The repair of articular cartilage defects in patients’ knees presents a particular challenge to the orthopedic surgeon because cartilage lacks the ability to repair or regenerate itself. Various cartilage repair techniques have not produced a superior or uniform outcome, which has led to a new generation of cartilage repair based on tissue-engineering strategies and the use of biological scaffolds. Clinical advances have been made regarding the regeneration of articular cartilage, and continue to be made toward the achievement of a suitable treatment method for resurfacing osteochondral defects, through cartilage tissue engineering and the use of pluripotent cells seeded on bio-scaffolds.
Articular cartilage is a highly organized tissue with complex biomechanical properties and substantial durability. Hyaline cartilage is a highly specialized connective tissue, which permits smooth frictionless movement of joints and comprises chondrocytes embedded in an abundant extracellular matrix (ECM). The ECM is synthesized and secreted by the chondrocytes and consists predominantly of type II collagen, proteoglycans, and water, along with smaller amounts of other collagen types and noncollagenous proteins. The biomechanical properties of articular cartilage are largely dependent on the composition and the integrity of its ECM.
Articular cartilage, because of its avascular nature, has limited capability for self-repair and regeneration. Defects of articular cartilage are of 2 main categories, partial-thickness and full-thickness defects. Partial-thickness defects, on the one hand, are limited to the cartilage only and have no access to bone marrow–derived stem cells. Therefore, a chondral injury that does not violate the subchondral bone lacks an inherent ability to heal spontaneously, and may gradually expand with time. Full-thickness cartilage defects, on the other hand, have access to the bone marrow–derived stem cells of the subchondral bone and undergo some spontaneous healing, to a degree, through the formation of fibrocartilage. Fibrocartilage has inferior mechanical and biological properties compared with hyaline cartilage and can also gradually break down with time, resulting in permanent degradation of structure and function, ultimately leading to symptomatology and dysfunction. However, the natural history of an isolated articular cartilage lesion is not completely understood, nor is it clinically predictable which lesions will become symptomatic. Nevertheless, these defects lead to substantial patient morbidity and may progress to diffuse osteoarthritis if not treated early.
Articular cartilage injuries are common in the knee joint across all age groups, but are being 16–19,diagnosed and treated more frequently in young athletes. In studies of knee arthroscopies, articular cartilage lesions were observed in 66% of 993 knees (median patient age, 35 years), 63% of 31,516 knees (mean patient age, 43 years), and 60% of 25,124 knees (mean patient age, 39 years). Patients with symptomatic cartilage lesions often report pain, swelling, joint locking, stiffness, and clicking. Symptoms may cause significant functional impairment, often limiting one’s ability to play sports, work, and perform activities of daily living. Young patients with isolated focal chondral defects in the knee have considerable impairment of their quality of life, similar to that of patients requiring knee replacements for the treatment of end-stage arthritis and worse than that of patients with chronic anterior cruciate ligament–deficient knees. Adolescent patients may be particularly susceptible to osteochondral shearing injuries and osteochondral fractures. In a bovine model, adolescent cartilage failed at a lower shear stress in comparison with immature or adult cartilage, and also showed significantly reduced fracture toughness, requiring less energy to initiate and propagate a crack to a failure.
Because articular cartilage has limited ability to repair or regenerate itself, several cartilage repair techniques have been used to relieve symptoms and functional limitations. Current treatments for cartilage injury include, but are not limited to, debridement, chondroplasty, marrow stimulation techniques (ie, microfracture), bioabsorbable screw/pin fixation, osteochondral autografting/allografting, and cell-based therapies using cultured autologous chondrocytes.
Nonoperative therapy
Conservative nonoperative therapy can also be used to treat symptomatic knee lesions and includes physiotherapy, weight reduction, systemic analgesics, intra-articular injections, and orthotic interventions. Not all articular cartilage defects are symptomatic, so careful assessment of other potential causes of knee pain is also crucial. These modalities in combination help not only improve range of motion and potentially strengthen the limb but also decrease forces on the knee joint itself. Furthermore, a wide variety of analgesic and nonsteroidal anti-inflammatory drugs can be used, either alone or in combination, to aid in pain relief and improvement of function.
Nonoperative treatment methods focus on the management of chondral lesions, and a few predictably result in structural hyaline cartilage restoration. Surgical management should be considered if the patient’s symptoms are consistent with an underlying cartilage defect and adequate nonoperative management fails to provide acceptable pain relief or increase in function. Multiple studies indicate that treatment strategies for cartilage repair can be based primarily on the location and size of the defect, with age as a potential prognostigator. Patients must understand that rehabilitation is essential, and a successful outcome may not always result on returning to sports or stressful activities.
Nonreparative/nonrestorative procedures
There is a wide spectrum of various treatment approaches. Surgical procedures, which include debridement, chondral shaving, and joint lavage, can help minimize pain and improve mobility, but they do not restore the structure or function of the diseased cartilage. These procedures can be performed independently or in combination with other techniques to promote enhanced integration of the newly formed repair tissue with the surrounding native cartilage. However, limitations to these techniques include perimeter chondrocyte injury between healthy and damaged cartilage after the removal of the injured cartilage as well as the potential reduction in long-term beneficial outcomes.
Nonreparative/nonrestorative procedures
There is a wide spectrum of various treatment approaches. Surgical procedures, which include debridement, chondral shaving, and joint lavage, can help minimize pain and improve mobility, but they do not restore the structure or function of the diseased cartilage. These procedures can be performed independently or in combination with other techniques to promote enhanced integration of the newly formed repair tissue with the surrounding native cartilage. However, limitations to these techniques include perimeter chondrocyte injury between healthy and damaged cartilage after the removal of the injured cartilage as well as the potential reduction in long-term beneficial outcomes.
Repair/bone marrow–stimulating techniques
Bone marrow stimulation techniques have been commonly used for treating small symptomatic lesions of the articular cartilage in the knee. Reparative or bone marrow stimulation techniques aim at initiating bleeding from the subchondral bone by perforating the subchondral plate, which permits the migration of bone marrow stromal cells to the lesion along with “super” blood clot formation. The pluripotent cells are able to differentiate into fibrochondrocytes, which contribute to fibrocartilage repair of the lesion and to some degree of hyaline-like cartilage tissue. However, the overall concentration of the marrow cellular elements can be less than predictable and also declines with age. The formation of a stable and adhesive blood clot that maximally fills the chondral defect is important, and it has been correlated with the success of bone marrow stimulation procedures. In addition, creating a contained lesion and removing the calcified cartilage layer at the base of the lesion is critical to achieving a stable base for filling the defect with a clot and an optimal adhesion of the clot.
The reparative fibrocartilage that results from the successful reparative procedures consists of types I, II, and III collagen in varying amounts. The fibrocartilage does not resemble the surrounding hyaline cartilage and has less type II collagen. Although fibrocartilage has inferior mechanical properties and does not restore hyaline cartilage, the formation of this cartilage layer does cover the exposed underlying bone, subsequently helping to reduce pain and swelling. Examples of marrow stimulation techniques include abrasion arthroplasty, subchondral drilling, microfracture chondroplasty, and spongialization. Although various marrow stimulation techniques have been proved to produce excellent short-term clinical outcomes, the clinical durability of marrow-stimulated repair tissue has shown an objective and functional decline with long-term follow-up.
With osteochondritis dissecans or larger osteochondral defects and fractures, repair may be attempted using bioabsorbable screws, rods, or pins. Data in the pediatric population are limited to case reports or small case series, but show promising results. Fixation of lateral femoral condyle lesions with poly- d -dioxanone pins, polylactide rods, or poly- l -lactic acid screws showed good bony union on magnetic resonance imaging (MRI) and osteochondral reintegration on second-look arthroscopy. The largest case series to date consists of 8 patients treated with polyglycolic acid (PGA) rod fixation showed good functional results with more than 5 years of follow-up. On MRI there were no full-thickness defects, with 6 of the 8 patients showing no or a small area of cartilage thinning. Osteochondritis dissecans has also been treated with bioabsorbable fixation in several case series. One study of 13 patients explored arthroscopic fixation with polylactide screws and showed a significant improvement in function and return to sport in 12 of the 13 patients, with all patients rating their knee function as improved or much improved. In another study using polylactic rods in 9 skeletally mature adolescent and young adult patients, 8 of the 9 patients achieved radiographically united knees, with 7 patients having good to excellent results. Bioabsorbable pin resorption was studied in 59 patients with 175 polydioxanone pins using MRI. At 24 months only 20% of pins were visible on MRI and, although at 6 months 32% of patients had focal defects present, at later time points this dropped to only 4%. Bioabsorbable fixation shows promising results functionally and on imaging in specific clinical situations, but warrants further study in larger trials.
Restorative techniques
The treatment of the pathologic condition of articular cartilage of the knee has traditionally focused on realignment osteotomies and prosthetic replacement, particularly in older patients. For example, high tibial osteotomies and knee replacements aim to unload and replace the articular surfaces, respectively. More recent restorative techniques have focused on more biological approaches to resurfacing. At present, there has been a greater tendency to use biological approaches to treat cartilage lesions, especially in younger patients. Biological approaches include osteochondral grafting (mosaicplasty/osteochondral autograft transplantation and osteochondral allograft transplantation) and autologous chondrocyte transplantation/implantation (ACI), which have been clinically used to restore cartilage function and structure. Restorative strategies have been developed to promote the healing of injured cartilage through the transfer or formation of tissue that resembles native hyaline cartilage. Osteochondral autografting, mosaicplasty, and osteochondral allograft attempt to replace the cartilage defect with host or donor articular cartilage in a single stage, whereas ACI attempts to generate hyaline or hyaline-like cartilage in a 2-step surgical procedure (an arthroscopic procedure to harvest chondrocytes and an open procedure to reimplant cells).
Michelli and colleagues surveyed 32 adolescent patients who underwent ACI for articular cartilage defects. Twenty-eight of the patients reported an improvement in their overall condition score. Osteochondritis dissecans was treated using ACI and produced integrated repair tissue with successful clinical results in more than 90% of patients at 2 to 10 years of follow-up. This trial included 7 patients younger than 18 years, but does not specifically address the success rate in the adolescent patients.
Like many of the aforementioned procedures attempted thus far, these restorative strategies have been fraught with their own limitations, concerns, and problems while attempting to relieve pain, restore function, and delay or halt the progression of osteoarthritis. Although osteochondral autologous transfer and mosaicplasty have yielded results showing improved function and decreased pain, especially in full-thickness defects measuring 1 to 5 cm 2 , there still exists the issues of donor site morbidity, technically demanding issues (optimum orientation of the graft to restore the contour of the femoral condyle), and the ability of the cartilage plug to integrate with the adjacent normal cartilage. Similarly, ACI, used clinically to treat focal cartilage defects, has significant limitations. The technique requires a 2-staged surgical procedure, including harvesting and repair procedures, which can be challenging because it necessitates suturing the harvested periosteum to adjacent articular cartilage. Even if one were to put aside the technical issues, biology would still limit the procedure. Questions arise as to whether the cells would produce ECM in the proper ratio of cells to matrix, whether the cells would have characteristics such as alignment and metabolism similar to those of the surrounding normal cartilage, and whether the collagen fibers would form and orient properly within the different layers of cartilage. Furthermore, the complications of periosteal hypertrophy and/or delamination, inadequate cell supply, donor site morbidity, and arthrofibrosis have been documented.
Although many of the currently available surgical techniques have had documented improvement of joint function, relief of pain, and overall enhancement of their quality of life, no method has been viewed as overwhelmingly superior. Surgical procedures have had limited success in restoring native articular tissue both in structure and biomechanical properties. Despite the advancements and attempts at comparative assessments of these treatments, the lack of a consensus regarding an optimal treatment for the surgical repair of cartilage has contributed to the continued investigation of more advanced techniques using tissue engineering.
Tissue engineering/scaffolds
The lack of a consistent, superior, and reliable methodology to promote the repair of cartilage defects has resulted in a greater interest in tissue engineering. The strategy includes use of a viable cell source, a stable scaffold, and bioactive molecules, such as growth factors and morphogens. The approach involves a combination of these 3 principles to facilitate the repair of native articular cartilage ( Fig. 1 ). The cells selected also must have the capacity to proliferate and produce matrix. The cells cannot achieve this independently because there is a need for chemotactic agents to direct the cells along the appropriate pathway of growth and differentiation. The scaffold is essential to stabilize, anchor, and orient the cell construct.
There are certain characteristics that should be considered when specific scaffolds are designed. Safran and colleagues cited a comprehensive list stating that ideal scaffolds should be biocompatible, noncytotoxic, biodegradable, permeable, able to support and hold cells, mechanically stable, reproducible, readily available, and versatile for both full-thickness and partial-thickness lesions. When evaluating scaffolds, biocompatibility and a noncytotoxic nature ensures that there is no local inflammatory response or disruption of the surrounding tissues, which could prevent the cells from proliferation. In addition, while the scaffold needs to be stable and mechanically sound to support the cells, it also needs to serve as only a temporary load-sharing matrix that can eventually be fully replaced by the ECM of the cells. After remodeling, the scaffold should aid in the development of tissue-engineered cartilage with biphasic properties similar to those of the native cartilage, with approximately 80% fluid-fluid phase (80% water and <1% electrolytes) and 20% solid phase (10%–15% collagen type II and 5%–10% proteoglycans).
When expanded in a monolayer culture, chondrocytes start to lose their chondrogenic phenotype, but then regain their native phenotype when seeded in a 3-dimensional (3D) system using scaffolds. Regarding versatility, the regenerated tissue derived from the cell/scaffold construct should entirely fill the defect in the native cartilage, regardless of size, and integrate optimally with the surrounding native cartilage.
The scaffolds that have been described clinically thus far comprise a wide array of materials that include natural polymers, carbohydrates (hyaluronan, agarose, alginate, chitosan), and proteins (collagen, gelatin, fibrin), or synthetic/artificial polymers (polylactic acid [PLA], PGA, polyethylene oxide). Many of these matrices are currently being tested, with much of the early attention being directed toward the natural polymers and more recent studies focusing on hybrids and synthetic scaffolds.
The ideal cell-carrier substance should be the one that closely mimics the natural environment in the cartilage-specific ECMs. Carbohydrates, given the importance of glycosaminoglycans (GAGs) in stimulating chondrogenesis in vitro, have been used as scaffolds to enhance the chondrogenesis required in the repair of chondral defects. Hyaluronan (also called hyaluronic acid [HA] or hyaluronate) is an anionic nonsulfated GAG that is widely distributed throughout connective, epithelial, and neural tissues. HA is a promising scaffold material used to promote cartilage repair and is important in tissue development, with elevated levels during early developmental phases (cellular proliferation and migration) and subsequent decreased levels as cells differentiate into mature phenotypes. HA scaffolds have been shown to regulate, stabilize, and stimulate chondrogenesis. The HA scaffolds provide the structural support for cell contact and matrix deposition, but the 3D nonwoven HA scaffolds prevent dedifferentiation of chondrocytes as they bind to the HA through cell-surface receptors (CD44) and promote the expression of chondrocyte-specific markers, which induce signals that modulate cell proliferation, migration, and appropriate differentiation. Previous studies of HA-based polymers in the repair of osteochondral defects has been associated with encouraging outcomes because the resulting tissue consisted of hyaline-like cartilage that appeared to be well integrated with the adjacent cartilage. In addition, studies by Nettles and colleagues in which HA was injected into a rabbit osteochondral defect model showed that HA integrated well with the native tissue, promoted the migration of cells, aided in the production of cartilage-specific matrix and, most importantly, served as a valuable scaffolding material.
Specifically, Hyalograft C (Fidia Advanced Biopolymers, Abano Terme, Italy) has received clinical attention. This HA-based scaffold is an esterified derivative of HA (ACP or HYAFF 11), which has been used alone and in combination with autologous chondrocytes that have been expanded in culture. Studies have shown that this scaffold supports the in vitro growth of chondrocytes and promotes the maintenance and expression of their original phenotype, with a decrease in the amount of type I collagen as well as an increase in the amount of type II collagen and Sox9 production. Hyalograft C has been investigated clinically with encouraging results, and histologic analysis has shown hyaline-like cartilage in the lesion as early as 12 months after implantation. Marcacci and colleagues conducted a multicenter clinical study with the primary objective of investigating the outcomes of patients treated with Hyalograft C. Clinical results of 141 patients, with follow-up assessments ranging from 2 to 5 years, were reported and showed that 91.5% of patients improved according to the International Knee Documentation Committee subjective evaluation, 76% had no pain, 88% had no mobility problems assessed by the EuroQol-EQ5D measure, and 96% had the involved knee rated as normal or nearly normal by the treating surgeon. Seventy patients of this group underwent biopsy of the lesion; at less than 18 months from implantation 46% had hyaline or mixed (hyaline and fibrous or fibrocartilage) cartilage, and at more than 18 months 69% had hyaline or mixed cartilage at the repair site. Similarly, Gobbi and colleagues looked at 32 patients with lesions, mainly in the patellofemoral region, treated with Hyalograft C. MRI studies were then conducted at 24 months and revealed that 71% of patients had almost normal cartilage with positive correlation to clinical outcomes, and 6 second-look arthroscopies revealed the repaired surface to be nearly normal with biopsy samples characterized as hyaline-like in appearance. These and several other studies, which have actually compared Hyalograft C with procedures such as ACI and microfracture, have shown that the use of this scaffold proves to be a more viable treatment of chondral lesions and achieves repair tissue associated with histologic characteristics similar to normal articular cartilage.
More recently, other carbohydrates, including agarose, alginate, and chitosan, have gained attention. These substances have shown varying degrees of success for their potential to be incorporated either alone or in combination with other materials into scaffolds, and thus promote the production of components of a functional ECM, accumulate and attract cartilage markers, and maintain typical chondrocyte phenotype. Chitosan has been used extensively in a wide variety of scaffolds and different applications in articular cartilage tissue generation. Specifically, a scaffold comprising chitosan and β-glycerophosphate, known as BST-CarGel, was introduced for clinical application by Biosyntech (Quebec, Canada). BST-CarGel was developed to stabilize the blood clot in the cartilage lesion by dispersing a soluble and adhesive polymer scaffold containing chitosan throughout uncoagulated whole blood. Results in both animal studies and human trials have shown an increase in the volume and hyaline character of repair tissue, with increased GAG and collagen content especially when compared with microfracture controls. Regarding clinical investigation, 33 human subjects were treated with BST-CarGel from August 2003 to December 2004 on a case-by-case basis involving a spectrum of both traumatic and degenerative lesions. The outcomes were monitored by Western Ontario McMaster (WOMAC) Osteoarthritis Index questionnaires administered preoperatively and again postoperatively after 3, 6, and 12 months. Postoperatively at 12 months, WOMAC scores for pain, stiffness, and function improved significantly in patients treated with BST-CarGel, and although the results were recognized as anecdotal and short-term, the uniformity of the data suggested a clinical benefit following BST-CarGel treatment and has led to a current prospective, randomized, multicenter clinical trial in Canada comparing BST-CarGel with microfracture alone.
Protein-based scaffolds have also experienced variable successes that have led to the approval of multiple carrier matrices and procedures across Europe and the United States for use in patients. These include collagen I/III-based scaffolds (Carticel, Chondro-Gide, Autologous matrix-induced chondrogenesis) and fibrin glue (FG) (Tissucol; Baxter, Deerfield, IL, USA); and the continued clinical investigation of many others involving collagen I/III (matrix-induced autologous chondrocyte implantation [MACI], Neocart, CaReS, Vericart), fibrin (DeNovo NT and ET grafts, Gelrin C), and gelatin. Collagen has been shown to display many of the ideal properties required for a scaffold, including high seeding efficiency, good cell adhesion, elasticity to conform to various shaped defects, preservation of cell viability and morphology, durability to tear, and elaboration of chondrocytic markers that all lead to more effective repair of osteochondral defects as they are filled with hyaline-like tissue. In addition to the purely protein-based scaffolds are those scaffolds that involve a combination of natural polymers, such as Chondromimetic (Orthomimetics, Cambridge, UK), a novel biphasic biological scaffold comprising collagen and GAG.
Of the treatment methods using proteins as the scaffold, MACI (Genzyme Biosurgery, Cambridge, MA, USA) is one of the most widely used and clinically studied techniques, although it has not yet been released in the United States. This procedure is a modification of ACI and uses autogenous cells that are expanded in vitro, seeded between layers of a bilaminar collagen I/III scaffold (although other biomaterials have been used, ie, Hyalograft C), and implanted into the defect with the construct held in place by an FG. In multiple studies thus far, comparable results have been found between MACI and ACI procedures. Bartlett and colleagues performed a prospective, randomized comparison of ACI-C (a modification of original ACI that uses porcine-derived type I/type III collagen as a cover) and MACI for the treatment of symptomatic chondral defects of the knee in 91 patients, of whom 44 received ACI-C and 47 MACI grafts, and after 1 year concluded that the clinical, arthroscopic, and histologic outcomes were comparable for both groups. Bartlett and colleagues included adolescent patients as young as 15 years of age, but did not analyze these patients separately. Most recently, Zeifang and colleagues randomized 21 patients with symptomatic, isolated, full-thickness cartilage defects at the femoral condyle to MACI or the original periosteal flap technique (ACI), with evaluation based on postoperative changes in knee function, quality of life, and physical activity at 3, 6, 12, and 24 months, and MRI at 6, 12, and 24 months to evaluate the repair of cartilage using the Magnetic Resonance Observation of Cartilage Repair Tissue score. At both 1 and 2 years after the procedure there was an improvement of patients’ function, quality of life, activity level, and the Magnetic Resonance Observation of Cartilage Repair Tissue score following autologous chondrocyte implantation, but there was no significant difference between traditional ACI and MACI. Both of these examples illustrate that although MACI is technically appealing, further long-term studies are required before the technique becomes widely adopted.
Also in the protein family, commercial FG has long been used to secure tissue-engineered cartilage in clinical settings, and recently has been investigated and used clinically as a scaffolding material. Specifically, Denovo NT grafts (Zimmer, Warsaw, IN, USA; ISTO Technologies, St. Louis, MO, USA) showcase aseptically minced cartilage obtained from juvenile allograft donor joint, which creates a larger surface area for cartilage expansion, that is then is mixed intraoperatively with FG, which serves as a scaffold carrier allowing a chondroconductive milieu to be implanted into the lesion.
Synthetic or artificial biodegradable scaffolds are currently undergoing different phases of early clinical investigation. These include scaffolds made from PGA (BioSeed-C, CAIS scaffolds), PLA (BioSeed-C), Polylactic coglycolic acid (TruFit bone substitute plus, BioSeed-C), and polyethylene glycol (Gelrin C, ChonDux). This class of scaffolds is interesting because they possess some of the important characteristics needed to function as an ideal scaffold, such as a high mechanical strength, a predictable degradation rate, high reproducibility, maintenance of chondrocyte morphology, promotion of the formation of an ECM resembling normal cartilage, and easy manipulation.
One particular synthetic scaffold that has gained significant attention as a promising device for the treatment of osteochondral defects is the TruFit plug (Smith & Nephew, Andover, MA, USA), a synthetic resorbable biphasic implant. The device is made predominantly from PGA and poly- d , l -lactide-coglycolide fibers, which are preferentially aligned to provide good structure and are separated by appropriately sized pores, to allow ingrowth. In addition, calcium sulfate forms 10% of the structure in its base, as well as a trace amount of surfactant. The bilayer design of TruFit provides cartilage and bone phases yielding comparable mechanical properties for adjacent tissue. The implant is designed to fully resorb over time, potentially allowing for the complete filling of the defect with repair tissue to the same height as that of the surrounding articular surface. Preclinical studies performed on osteochondral defects involving the medial femoral condyles and the distal-medial portion of the patellar grooves in goat models led to qualitative evaluations that showed repair sites with a high percentage of hyaline cartilage, good bony restoration, and the good integration of new tissue with the native cartilage. These studies led to the clinical investigation of TruFit, which is approved in Europe for the treatment of acute osteochondral defects and by the US Food and Drug Administration only for backfill of osteochondral autograft sites. Clinical investigation with the use of these plugs to repair cartilage in knees has thus far led to some conflicting clinical outcomes. Most recently, Dhollander and colleagues reported on 20 patients who were consecutively treated for their cartilage lesions with the plug technique. These patients were then prospectively clinically evaluated at 6 and 12 months of follow-up and also examined by MRI. The investigators noted the short-term clinical and MRI outcomes to be modest. Of the 15 patients followed up during 1 year, 3 were considered failures (20%) because they showed persistent clinical symptoms or even more clinical symptoms after insertion of the plug. These patients underwent revision surgery, and the histologic assessment of biopsy specimens taken showed fibrous vascularized repair tissue with the presence of foreign-body giant cells.
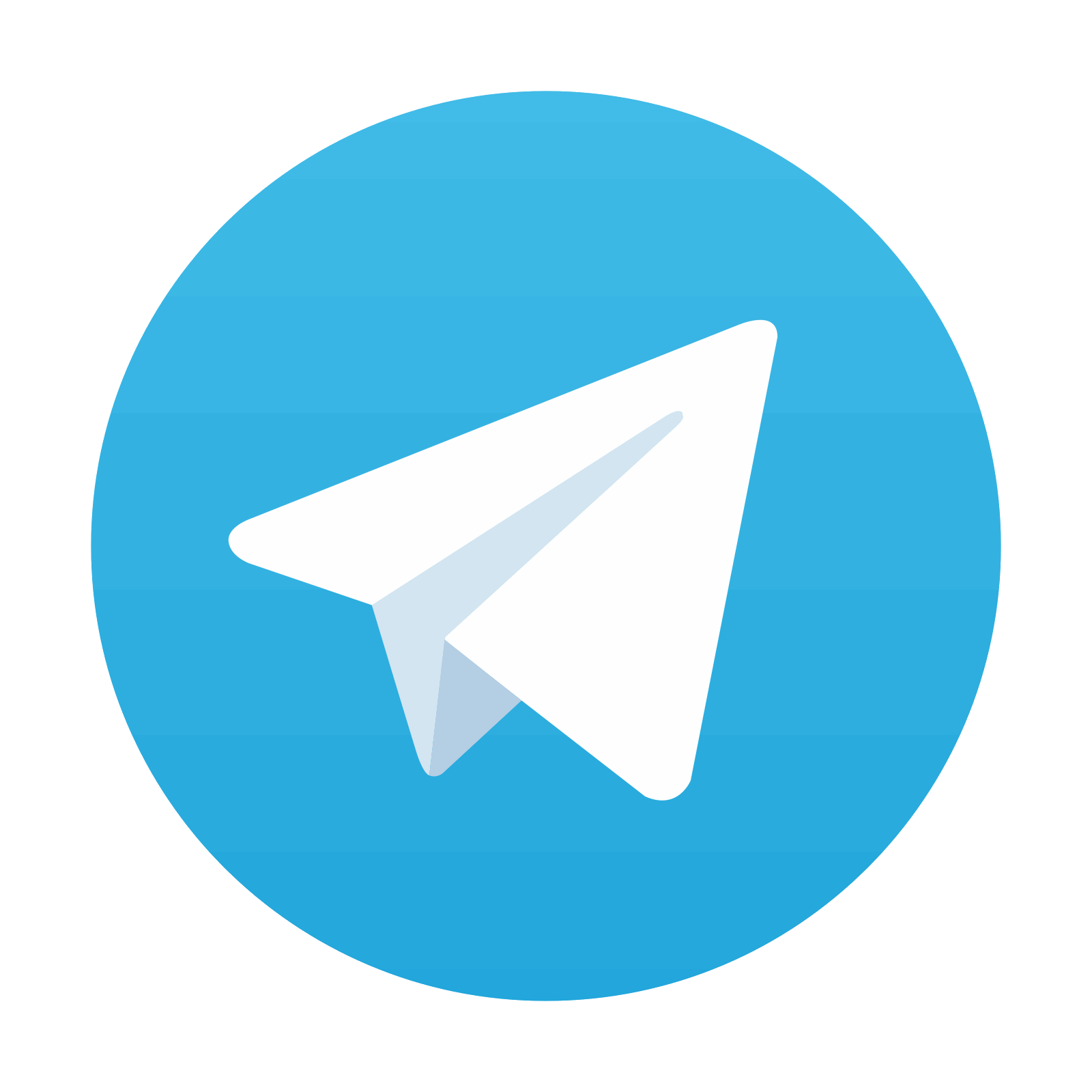
Stay updated, free articles. Join our Telegram channel

Full access? Get Clinical Tree
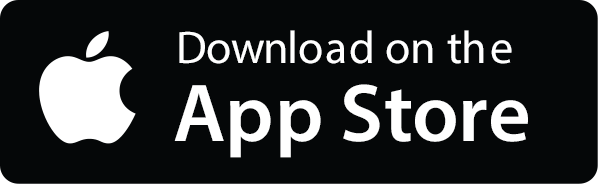
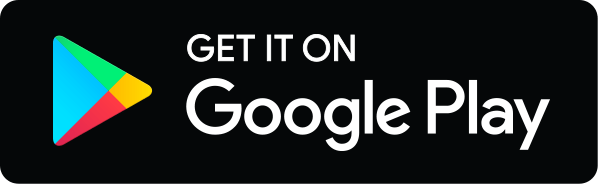