, and also in a different cohort of hypertensive individuals with and without hyperuricemia. Another study also reported a dose response relation between SU and carotid atherosclerotic plaques in men with and without cardiovascular risk factors. However, the same group did not find any association between the levels of SU and coronary artery calcification (CAC). The associations between SU, CAC, and IMT were reevaluated in an analysis of 5115 young adults aged 18 to 30 years and followed for 25 years. Using CAC and carotid IMT as markers of subclinical atherosclerosis, the investigators reported increased risks for CAC progression from years 15 to 25 with respect to baseline SU. For carotid IMT, SU at year 15 significantly predicted greater IMT at year 20, but this association remained significant in men only after adjusting for BMI. Greater increments in SU concentrations from years 0 to 15 were associated with higher risks of CAC progression and IMT. These findings supported a role for SU as a potential biomarker of subclinical atherosclerosis in young adults.
Urate-induced endothelial dysfunction secondary to reduced NO production precedes plaque formation and may play a more direct role ( Fig. 2 ). A recent review and meta-analysis of the use of xanthine oxidase inhibitors for the treatment of cardiovascular disease evaluated 3 outcome parameters (brachial artery flow–mediated dilation, forearm blood flow responses to acetylcholine infusion, and circulating markers of oxidative stress) and showed a significant improvement in each of them in patients with, or at risk of, cardiovascular disease. However, data on this aspect are still not conclusive.
Since the Framingham Heart Study in 1999 failed to identify a significant association between SU, cardiovascular disease, and cardiovascular death, several other population studies have presented contradictory evidence. An NHANES 2007–2008 analysis reported a 14% prevalence of myocardial infarction among individuals with gout, with an age-adjusted and sex-adjusted odds ratio (OR) of 2.68 (1.45 for men; 6.86 for women) compared with individuals who did not have gout. Prevalence of myocardial infarction in individuals with hyperuricemia was 5.7%, with an OR of 1.21 compared with normouricemic individuals. Prevalence of myocardial infarction was significantly higher in hyperuricemic or normouricemic individuals with gout (11.6% and 14.1%, respectively) compared with hyperuricemic individuals with no diagnosis of gout (5.7%). Other studies also support an increased risk of coronary heart disease (CHD) in women with gout. Recently, a population-based study of a Taiwanese cohort also reported gout as an independent risk factor for myocardial infarction and stated that this risk was greater in younger individuals without cardiovascular risk factors.
An initial meta-analysis on the subject reported a 13% increased risk (RR 1.13; 95% CI 1.07–1.20) of CHD among those in the top tertile of SU levels compared with those in the lowest tertile. A more recent meta-analysis of 26 studies with 402,997 adults reported a modest but significant increased risk of CHD incidence and mortality on hyperuricemic individuals, 1.09 (95% CI 1.03–1.16) and 1.16 (95% CI 1.05–1.19), respectively, even after adjusting for traditional risk factors. A nonsignificant increased CHD mortality RR of 1.12 was reported for each 1-mg/dL SU increase, with only the women subgroup analysis having a statistically significant but modest increase. So, although statistically significant, evidence so far has shown a small increase in risk of both incidence and mortality in CHD with hyperuricemia.
Peripheral arterial disease (PAD) is another manifestation of atherosclerosis, and scarce evidence regarding an association with SU has been published. A cross-sectional study among 3987 participants in NHANES 1999–2009 found that higher SU levels were significantly associated with PAD, independently from traditional cardiovascular risk factors. Another study analyzing data from the Multiple Risk Factor Intervention Trial showed an increased, although nonsignificant, odds of having PAD in association with hyperuricemia with an OR of 1.23 (95% CI 0.98–1.54). However, a history of gout was associated with an OR of 1.33 (95% CI 1.07–1.66), even after adjustment of underlying hyperuricemia. These findings are regarded as insufficient to assume a possible therapeutic approach.
Congestive heart failure
Increasing SU levels and hyperuricemia have been associated with increased incidence of congestive heart failure (CHF) and increased mortality in patients with established CHF. Data from NHANES 2007–2008 estimated increased point prevalences for CHF in hyperuricemic individuals compared with normouricemic individuals, with an OR of 2.52 (95% CI 1.58–4.04), and in individuals with gout compared with nongout, with an OR of 2.68 (95% CI 1.88–3.83). An analysis of the Framingham offspring cohort of 4989 adults, with no clinical CHF at baseline, showed that individuals with gout had a 2 to 3 times higher incidence of CHF and echocardiographic measures of systolic dysfunction. Median follow-up time was 15.9 years. Mortality was increased in participants with gout, with an adjusted hazard ratio (HR) of 1.58 (95% CI 1.40–1.78), compared with people without gout, and this effect was also observed in subgroup analysis comparing individuals with gout and CHF compared with those with heart failure but without gout.
However, evidence suggests that increased xanthine oxidase activity in damaged myocardial tissue results in the production of urate precursors and radical oxygen species, which are responsible for cardiac hypertrophy, myocardial fibrosis, left ventricular remodeling, and contractility impairment. This finding poses urate as a marker of increased xanthine oxidase activity rather than a cause. Support for this hypothesis was provided by an analysis on CHF outcomes in patients with and without chronic kidney disease (CKD), which concluded that hyperuricemia is associated with a poor outcome in CHF without CKD but not in patients with CHF and CKD. In the former case, hyperuricemia would be secondary to increased xanthine oxidase activity, rather than impaired excretion like in CKD. Although there are few therapeutic trials, data are suggestive of improvements in myocardial function and ejection fraction being secondary to xanthine oxidase inhibition rather than decreasing SU levels.
Cerebrovascular disease
New evidence has emerged regarding an association between SU and cerebrovascular disease. A study using brain magnetic resonance imaging (MRI) evaluated the aggregate volume of white matter hyperintense signals in a sample of 177 adults. High-normal SU (SU >5.75 mg/dL for men; >4.8 mg/dL for women) concentrations were associated with a significant increase in white matter hyperintense signals compared with participants with low to moderate SU levels. This association was still significant after adjusting for traditional risk factors. SU has also been postulated as a predictor of poor prognosis and recurrent events in stroke survivors.
Results from NHANES 2007–2008 showed an increased incidence of stroke in individuals with gout, with an OR of 2.02 (95% CI 0.98–4.19) and hyperuricemia 1.74 (95% CI 1.16–2.59), compared with the control population. Although the risk was increased in women, this difference was not significant. A systematic review and meta-analysis pooled a total of 16 studies including 238,449 adults and after adjusting for known risk factors, hyperuricemia was associated with a 47% (95% CI 1.19–1.76) increased risk of stroke and a 26% (95% CI 1.12–1.39) increased mortality. In this analysis, no significant difference by sex was observed. The intervention trials with ULT have had conflicting results on subclinical parameters, and no evidence supporting use of ULT in stroke patients is available.
An association between SU concentrations and cardiovascular disease is becoming firmly established for hypertension and is an evolving field, with still insufficient evidence for atherosclerosis, coronary artery disease, stroke, and CHF. The first attempts at a leap to causality are being made by the development of interventional clinical trials aimed at decreasing SU levels and affecting cardiovascular outcomes.
Renal Disease
Urate and renal disease: laboratory and animal models
Almost 90% of the filtered urate is reabsorbed at the proximal tubule by the urate anion exchanger URAT-1, located at the apical membrane of tubular cells. Urate regulation at the tubular level is a complex process that involves several other transporters, and conditions such as Lesch-Nyhan syndrome and tumor lysis syndrome, in which SU levels increase more than 10 mg/dL, cause renal damage through urate deposition in the tubuli. Deposition of crystals within the tubuli has also been mentioned as an initial phase of the translocation of urate crystals to the interstitium and medulla, a component of the crystal-related nephropathy. This mechanism, which leads to tubular atrophy and vascular degeneration, used to be considered as the explanation for renal damage in patients with gout. However, with the decrease in incidence and severity of crystal nephropathy, this diagnosis is now considered only for specific subgroups, which include lead intoxication or genetic defeats leading to increased urate production.
However, animal models have shown significant evidence of renal injury and disease in absence of crystal deposition. Systemic and glomerular hypertension with increased vascular resistance and reduced renal blood flow secondary to increased oxidative stress and endothelial dysfunction was observed in rats with oxonic acid–induced hyperuricemia. In 2 of these models, changes were reversed by using tempol (a superoxide scavenger) and l -arginine (a substrate for endothelial NO synthase). Activation of the RAS also contributes to the development of vascular disease of the afferent arteriolar system and glomerular hypertrophy. The development of arteriolopathy leads to glomerular hypoxia and ineffective autoregulation mechanisms, which further increases the damage to the glomerulus. These changes result from specific mechanisms that involve stimulation of nicotinamide adenine dinucleotide phosphate (NADPH) oxidases with mitochondrial dysfunction, production of reactive oxygen species, activation of the RAS, smooth muscle cell proliferation, and induction of proinflammatory cytokines. Recent data have also shown a direct effect of urate in tubular cells, promoting a phenotypic transition of renal tubular cells such as epithelial-to-mesenchymal transition by decreasing expression of E-cadherin synthesis. Epithelial-to-mesenchymal transition is considered one of the initial phenomena in renal fibrosis.
Hyperuricemia-induced renal damage has been shown to have a significant effect in animal models with preexisting renal disease. This effect has been proved in nephrectomy injury models, in which ULT was shown to improve blood pressure and renal function and decrease histologic changes. In a model of cyclosporine nephropathy, increasing urate worsened renal disease and ULT ameliorated renal damage. An animal model of diabetic mice also showed that reducing SU improved diabetic nephropathy by reducing tubulointerstitial injury, with no effect on glomerular damage.
CKD
An association between hyperuricemia, including gout, and CKD has been frequently described in the literature and population studies. Data from NHANES 2007–2008 described a prevalence of CKD stage 3 or more in 19.9% of individuals with gout, associated with an OR of 2.32 (95% CI 1.65–3.26) when compared with individuals without gout. Prevalence in a hyperuricemic population was also significantly increased compared with a normouricemic population (14.8% vs 3.3%, respectively), with an OR of 3.96 (95% CI 2.63–5.97). Increased prevalence was observed with increasing values for SU. Although the causality of these observations has been difficult to confirm, because of the increasing SU with declining renal function, evidence from experimental data explained a possible role of SU in the incidence and progression of CKD.
The largest epidemiologic study to date, which included 177,570 adults from the US Renal Data System for 25 years, reported an independent association between SU and risk for end-stage renal disease with an HR of 2.14 (1.65–2.77) when comparing the highest with the lowest quartile. Another large cohort study evaluating 21,547 adults of the Vienna Health Screening project reported an almost double (OR 1.74; 95% CI 1.45–2.09) increased risk of kidney disease in individuals with SU levels between 7.0 and 8.9 mg/dL and a triple risk in individuals with levels more than 9.0 mg/dL (OR 3.12; 95% CI 2.29–4.25). A pooled study from the Atherosclerosis Risks in Communities and the Cardiovascular Health Study cohorts and 2 analyses from the Okinawa General Health Maintenance Association Study cohort also support an association between SU and the development of end-stage renal disease.
In IgA nephropathy, increased SU levels have also been reported as an independent predictor for the development of CKD. In diabetic patients, increased SU levels have been described as an independent predictor of the development of diabetic nephropathy, microalbuminuria and macroalbuminuria, and declining renal function in patients with type 1 diabetes. Hyperuricemia has also been associated, after adjusting for possible confounders, with an increased risk of incident CKD (OR 2.10; 95% CI 1.16–3.76) among patients with type 2 diabetes with normal kidney function. However, data analyzing the association between progression of CKD and SU are still not conclusive. A recent study of middle-aged and elderly Taiwanese adults with stage 3 to 5 CKD concluded that increased urate levels increased the risk of renal disease only in stage 3 CKD but not in more advanced stages. SU has also been reported as an independent risk factor for progression of kidney disease by other studies, although no association has been reported. This information may point to urate as a stronger risk factor for incidence rather than progression of CKD.
Treatment with allopurinol in hyperuricemic individuals with normal renal function has shown a beneficial effect on estimated glomerular filtration rate (eGFR). Interventional trials on patients with CKD, although scarce and small, have also shown supporting results. A randomized study of allopurinol and placebo in 54 patients with stage 3 to 4 CKD showed a slowing in disease progression in the treatment arm compared with placebo. Another study that included 113 patients with eGFR <60 mL/min/1.73 m 2 randomized patients either to allopurinol 100 mg/d or placebo for 24 months. After 24 months, there was no significant change in eGFR in the allopurinol group, whereas a significant decrease in eGFR was noticed in the control group. Allopurinol treatment slowed renal disease progression, estimating an HR reduction of 0.53 (95% CI 0.28–0.99). A beneficial effect on cardiovascular end points was also observed. A study using a different approach randomized 50 patients with CKD 3 to 4 already on allopurinol for treatment of mild hyperuricemia, to either continuation or withdrawal of allopurinol. After allopurinol withdrawal, there was a significant acceleration in the decline of renal function as well as worsening hypertension. In a post hoc analysis of the RENAAAL (Reduction of Endpoints in NIDDM with the Angiotensin II Antagonist Losartan) trial, in which the intervention was treatment with the antihypertensive losartan, a reduction of 6% of renal events was observed for every 0.5-mg/dL reduction of SU. The impact of SU reduction over time on the renoprotective effect of losartan was estimated by adjusting for the residual SU in the analysis of renal events, and after observing a mild reduction on the effect of losartan (from 22% to 17%), the investigators concluded that one-fifth of the observed renoprotective effect of losartan was attributed to its uricosuric effect. In diabetic patients with diabetic nephropathy, a small randomized placebo-controlled trial of 40 patients showed a reduction of proteinuria in patients treated with allopurinol.
Acute kidney injury
Hyperuricemia and acute kidney injury (AKI), via crystal-dependent mechanisms, have usually been associated in the context of tumor lysis syndrome. However, based on the observations of experimental models on crystal-independent renal injury, a possible association of SU and AKI has been described. In a small trial evaluating the incidence of postoperative AKI in patients undergoing high-risk cardiovascular surgery, SU greater than 6 mg/dL was associated with a 4-fold increase for AKI (OR 3.98; 95% CI 1.10–14.33). Poor survival after coronary artery bypass grafting has also been associated with increasing SU levels. A more recent study of 190 patients who underwent cardiovascular surgery reported increasing incidences of AKI (defined as absolute increase in serum creatinine level ≥0.3 mg/dL from baseline within 48 hours after surgery) with increasing levels of SU. In the multivariate analysis, SU levels starting from equal to or more than 5.5 mg/dL were associated with an increased risk, ascending up to a 35-fold (OR 35.4; 95% CI 9.7–128.7) with SU equal to or more than 7 mg/dL. A double-blind, placebo-controlled, randomized interventional trial using preoperative rasburicase in hyperuricemic patients undergoing high-risk cardiovascular surgery showed no benefit on postoperative serum creatinine levels. However, a decrease in the urine neutrophil–associated lipocalcin (a predictive marker of AKI in cardiovascular surgery patients) was reported in the rasburicase-treated patients. Information on this subject is still scarce and inconclusive.
Urolithiasis
Urate nephrolithiasis represents 7% to 10% of all nephrolithiases in the United States. Most individuals suffering from urate kidney stones are neither hyperuricemic nor suffer from gout, because the usual abnormality observed in these individuals is consistently acidic urine (pH <5.5). However, a large population study did report an association between the diagnosis of gout and an increased risk of incident kidney stones (RR 2.12; 95% CI 1.22–3.68). Hyperuricosuria, decreased urinary pH, and low urinary volume are considered the 3 main factors involved in the development of urate nephrolithiasis. The role of hyperuricemia and gout as risk factors may still not be clear, especially because studies have shown that most patients with gout are urate underexcretors. A stronger association between urate nephrolithiasis with type 2 diabetes mellitus and obesity has been described and explained in the basis of predisposition to an acidic urinary environment.
Evidence for urate crystal–independent mechanisms of renal injury, coupled to epidemiologic data, show a role for urate in the development of CKD. However, its importance in the progression of the disease and the potential use of ULT in patients with CKD is still unclear. Further clinical trials in patients with CKD are needed, as well as further information on the possible role of SU as a risk factor for AKI.
Metabolic Disease
Urate pathways and fructose: laboratory and animal models
The increased renal reabsorption of SU at the proximal tubules secondary to hyperinsulinemia has been regarded as the main hypothesis for the association between hyperuricemia and the metabolic syndrome (MS). However, models incorporating fructose metabolism are uncovering the contributory role of urate in the MS. Fructose consumption, either in the forms of table sugar or high-fructose corn syrup (in beverage and food sweetening), has increased in the last 30 years, and epidemiologic data have shown similar increments in obesity and associations with certain components of the MS. Fructose is phosphorylated by the enzyme fructokinase, which by having no negative feedback mechanism works uninterruptedly, causing intracellular phosphate and adenosine triphosphate depletion and increased activity by the adenosine monophosphate deaminase, leading to increased levels of urate. An overlap of the increasing consumption of fructose and a corresponding trend of SU has been recognized. Fructose-induced hyperuricemia has been proved to result in the development of insulin resistance (IR), hypertension, and renal injury in several animal models. This process has also been reversed by the administration of xanthine oxidase inhibitors (allopurinol and febuxostat) and uricosuric drugs (benzodiarone), suggesting a dependency on SU concentrations and not xanthine oxidase activity. Recent data on a trial using oxonic acid on rats fed with physiologic concentrations of fructose showed that although hyperuricemia did not increase body weight, blood pressure, or triglyceride levels, it did cause structural renal damage and significant increase in plasma insulin levels. Increased plasma insulin level, through development of IR, is regarded as the potential central promoter of the MS.
Based on findings and observations from experimental data, 2 different mechanisms that could explain the induction of IR, and hence hyperinsulinemia, have been proposed ( Fig. 3 ). First, hyperuricemia reduces endothelial NO bioavailabilty. Because NO is necessary for glucose uptake in skeletal muscle, alterations in carbohydrate metabolism occur secondary to this deficiency. Hypertension, another result of reduced NO bioavailability and damage to the endothelium has also been pointed as a possible mediator of the MS. A second important mechanism results from the inflammatory and oxidative changes in adipose tissue secondary to exposure to increased concentrations of urate. Intracellular urate in adipocytes, probably after translocation by URAT-1 transporters, increases oxidative stress by an increase in the enzymatic activity of the reduced form of the NADPH oxidase, giving rise to reactive oxygen species, which lead to protein nitrosylation, lipid peroxidation, and further NO reduction. This process also induces macrophage infiltration, liberation of other inflammatory molecules such as monocyte chemotactic protein 1, and reduction of adiponectin. These inflammatory and oxidative changes in adipocytes have been shown to cause MS in obese mice. A study with obese hyperuricemic mice showed that after decreasing urate levels with allopurinol, the proinflammatory endocrine imbalance was improved, with reduction of macrophage infiltration in adipose tissue, increase in adiponectin levels and reduction in IR.
IR and diabetes
As already seen in animal models, human studies have also shown an inverse correlation between high urate levels and insulin sensitivity. A large population study of 53,477 Korean adults showed that SU levels were independently correlated with IR and that this risk, as for the MS, was maintained when patients were within the normal range. Although a causal association is still in debate, a recent 15-year follow-up study of 5012 US nondiabetic adults has supported the contribution of SU to IR. After using regression models, it showed that individuals with hyperuricemia (defined as >7.0 mg/dL) were more likely to develop IR and prediabetes (HR 1.36 [95% CI 1.23–1.51] and 1.25 [95% CI 1.04–1.52], respectively). Another study also supported a higher risk of developing hyperinsulinemia with increased baseline urate levels in nondiabetic patients. Although interventional studies are still missing, a clinical trial of ULT with benzbromarone in patients with CHF and decreasing SU showed an improvement in IR.
Type 2 diabetes mellitus, the final expression of IR, has also been associated with increasing SU concentrations in epidemiologic studies. A 15-year follow-up study reported an increased risk for the development of diabetes in individuals with hyperuricemia (HR 1.87; 95% CI 1.33–2.62). An increased risk has also been reported by different cohorts. Data from NHANES 2007–2008 showed that individuals with hyperuricemia presented an increased risk for diabetes (OR 1.63; 95% CI 1.13–2.34) and that this risk had a dose-dependent association with SU levels. Diabetes prevalence among individuals with gout was also increased compared with individuals without gout (25.7% and 7.8%, respectively), reflecting an increased risk for diabetes among individuals with gout. However, data on this relation are still not conclusive, with several studies indicating either no association between SU and diabetes, or even an inverse relationship between both. Although results are based on epidemiologic data, causality is still controversial; use of SU as a predictor of all-cause mortality in type 2 diabetic patients was recommended in a study carried out on a cohort of 535 diabetic adults. This association remained significant after adjusting for other covariables.
MS
The MS represents a cluster of physiologic and anthropometric abnormalities (IR, increased blood pressure, truncal obesity, hypertriglyceridemia, and low high-density lipoprotein cholesterol [HDLc]) and is regarded as a risk factor for the development of diabetes and cardiovascular diseases. An association between MS and hyperuricemia has been already been well described. An analysis of NHANES III data showed an increasing prevalence of the MS with increasing levels of SU, equal to or more than 10 mg/dL compared with individuals with levels less than 6.0 mg/dL (70.7% and 18.9%, respectively). A significant difference in prevalence between individuals with and without gout was also shown (62.8% and 25.4%, respectively). The increasing risk of MS in individuals with higher SU levels has also been reported in other studies, and the increased SU levels have also been observed to be significantly increased by the number of components of MS. An increased risk has even been described in individuals with high-normal SU levels in a Korean cohort.
Besides IR and hypertension, associations between SU and other individual components of the MS have also been described. In a study of 11,182 subjects older than 65 years old, triglyceride levels and waist circumference showed a positive correlation with urate levels, whereas HDLc showed a negative correlation. These observations had been previously reported on patients with high cardiovascular risk in a population-based study in Spain. A strong relationship between hyperuricemia, gout, and obesity has been well documented, and data from an analysis of NHANES 1988–1994 and 2007–2010 have shown a progressively greater prevalence of gout in higher BMI categories. However, direct causality is still not clear, and it may involve leptin, adiponectin, and inflammation on adipocytes (see Fig. 3 ). A study analyzing data from individuals with and without hyperuricemia in Taiwan identified obesity and hypertriglyceridemia as possible potentiating factors on SU for the development of gout.
Neurologic Disorders
Antioxidants effects of urate
Compared with other mammals, higher levels of urate are secondary to the evolutionary loss of urate oxidase. This mutation was seen as beneficial and part of adaptation by several investigators, who postulated the presence of urate as a stimulant with positive effects on cognition, alertness, and motivation, or an antiaging effect through its ability to prevent oxidative damage. Although the theories of the antioxidant capacity of urate were ignored because of the association of high urate levels and cardiovascular risk, evidence for its neuroprotective effects and association with neurodegenerative disorders have resurfaced.
Urate can act as a powerful scavenger for peroxynitrite, peroxyl and hydroxyl radicals and has been shown to reduce oxidative damage in DNA molecules. In studies using cellular models of neurodegeneration, reduced oxidative stress and cell death have been associated with urate, and 1 study using a mouse model of Parkinson disease showed suppressed oxidative stress and death of dopaminergic cells. In 1994, the first study reporting an association of SU and Parkinson disease in postmortem human tissue samples showed that urate was significantly reduced in the substantia nigra of patients with Parkinson disease, and its addition decreased oxidation of dopamine in the caudate nucleus and substantia nigra.
Parkinson disease and other neurodegenerative conditions
The largest prospective study evaluating the relationship between hyperuricemia and the risk of developing Parkinson disease analyzed data from 18,018 men from the Health Professional Follow-up Study. During the observation period, 84 individuals were diagnosed with Parkinson disease, and after adjusting for other variables, the rate ratio for the highest quartile of uricemia compared with the lowest was 0.43 (95% CI 0.18–1.02). Data from a more recent study, the Atherosclerosis Risk in Communities (ARIC) study, reported a similar OR (0.4 [95% CI 0.2–10]), when comparing extreme quartiles of plasma urate. Data regarding risk of Parkinson disease on individuals with gout have also been reported by 2 studies. An analysis of the General Practice Research Database, which includes more than 3 million adults in the United Kingdom, reported that individuals with a previous history of gout had a lower risk, (OR 0.69; 95% CI 0.48–0.99) of developing Parkinson disease. This association was seen only in men. Another study based on an 8-year median follow-up of a Canadian cohort reported an adjusted RR of 0.70 (95% CI 0.59–0.83) among those with gout. A recent review on this subject suggested, although still not at a clinical level, the feasibility of using SU as a risk, diagnostic, and prognostic marker for Parkinson disease.
Data supporting this association have also been observed in other neurodegenerative diseases. In multiple sclerosis, a study showed that lower urate levels correlated with a worse prognosis, which manifested as relapses. A study of Huntington disease showed that higher SU levels correlated with a slower disease progression and identified a trend of decreased worsening of motor function with increasing urate. Although evidence from interventional trials is lacking, a small trial with 11 patients with multiple sclerosis treated with inosine (aimed at increasing SU levels) showed clinical improvement in 3 of the patients, and no disease progression in the rest. Evidence of this association is lacking, and further studies are needed to determine the nature of these observations.
Comorbidities associated with calcium pyrophosphate dihydrate crystal deposition disease
Pseudogout is just part of the spectrum of calcium pyrophosphate dihydrate crystal deposition (CPPD) disease, which also includes pyrophosphate arthropathy, asymptomatic chondrocalcinosis, and unusual presentations such as pseudorheumatoid arthritis or crowned dens syndrome. Population studies using radiologic evidence of chondrocalcinosis have estimated prevalence ranging between 7% and 10%, usually in the population older than 60 years, and have proved a positive association with age. However, prevalence can vary depending on the method of identification. Studies examining synovial fluid of osteoarthritic joints at the time of joint replacement have reported a 25% to 43% prevalence of CPPD crystals. Data on CPPD, as well as on the associations with other comorbidities, are scarce. Most relevant evidence for these associations is presented in the following sections.
Osteoarthritis
An association between CPPD disease and osteoarthritis (OA) has been discussed and suggested for years; however, the precise nature of this relationship is still unclear, and the existence of common risk factors (eg, aging and joint injury), makes studying the relationship complex and challenging. CPPD and other calcium crystals, such as basic calcium phosphate crystals, have been shown to generate calcium oscillations in articular chondrocytes and a prolonged inflammatory response, which can contribute or amplify articular degeneration and joint damage. Increased transcription of the progressive ankylosis homolog gene (ANKH), the mutations of which have been described in familial forms of CPPD disease, has been reported in OA meniscal cells.
The European League Against Rheumatism (EULAR) recommendations on CPPD disease analyzed the association with OA, taking into account 4 cross-sectional and 5 case-control studies. It estimated that people with OA were almost 3 times more likely to have CPPD (OR 2.66; 95% CI 2.00–3.54). A study including 2 cohorts of people with knee OA and radiographic Kellgren scores of greater than or equal to 2 used MRI to explore the relation between radiographic chondrocalcinosis and OA progression. After 30 months of imaging and follow-up, cartilage loss, used as a proxy for progressive OA, showed no correlation with the presence of chondrocalcinosis. Evidence about this subject is still not conclusive, and although calcium crystals may be involved in the process of OA, more studies are needed.
Metabolic and Endocrine Disorders
A relationship between hemochromatosis and CPPD disease has been described. A study of 178 patients diagnosed with hereditary hemochromatosis and not yet treated with phlebotomy showed a 30% prevalence of chondrocalcinosis and a positive correlation between the number of joints involved with age, ferritin level, and PTH 44–68. An older case series of 54 patients with hemochromatosis reported a significant association (OR 6.81; 95% CI 2.02–22.95) with chondrocalcinosis. Although still under debate, the significance of this association may be relatively small. This observation was shown by a study that carried out systematic genetic testing of 128 patients with chondrocalcinosis and pseudogout, which reported a low prevalence of C282Y homozygotes and C282Y/H63D compound heterozygotes (1.6% and 3.1%, respectively).
The 2011 EULAR report on CPPD disease, which pooled data from 5 studies, described an association between CPPD disease and hyperparathyroidism, showing that patients with hyperparathyroidism were 3 times more likely to have CPPD than controls (OR 3.03; 95% CI 1.15–8.02). Triggering of pseudogout attacks by parathyroidectomy has also been reported by some studies. However, data on this association are still scarce.
A cross-sectional study of 72 patients with intestinal failure and in parenteral nutrition showed a significant association between hypomagnesemia and chondrocalcinosis. Compared with healthy controls, these patients with chronic hypomagnesemia presented a higher prevalence of chondrocalcinosis (16.6% vs 2.7% in controls), and prevalence of chondrocalcinosis was significantly higher (OR 13.5; 95% CI 2.76–127.3) in patients with lower serum magnesium levels. Reports on Gitelman syndrome and chondrocalcinosis support this association. CPPD arthropathy or pseudogout can be the onset of presentation of Gitelman syndrome, and this disease should be considered in the differential of younger patients presenting with CPPD disease.
Hypophosphatasia, gout, ochronosis, familial hypocalciuric hypercalcemia, X-linked hypophostatemic rickets, Wilson disease, and acromegaly are additional diseases that have been linked to CPPD disease. However, data on these associations are based only on case reports.
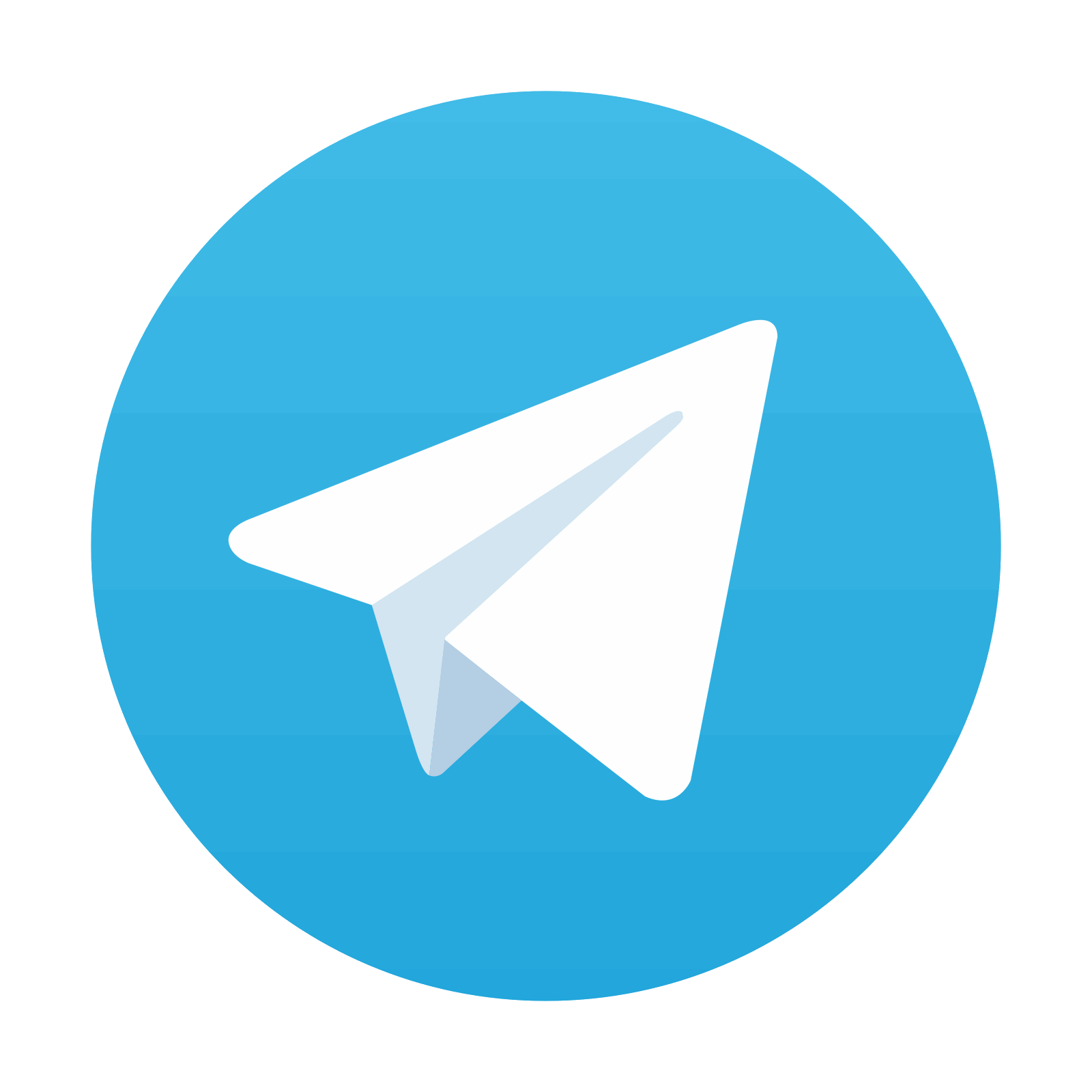
Stay updated, free articles. Join our Telegram channel

Full access? Get Clinical Tree
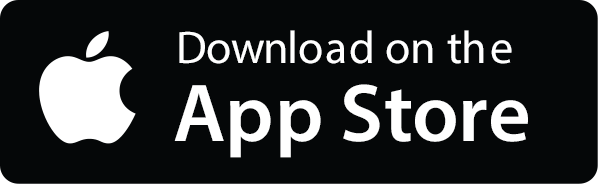
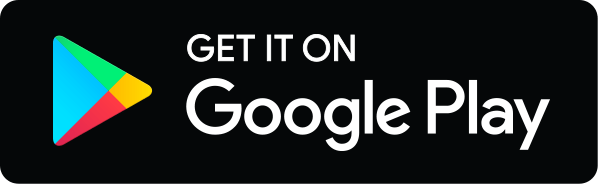