Direction relative to aircraft
Part 23
Part 25
Part 27 and 29
Small airplanes
Transport airplanes
Rotorcraft
Load factor
Load factor
Load factor
Forward
9.0
9.0
16.0
Sideward
1.5
4.0
8.0
Upward
3.0a
3.0
4.0
Downward
3.0b
6.0
20.0
Rearward
None
1.5
1.5
Occupant weight
215 lbc
170 lb
170 lb
Fitting factor
1.33
1.33
1.33
Accident investigations and research utilizing dynamic impact tests revealed that crash survival could be greatly improved if a means of quantitatively evaluating injury potential of the seating systems was developed and incorporated into the seat safety standards [4]. These new injury criteria, assessed during dynamic tests, would complement, rather than replace, the existing static strength and occupant protection requirements. In the early 1980s, a combined industry and government group known as the General Aviation Safety Panel (GASP) utilized the results of accident investigations, full-scale impact test data, military crashworthiness standards, and automotive injury criteria to formulate recommendations for dynamic test requirements and injury criteria for evaluation of general aviation seating systems. The GASP recommendations served as the basis for additional FAA regulations pertaining to general aviation. The injury criteria cited in the recommendations were also used to develop similar regulations covering rotorcraft and transport category aircraft [7].
Dynamic testing and occupant injury assessment have been required for seats in transport category airplanes certified since the adoption of 14 CFR 25.562 in 1988, and similar regulations in Parts 23, 27, and 29 [8]. The FAA later extended the safety benefit of these regulations to older airliner designs (referred to as derivatives) that are continuing to be built. This regulation required that all newly built aircraft intended for Part 121 (regularly scheduled airline) service delivered after October 2009 also meet the dynamic testing requirements [9]. The regulations require two basic tests (Fig. 28.1 and Table 28.2). Test 1 is a primarily vertical impact test that assesses the structural strength of the seat, and potential spinal injuries. Test 2 is primarily a horizontal test that assesses the structural strength of the seat, with an emphasis on the restraint system’s performance. Both tests define the impact in terms of a total velocity change, peak acceleration, and a maximum time to achieve that peak. These impact requirements reflect the forces that could be transmitted to the aircraft cabin during the most likely, survivable impact scenarios.
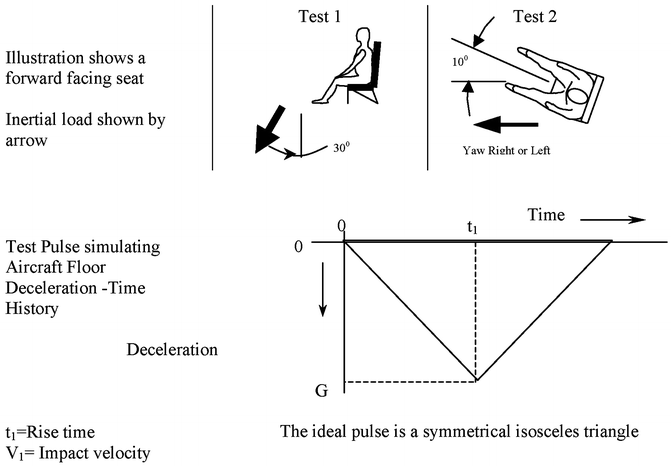
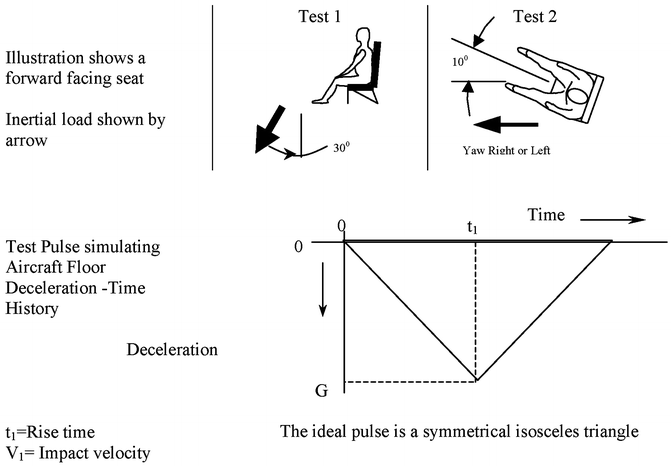
Fig. 28.1
Dynamic test requirements- seat orientation and test pulse shape
Table 28.2
Dynamic test requirements-summary
Dynamic test requirements | Part 23 | Part 25 | Part 27 | Part 29 |
---|---|---|---|---|
Test 1 | ||||
Velocity change (ft/s) | 31 | 35 | 30 | 30 |
Seat pitch (degree) | 60 | 60 | 60 | 60 |
Seat yaw (degree) | 0 | 0 | 0 | 0 |
Peak acceleration (G) | 19/15a | 14 | 30 | 30 |
Time to peak (s) | 0.05/0.06a | 0.08 | 0.031 | 0.031 |
Floor deformation (degree) | None | None | 10 pitch | 10 pitch |
10 roll | 10 roll | |||
Test 2 | ||||
Velocity change (ft/s) | 42 | 44 | 42 | 42 |
Seat pitch (degree) | 0 | 0 | 0 | 0 |
Seat yaw (degree) | 10 | 10 | 10 | 10 |
Peak acceleration (G) | 26/21a | 16 | 18.4 | 18.4 |
Time to peak (s) | 0.05/0.06a | 0.09 | 0.071 | 0.071 |
Floor deformation (degree) | 10 pitch | 10 pitch | 10 pitch | 10 pitch |
10 roll | 10 roll | 10 roll | 10 roll |
Small airplanes and rotorcraft have more severe test requirements than transport category aircraft. For the vertical test, this increase in severity reflects the additional load transmitted to the aircraft cabin due to the lack of crushable space between the bottom of the fuselage and the floor of the small aircraft. The horizontal test has higher severity because the lower momentum of these smaller and lighter aircraft results in a shorter stopping distance. For a given impact velocity, a shorter stopping distance produces a higher level of acceleration. Both of these test conditions have associated injury metrics (Table 28.3) that must be met before a test is considered a pass and the seat is certified for use in aircraft. These injury metrics include limits on lumbar spine and leg loads, limits on head acceleration, and limits on shoulder strap loads. The pass/fail requirements also include qualitative injury criteria that are based on observed occupant interaction rather than a specific measurement. These criteria specifically require that belts remain in place on the pelvis and shoulder. While not explicitly limited, these occupant injury criteria are primarily focused on protecting the occupant during forward and vertical impacts. This chapter will explain the origin and application of the criteria and how their intent has been applied to new seating configurations to maintain the level of safety established in the regulations.
Table 28.3
Dynamic test requirements-injury criteria pass/fail limits
Injury criteria | Part 23 | Part 25 | Part 27 | Part 29 |
---|---|---|---|---|
Max HIC | 1,000 | 1,000 | 1,000 | 1,000 |
Max lumbar load (lb) | 1,500 | 1,500 | 1,500 | 1,500 |
Max shoulder strap load (lb) | 1,750/2,000a | 1,750/2,000a | 1,750/2,000a | 1,750/2,000a |
Max femur load (lb) | N/A | 2,250 | N/A | N/A |
28.1 Injury Criteria Cited in the Aviation Regulations
28.1.1 Criteria Selection
The adoption of the dynamic seat requirements (Part xx.562) included the first occupant injury criteria in aviation regulations. The criteria were selected to protect occupants during expected impact scenarios (primarily longitudinal and vertical impact vectors relative to the aircraft), when seated in the most common types of seats (forward and aft facing). The criteria focused on controlling the most serious injuries that were likely to result from those combinations of seat types and impact scenarios. Some of the criteria selected were originally developed for automotive application but were found to also be applicable to the aviation environment for cases where the loading conditions were similar. However, some loading conditions, such as the high vertical loads that can be experienced in an aviation crash, required unique injury criteria.
28.1.2 Head
Injuries to the head during an aviation accident are possible if the head comes into contact with any of the various structures in the aircraft. These include locations on the seat, such as the seat back, arm rest, tray table, or any equipment mounted on the seat. The head could also contact nearby structures such as a partition, galley wall, instrument panel, glare shield, or flight control. Contacts with hard surfaces can result in skull fractures and multiple types of brain injuries. Brain injuries are also possible even without head contact if inertial forces are high enough [10]. While serious head trauma is an immediate threat to life, in an aviation accident, lesser head injuries that render the occupant unconscious can result in fatality since rapid egress is important.
The head injury criterion (HIC) is used to evaluate head injury risk and is calculated according to the following equation:
Where:
![$$ HIC=\left\{\left({t}_2-{t}_1\right){\left[\frac{1}{\left({t}_2-{t}_1\right)}\underset{t_1}{\overset{t_2}{{\displaystyle \int }}}\;a(t)dt\right]}^{2.5}\right\} \max $$](/wp-content/uploads/2016/09/A31137_3_En_28_Chapter_Equa.gif)
t1 is the initial integration time, t2 is the final integration time, and a(t) is the total acceleration vs. time curve for the head strike, and where (t) is in seconds, and (a) is in units of gravity (g).
Note:
The values of t1 and t2 are selected such that the HIC value is the maximum possible for the time period being evaluated.
At the time of its adoption, HIC was considered the best available head injury indicator, and is still the most widely accepted method. The HIC calculation, however, tends to overestimate the injury risk for impacts that include a long period of low G acceleration, as can happen during occupant flailing prior to head contact with a hard surface or during contact with soft surfaces such as air bags. To address this issue, the aviation regulations specify that HIC is calculated using head acceleration data gathered during contact with the airplane interior. In addition to this requirement, the rotorcraft regulations limited the HIC time interval to a maximum of 50 ms, which in the course of the rule’s development, had been suggested as another means of addressing HIC’s tendency to overestimate the injury risk [11].
A value of 1,000 is cited as a pass/fail limit for HIC in the aviation regulations. Applying a cumulative normal distribution function (Fig. 28.2) to the available injury data indicates that a HIC = 1,000 is a 23 % risk of an AIS-3 or greater (serious) head injury or a 47 % risk of an AIS-2 or greater (moderate) head injury. The Abbreviated Industry Scale (AIS) developed by the Association for the Advancement of Automotive Medicine provides a means of quantifying the severity (or threat to life) of a specific injury [12, 13]. The relationship between HIC and injury is based on impact cases that are of short duration (less than 12 milliseconds), so it is valid for any HIC calculation method that disregards low-G, long duration acceleration by limiting HIC interval duration or excluding pre-impact acceleration.
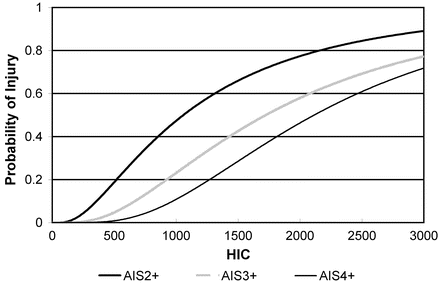
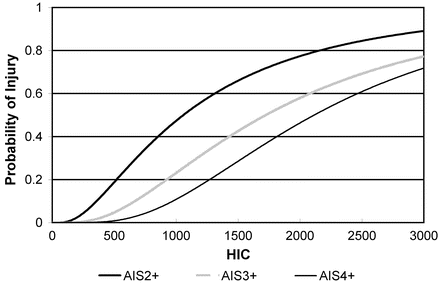
Fig. 28.2
Probability of AIS 2+, 3+, and 4+ head injury as a function of HIC
In U.S. automotive regulations [14], rather than limit the calculation period to only the duration of head contact, the pre- and post-contact periods of low-g acceleration were initially addressed by limiting the HIC time interval to 36 ms (a method referred to as HIC36), with a pass/fail value of 1,000. While this was an effective method for that impact environment, it was not adopted for aviation use because of the longer duration of some aviation impacts. The flail envelope of lap belt-restrained occupants can permit head contact with multiple interior features during a single impact event. Excluding time periods between contacts or calculating HIC separately for each period of contact would not comply with the original purpose of limiting HIC to the duration of contact, which was to exclude pre-contact, low-g acceleration. Calculating the HIC for each individual contact may also lead to allowing some designs to pass, where the method of continuously calculating HIC would show their non-compliance. Recent research indicates that some types of brain injuries are cumulative, not only during a specific impact event, but over a period of months or years [15, 16]. Beginning the HIC calculation at the time of initial contact and continuing through the end of the head impact event improves the validity of the injury risk prediction by excluding the pre-contact accelerations that can skew the prediction, while at the same time including all of the acceleration that could contribute to injury.
The HIC calculation method in U.S. auto regulations was later revised to limit the maximum time interval to 15 ms and reduce the pass/fail limit to 700 (referred to as HIC15). This change in calculation method was one of several new or revised injury criteria adopted to better assess the safety of autos incorporating airbags. The shorter time interval excludes more of the low-G acceleration produced during typical airbag contact. The lower limit was necessary for the HIC15 calculation to provide an equally stringent evaluation of long duration events. While HIC15 has not been adopted for aviation use at this time, it may be useful for evaluation of aviation airbag installations that produce occupant loading similar to automotive airbags.
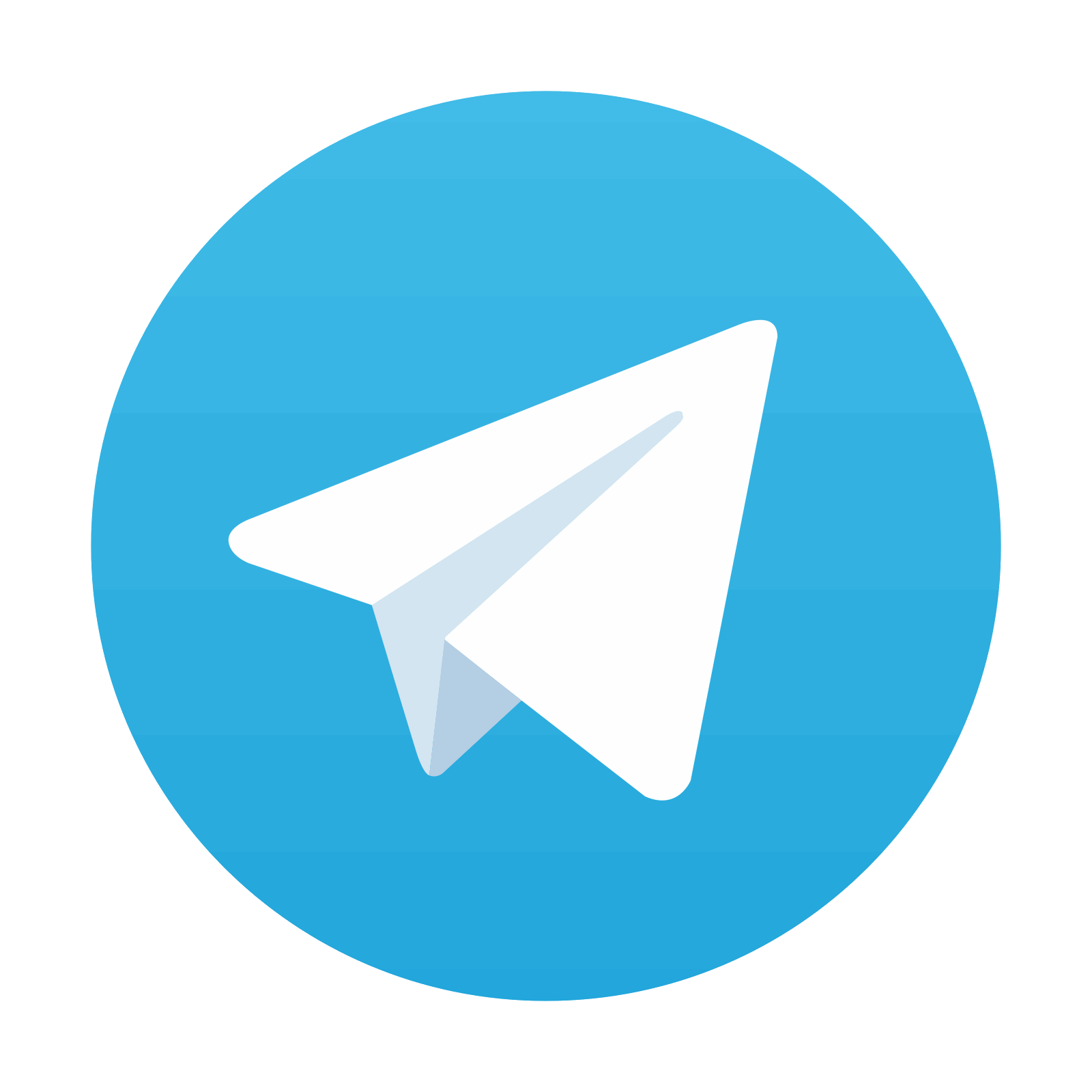
Stay updated, free articles. Join our Telegram channel

Full access? Get Clinical Tree
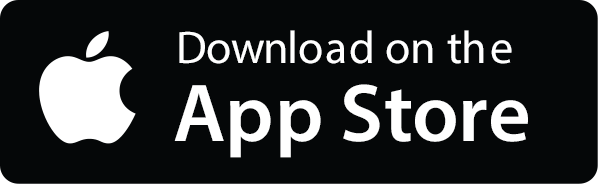
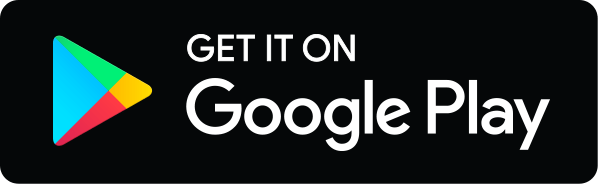