Radiographic Evaluation of Specific Areas of the Spine
Atlantooccipital Junction. The atlantooccipital interval remains the most difficult to assess for abnormalities, partly because of the difficulty in obtaining quality radiographs and partly because of the lack of discrete and reproducible landmarks. The distance between the occipital condyles and the facet joints of the atlas should be less than 5 mm; any distance of more than this suggests an atlantooccipital disruption.70,231 The foramen magnum and its relationship to the atlas also are useful in detecting injuries of the atlantooccipital region. The anterior cortical margin of the foramen magnum is termed the basion, whereas the posterior cortical margin of the foramen magnum is termed the opisthion. The distance between the basion and the tip of the dens should be less than 12 mm as measured on a lateral radiograph.44 The Powers ratio (Fig. 23-1) is used to assess the position of the skull base relative to the atlas and is another way of evaluating the atlantooccipital region. To determine this ratio, a line is drawn from the basion to the anterior cortex of the posterior arch of C1, and this distance is divided by the distance of a line drawn from the opisthion to the posterior cortex of the anterior arch of C1. The value should be between 0.7 and 1; a higher value indicates anterior subluxation of the atlantooccipital joint and a lower value indicates a posterior subluxation. The problem lies in the fact that the basion is not always visible on plain radiographs. The Wackenheim line, which is drawn along the posterior aspect of the clivus, probably is the most easily identified line to determine disruption of the atlantooccipital joint. If the line does not intersect the tip of the odontoid tangentially and if this line is displaced anteriorly or posteriorly, disruption or increased laxity about the atlantooccipital joint should be suspected.
FIGURE 23-1 The Powers ratio is determined by drawing a line from the basion (B) to the posterior arch of the atlas (C) and a second line from the opisthion (O) to the anterior arch of the atlas (A). The length of the line BC is divided by the length of the line OA, producing the Powers ratio. (From Lebwohl NH, Eismont FJ. Cervical spine injuries in children. In: Weinstein SL, ed. The Pediatric Spine: Principles and Practice. New York, NY: Raven, 1994, with permission.)
Atlantoaxial Joint. The atlanto–dens interval (ADI) and the space available for the spinal canal are two useful measurements for evaluation of the atlantoaxial joint (Fig. 23-2). The ADI in a child is considered normal up to 4.5 mm, partly because the unossified cartilage of the odontoid, which is not seen on plain films, gives an apparent increase in the interval. At the level of the atlantoaxial joint, the space taken up is broken into Steel’s rule of thirds: one-third is occupied by the odontoid, one-third by the spinal cord, and one-third is free space available for the cord. These intervals also are easily measured on flexion and extension views and are helpful in determining instability. In children, extension views give the appearance of subluxation of the anterior portion of the atlas over the unossified dens, but this represents a pseudosubluxation and not instability.52,66
FIGURE 23-2 The ADI and the space available for cord are used in determining atlantoaxial instability. The Wackenheim clivus-canal line is used to determine atlantooccipital injury, while the McRae and McGregor lines are used in the measurement of basilar impression. (modified from Copley LA, Dormans JP. Cervical spine disorders in infants and children. J Am Acad Orthop Surg. 1998;6:204–214.)
Upper Cervical Spine. Anterior displacement of one vertebral body on another may or may not indicate a true bony or ligamentous injury. Displacement of less than 3 mm at one level is a common anatomic variant in children at the levels of C2 to C3 and C3 to C4. This displacement is seen on flexion radiographs and reduces in extension. The posterior line of Swischuk and Rowe287 has been described to differentiate pathologic subluxation from normal anatomic variation; this line is drawn from the anterior cortex of the spinous process of C1 to the spinous process of C3 (Fig. 23-3). The anterior cortex of the spinous process of C2 should lie within 3 mm of this line; if the distance is more than this, a true subluxation should be suspected (Fig. 23-4). Widening of the spinous processes between C1 and C2 of more than 10 mm also is indicative of a ligamentous injury and should be evaluated by further imaging studies.3
FIGURE 23-3 The spinolaminar line (Swischuk line) is used to determine the presence of pseudosubluxation of C2 on C3. (From Copley LA, Dormans JP. Cervical spine disorders in infants and children. J Am Acad Orthop Surg. 1998;6:201–214, with permission.)
FIGURE 23-4 A: Pseudosubluxation of C2 on C3. In flexion, the posterior element of C2 should normally align itself with the posterior elements C1 and C3. The relationship of the body of C2 with the body of C3 gives the appearance of subluxation; however, the alignment of the posterior elements of C1 to C3 confirms pseudosubluxation. B: True subluxation.
Lower Cervical Spine. Lateral radiographs of the cervical spine should be evaluated for overall alignment. The overall alignment can be evaluated by the continuous lines formed by the line adjoining the spinous processes, the spinolaminar line, and the lines adjoining the posterior and anterior vertebral bodies (Fig. 23-5). These lines should all be smooth and continuous with no evidence of vertebral translation at any level. Loss of normal cervical lordosis may be normal in children, but there should be no associated translation at any level.304 The interspinous distance at each level should be evaluated and should be no more than 1.5 times the distance at adjacent levels; if this ratio is greater, an injury should be suspected. There are calculated norms for the interspinous distances in children, and any value greater than two standard deviations above normal is indicative of a ligamentous injury.168 The measurement of soft tissue spaces is important in evaluating any evidence of swelling or hemorrhage, which may be associated with an occult injury. The normal retropharyngeal soft tissue space should be less than 6 mm at C3 and less than 14 mm at C6. These spaces may be increased in children without an injury who are crying at the time of the radiograph, because the attachment of the pharynx to the hyoid bone results in its forward displacement with crying, producing an apparent increase in the width of these spaces. These radiographs must be taken with the patient quiet and repeated if there is any doubt.
FIGURE 23-5 Normal relationships in the lateral cervical spine: 1, spinous processes; 2, spinolaminar line; 3, posterior vertebral body line; 4, anterior vertebral body line. (From Copley LA, Dormans JP. Cervical spine disorders in infants and children. J Am Acad Orthop Surg. 1998;6: 204–214, with permission.)
Special Imaging Studies
Most cervical spine injuries in children are detected by plain radiographs.10 Most ligamentous injuries can be identified on flexion and extension views of the cervical spine in a cooperative and alert patient. The roles of computed tomography (CT) imaging and magnetic resonance imaging (MRI) continue to evolve in the evaluation of trauma patients.55
Plain radiographs remain the standard for initial evaluation of the pediatric cervical spine; CT imaging as an initial diagnostic study is associated with an increase in radiation with no demonstrable benefit over plain radiographs.2 However, when CT imaging is used in children, a few salient points should be kept in mind. First, the proportion of a child’s head to his or her body is greater than that of an adult, so care must be taken not to position the head in flexion to obtain the scan, which could potentiate any occult fracture not seen on plain films (Fig. 23-6). Second, the radiation doses for CT imaging are significantly higher than for plain radiographs, and CT protocols for children should be used to limit the amount of radiation. Although axial views are standard, coronal and sagittal formatted images and three-dimensional reconstruction views provide improved anatomic detail of the spine and can be obtained without any additional radiation to the patient.129,184 In patients with head injuries, the cervical spine can be included in the CT image of the head to reduce the number of plain films necessary to rule out an occult spinal injury.155
FIGURE 23-6 Anterior translation with patient on a spine board.
MRI has become increasingly useful in evaluating pediatric patients with suspected cervical spine injuries (Fig. 23-7), especially for ruling out ligamentous injuries in patients who cannot cooperate with flexion and extension views.93 The advantages of an early MRI are the ability to allow mobilization if no injury is present and the early detection of an unrecognized spinal fracture to allow proper treatment. MRI also is useful in evaluating patients with SCIWORA. MR angiography (MRA) has replaced standard arteriography for evaluation of the vertebral arteries in patients with upper cervical spine injuries who have suspected arterial injuries.224 MRI also remains the best imaging modality for evaluating injuries of the intervertebral disks and is especially useful to detect disk herniation in adolescent patients with facet joint injuries that may require operative reduction.
FIGURE 23-7 MRI depicts injury to the cervical cord and upper cervical spine.
Classification of Cervical Spine Injuries
There is currently no fracture classification for pediatric cervical spine injuries. Fractures are defined by the level of injury as well as whether there is an associated bony injury or ligamentous injury. The presence of ligamentous injuries is more common in younger children whereas subaxial cervical injuries are more common toward skeletal maturity, and fractures may then be classified using adult fracture classifications. Classifications for specific fractures are discussed later in the chapter.
PATHOANATOMY AND APPLIED ANATOMY RELATING TO CERVICAL SPINE INJURY
Understanding the normal growth and development of the cervical spine is essential when treating a child with a suspected cervical spine injury. This will allow the physician to differentiate normal physes or synchondroses from pathologic fractures or ligamentous disruptions and will alert the physician to any possible congenital anomalies that may be mistaken for a fracture.
Upper Cervical Spine
At birth, the atlas is composed of three ossification centers, one for the body and one for each of the neural arches (Fig. 23-8). In approximately 20% of individuals, the ossification center for the anterior arch is present at birth; in the remainder they appear by 1 year of age. Occasionally, the anterior arch is bifid, and the body may be formed from two centers, or it may fail to completely form. The posterior arches usually fuse by 3 years of age; however, occasionally the posterior synchondrosis between the two arches fails to fuse, resulting in a bifid arch. The neurocentral synchondroses that link the neural arches to the body close by 7 years of age. They are best seen on an open-mouth odontoid view and should not be mistaken for fractures.50 The canal of the atlas is large enough to allow for the rotation that is necessary at this joint as well as some forward translation.52 The vertebral arteries are about 2 cm from the midline and run in a groove on the superior surface of the atlas. This must be remembered during lateral dissection at the occipital–cervical junction. The ring of C1 reaches about normal adult size by 4 years of age.10
FIGURE 23-8 Diagram of C1 (atlas). The body (A) is not ossified at birth, and its ossification center appears during the first year of life. The body may fail to develop, and forward extension of neural arches (C) may take its place. Neural arches appear bilaterally about the seventh week (D), and the most anterior portion of the superior articulating surface usually is formed by the body. The synchondrosis of the spinous processes unites by the third year. Union rarely is preceded by the appearance of the secondary center within the synchondrosis. Neurocentral synchondrosis (F) fuses about the seventh year. The ligament surrounding the superior vertebral notch (K) may ossify, especially in later life. (From Bailey DK. Normal cervical spine in infants and children. Radiology. 1952;59: 713–714, with permission.)
The axis develops from at least four separate ossification centers: one for the dens, one for the body, and two for the neural arches (Fig. 23-9). Between the odontoid and the body of the axis is a synchondrosis or vestigial disk space that often is mistaken for a fracture line. This synchondrosis runs well below the level of the articular processes of the axis and usually fuses at 6 to 7 years of age, although it may persist as a sclerotic line until 11 years of age.52 The most common odontoid fracture pattern in adults and adolescents is transverse and at the level of the articular processes. The normal synchondrosis should not be confused with this fracture; the synchondrosis is more cup-shaped and below the level of the articular processes. After 7 years of age, the synchondrosis should not be present on an open-mouth odontoid view; a fracture should be considered if a lucent line is present after this age. The neural arches of C2 fuse at 3 to 6 years of age; these are seen as vertical lucent lines on the open-mouth odontoid view. Occasionally, the tip of the odontoid is V-shaped (dens bicornum), or a small separate summit ossification center may be present at the tip of the odontoid (ossiculum terminale). An os odontoideum is believed to result from a history of unrecognized trauma. The differentiation between an os odontoideum and the synchondrosis of the body is relatively easy because of their relationships to the level of the C1 to C2 facet (Fig. 23-10).
FIGURE 23-9 Diagram of C2 (axis). The body (A) in which one center (occasionally two) appears by the fifth fetal month. Neural arches (C) appear bilaterally by the seventh fetal month. Neural arches fuse (D) posteriorly by the second or third year. Bifid tip (E) of spinous process (occasionally a secondary center is present in each tip). Neurocentral synchondrosis (F) fuses at 3 to 6 years. The inferior epiphyseal ring (G) appears at puberty and fuses at about 25 years of age. The summit ossification center (H) for the odontoid appears at 3 to 6 years and fuses with the odontoid by 12 years. Odontoid (dens) (I). Two separate centers appear by the fifth fetal month and fuse with each other by the seventh fetal month. The synchondrosis between the odontoid and neural arch (I) fuses at 3 to 6 years. Synchondrosis between the odontoid and body (L) fuses at 3 to 6 years. Posterior surface of the body and odontoid (M). (From Bailey DK. Normal cervical spine in infants and children. Radiology. 1952;59:713–714, with permission.)
FIGURE 23-10 CT scan showing presence of an os odontoideum. Note the position of the os well above the C1 to C2 facets. The scan also shows the vestigial scar of the synchondrosis between the dens and the body below the C1 to C2 facet.
The arterial supply to the odontoid is derived from the vertebral and carotid arteries. The anterior and posterior ascending arteries arise from the vertebral artery at the level of C3 and ascend anterior and posterior to the odontoid, meeting superiorly to form an apical arcade. These arteries supply small penetrating branches to the body of the axis and the odontoid process. The internal carotid artery gives off cleft perforators that supply the superior portion of the odontoid. This arrangement of arteries and vessels is necessary for embryologic development and anatomic function of the odontoid. The synchondrosis prevents direct vascularization of the odontoid from C2, and vascularization from the blood supply of C1 is not possible because the synovial joint cavity surrounds the odontoid. The formation of an os odontoideum after cervical trauma may be related to this peculiar blood supply (Fig. 23-11).
FIGURE 23-11 Blood supply to odontoid: posterior and anterior ascending arteries and apical arcade. (From Schiff DC, Parke WW. The arterial supply of the odontoid process. J Bone Joint Surg Am 1973;55:1450–1464, with permission.258)
Lower Cervical Spine
The third through seventh cervical vertebrae share a similar ossification pattern: a single ossification center for the vertebral body and an ossification center for each neural arch (Fig. 23-12). The neural arch fuses posteriorly between the second and third years, and the neurocentral synchondroses between the neural arches and the vertebral body fuse by 3 to 6 years of age. These vertebrae normally are wedge-shaped until 7 to 8 years of age.16,170,239 The vertebral bodies, neural arches, and pedicles enlarge by periosteal appositional growth, similar to that seen in long bones. By 8 to 10 years of age, a child’s spine usually reaches near adult size and characteristics. There are five secondary ossification centers that can remain open until 25 years of age.169 These include one each for the spinous processes, transverse processes, and the ring apophyses about the vertebral endplates. These should not be confused with fractures.
FIGURE 23-12 Diagram of typical cervical vertebrae, C3 to C7. The body (A) appears by the fifth fetal month. The anterior (costal) portion of the transverse process (B) may develop from a separate center that appears by the sixth fetal month and joins the arch by the sixth year. Neural arches (C) appear by the seventh to ninth fetal week. The synchondrosis between spinous processes (D) usually unites by the second or third year. Secondary centers for bifid spine (E) appear at puberty and unite with spinous process at 25 years. Neurocentral synchondrosis (F) fuses at 3 to 6 years. Superior and inferior epiphyseal rings (G) appear at puberty and unite with the body at about 25 years. The seventh cervical vertebra differs slightly because of a long, powerful, nonbifid spinous process. (From Bailey DK. Normal cervical spine in infants and children. Radiology. 1952; 59:713–714, with permission.)
The superior and inferior endplates are firmly bound to the adjacent disk. The junction between the vertebral body and the endplate is similar to a physis of a long bone. The vertebral body is analogous to the metaphysis and the endplate to the physis, where longitudinal growth occurs. The junction between the vertebral body and the endplate has been shown to be weaker than the adjacent vertebral body or disk, which can result in a fracture at the endplate in the area of columnar and calcified cartilage of the growth zone, similar to a Salter–Harris type I fracture of a long bone.16 The inferior end plate may be more susceptible to this injury than the superior endplate because of the mechanical protection afforded by the developing uncinate processes.31
The facet joints of the cervical spine change in orientation with age. The angle of the C1 to C2 facet is 55 degrees in newborns and increases to 70 degrees at maturity. In the lower cervical spine, the angle of the facet joints is 30 degrees at birth and 60 to 70 degrees at maturity. This may explain why the pediatric cervical spine may be more susceptible to injury from the increased motion or translation allowed by the facet joint orientation.
Increased ligamentous laxity in young children allows a greater degree of spinal mobility than in adults. Flexion and extension of the spine at C2 to C3 are 50% greater in children between the ages of 3 and 8 years than in adults. The level of the greatest mobility in the cervical spine descends with increasing age. Between 3 and 8 years of age, the most mobile segment is C3 to C4; from 9 to 11 years, C4 to C5 is the most mobile segment, and from 12 to 15 years, C5 to C6 is the most mobile segment.4,231 This explains the tendency for craniocervical injuries in young children.
Several anomalies of the cervical spine may influence treatment recommendations. The atlas can fail to segment from the skull, a condition called occipitalization of the atlas, and can lead to narrowing of the foramen magnum, neurologic symptoms, and increased stresses to the atlantoaxial articulation, which often causes instability. Failure of fusion of the posterior arch of C1 is not uncommon and should be sought before any procedure that involves C1. Wedge-shaped vertebrae, bifid vertebrae, or a combination of these also can occur. Klippel–Feil syndrome consists of the classic triad of a short neck, low posterior hairline, and severe restriction of motion of the neck from fusion of the cervical vertebrae.134,160 Congenital fusion of the cervical spine may predispose a child to injury from trauma by concentrating stresses in the remaining mobile segments.
Hensinger et al.133 reported congenital anomalies of the odontoid, including aplasia (complete absence), hypoplasia (partial absence in which there is a stubby piece at the base of the odontoid located above the C1 articulation), and os odontoideum. Os odontoideum consists of a separate ossicle of the odontoid with no connection to the body of C2. The cause may be traumatic. These anomalies also may predispose a child to injury or instability.
TREATMENT OPTIONS FOR CERVICAL SPINE INJURIES
Initial Management of Patients with Suspected Cervical Spine Injury
The initial management of a child with a suspected cervical spine injury is paramount to avoiding further injury to the cervical spine and spinal cord. The initial management of any child suspected of having a cervical spine injury starts with immobilization in the field. Extraction from an automobile or transport to the hospital may cause damage to the spinal cord in a child with an unstable cervical spine injury if care is not taken to properly immobilize the neck. The immobilization device should allow access to the patient’s oropharynx and anterior neck if intubation or tracheostomy becomes necessary. The device should allow splintage of the head and neck to the thorax to minimize further movement.
The use of backboards in pediatric trauma patients deserves special attention because of the anatomic differences between children and adults. Compared to adults, children have a disproportionately larger head with respect to the body. This anatomic relationship causes a child’s cervical spine to be placed in flexion if immobilization is done on a standard backboard. Herzenberg et al.136 reported 10 children under the age of 7 years whose cervical spines had anterior angulation or translation on radiograph when they were placed on a standard backboard. The use of a backboard with a recess so that the head can be lowered into it to obtain a neutral position of the cervical spine is one way to avoid unnecessary flexion. Another is a split-mattress technique in which the body is supported by two mattresses and the head is supported by one mattress, allowing the cervical spine to assume a neutral position. Children younger than 8 years of age should be immobilized on a backboard using one of these techniques (Figs. 23-13 and 23-14).57,213
FIGURE 23-13 A: Adult immobilized on a standard backboard. B: Young child on a standard backboard. The relatively large head forces the neck into a kyphotic position. (From Herzenberg JE, Hensinger RN, Dedrick DK, et al. Emergency transport and positioning of young children who have an injury of the cervical spine: the standard backboard may be hazardous. J Bone Joint Surg Am. 1989;71:15–22, with permission.)
FIGURE 23-14 A: Young child on a modified backboard that has a cutout to the recess of the occiput, obtaining better supine cervical alignment. B: Young child on modified backboard that has a double-mattress pad to raise the chest, obtaining better supine cervical alignment. (From Herzenberg JE, Hensinger RN, Dedrick DK, et al. Emergency transport and positioning of young children who have an injury of the cervical spine: the standard backboard may be hazardous. J Bone Joint Surg Am. 1989;71:15–22, with permission.)
Cervical collars supplement backboards for immobilization in the trauma setting. While soft collars tend to be more comfortable and cause less soft tissue irritation, rigid collars are preferred for patients with acute injuries because they provide better immobilization. Even rigid collars may allow up to 17 degrees of flexion, 19 degrees of extension, 4 degrees of rotation, and 6 degrees of lateral motion.63,196 Supplemental sandbags and taping on either side of the head are recommended in all children and have been shown to limit the amount of spinal motion to 3 degrees in any plane.142
Further displacement of an unstable cervical injury may occur if resuscitation is required. The placement of pediatric patients on an appropriate board with the neck in a neutral position makes recognition of some fractures difficult because positional reduction may have occurred, especially with ligamentous injuries or endplate fractures. An apparently normal lateral radiograph in a patient with altered mental status or multiple injuries does not rule out a cervical spine injury. A study of four patients with unstable cervical spine injuries who had attempted resuscitation in the emergency department showed that axial traction actually increased the deformity.31 Any manipulation of the cervical spine, even during intubation, must be done with caution and with the assumption that the patient has an unstable cervical spine injury until proven otherwise.
The physical evaluation of any patient with a suspected cervical spine injury should begin with inspection. Head and neck trauma is associated with a high incidence of cervical spine injuries.4,16 Soft tissue abrasions or shoulder-harness marks on the neck from a seatbelt are clues to an underlying cervical spine injury (Fig. 23-15).95,106,140 Unconscious patients should be treated as if they have a cervical spine injury until further evaluation proves otherwise. The next step in the evaluation is palpation of the cervical spine for tenderness, muscle spasm, and overall alignment. The most prominent levels should be the spinous processes at C2, C3, and C7. Anterior palpation should focus on the presence of tenderness or swelling. The entire spine should be palpated and thoroughly examined because 20% of patients with cervical spine injuries have other spinal fractures.
FIGURE 23-15 Clinical photograph of a patient with a cervical spine injury resulting from impact with the shoulder harness of a seatbelt. Note location of skin contusions from the seatbelt.
A thorough neurologic examination should be done, which can be difficult in pediatric patients. Strength, sensation, reflexes, and proprioception should be documented. In patients who are uncooperative because of age or altered mental status, repeat examinations are important; however, the initial neurovascular examination should be documented even if it entails only gross movements of the extremities. The evaluation of rectal sphincter tone, bulbocavernosus reflex, and perianal sensation are important, especially in obtunded patients and patients with partial or complete neurologic injuries, regardless of age. Patients who are cooperative and awake can be asked to perform supervised flexion, extension, lateral rotation, and lateral tilt. Uncooperative or obtunded patients should not have any manipulation of the neck.
Nonoperative Treatment of Cervical Spine Injury
Immobilization of the cervical spine may continue after the emergency setting if there is an injury that requires treatment. Specific injuries and their treatment are described later in this chapter. Further immobilization of some cervical spine injuries requires a cervical collar. A rigid collar can be used for immobilization if it is an appropriately fitting device with more padding than a standard cervical collar placed in the emergency department. More unstable or significant injuries can be treated with a custom orthosis, a Minerva cast, or a halo device. An advantage of custom devices is the ability to use lightweight thermoplastic materials that can be molded better to each patient’s anatomy and can be extended to the thorax (Fig. 23-16). These devices must be properly applied for effective immobilization, and skin breakdown, especially over the chin region, needs to be carefully monitored. Minerva casts tend to provide more immobilization than thermoplastic devices, but their use is not as common and their application requires attention to detail.
FIGURE 23-16 Custom-made cervicothoracic brace used to treat a C2 fracture that reduced in extension.
A halo device can be used for the treatment of cervical spine injuries even in children as young as 1 year old.253 The halo can be used as either a ring alone to apply traction or with a vest for definitive immobilization of an unstable cervical spine injury. Prefabricated vests are available in sizes for infants, toddlers, and children, with measurements based on the circumference of the chest at the xiphoid process.
The fabrication of a halo for any patient needs to consider both the size of the ring and the size of the vest. Prefabricated rings and prefabricated vests are available for even the smallest of patients and are based on circumferential measurements at the crown and at the xiphoid process. If the size of the patient or the anatomy of the patient does not fit within these standard sizes, the fabrication of a custom halo may be necessary. Mubarak et al.203 recommended the following steps in the fabrication of a custom halo for a child: (a) the size and configuration of the head are obtained with the use of the flexible lead wire placed around the head, (b) the halo ring is fabricated by constructing a ring 2 cm larger in diameter than the wire model, (c) a plaster mold of the trunk is obtained for the manufacture of a custom bivalved polypropylene vest, and (d) linear measurements are made to ensure appropriate length of the superstructure.
The placement of pins into an immature skull deserves special attention because of the dangers of inadvertent skull penetration with a pin. CT imaging before halo application aids in determining bone structure and skull thickness. It also aids in determining whether or not cranial suture interdigitation is complete and if the fontanels are closed. The thickness of the skull varies greatly up to 6 years of age and is not similar to that of adults until the age of 16 years.175 Garfin et al.97 evaluated the pediatric cranium by CT and determined that the skull is thickest anterolaterally and posterolaterally, making these the optimal sites for pin placement.
The number of pins used for placement of a ring and the insertion torques used in younger children also deserve special mention. The placement of pins at the torque pressures used in adults will lead to penetration during insertion.175 Pins should be inserted at torques of 2- to 4-in pounds; however, the variability and reliability of pressures found with various torque wrenches during cadaver testing are great, and each pin must be inserted cautiously.61 The use of 8 to 12 pins inserted at lower torque pressures aids in obtaining a stable ring with less chance of inadvertent penetration (Fig. 23-17). The insertion of each pin perpendicular to the skull also improves the pin–bone interface and the overall strength of the construct.62 We have had success using halo vests even in children younger than 2 years of age by using multiple pins inserted to finger-tightness rather than relying on torque wrenches.
FIGURE 23-17 “Safe Zone” for halo pin insertion. (Adapted from Manson NA, An HS. Halo placement in the pediatric and adult patient. In: Vaccaro AR, Barton EM: Operative Techniques in Spine Surgery. Philadelphia, PA: Saunders (2008), p. 13.185)
Technique of Halo Application
A halo can be applied in older children and adolescents with a local anesthetic; however, in most younger children a general anesthetic should be used. The patient is positioned on the operating table in a position that prevents unwanted flexion of the neck and maintains the proper relationship of the head and neck with the trunk. The area of skin in the region of pin insertion is cleaned with antiseptic solution and appropriate areas are shaved as needed for pin placement posteriorly. The ring is placed while an assistant holds the patient’s head; it should be placed just below the greatest circumference of the skull, which corresponds to just above the eyebrows anteriorly and 1 cm above the tips of the earlobes laterally. We recommend injection of local anesthetic into the skin and periosteum through the ring holes in which the pins will be placed. The pins are placed with sterile technique.
To optimize pin placement, a few points should be kept in mind. The thickest area of the skull is anterolaterally and posterolaterally, and pins inserted at right angles to the bone have greater force distribution and strength.62,97 Anterior pins should be placed to avoid the anterior position of the supraorbital and supratrochlear nerves (Fig. 23-18). Placement of the anterior pins too far laterally will lead to penetration of the temporalis muscle, which can lead to pain with mastication and talking, as well as early pin loosening. The optimal position for the anterior pins is in the anterolateral skull, just above the lateral two-thirds of the orbit and just below the greatest circumference of the skull. The posterior pins are best placed posterolaterally directly diagonal from the anterior pins. We also recommend placing the pins to finger-tightness originally and tightening two directly opposing pins simultaneously. During placement of the pins, meticulous attention should be paid to the position of the ring to have a circumferential fit on the patient’s skull and to avoid any pressure of the ring on the scalp, especially posteriorly.
FIGURE 23-18 Child immobilized in a halo for C1 to C2 rotary subluxation. Note the position of the anterior pins, as well as the placement of the posterior pins at 180 degrees opposite the anterior pins.
The number of pins used and the torque pressures applied vary according to the age of the patient. In infants and younger children, we recommend the placement of multiple pins (8 to 12) tightened to finger-tightness or 2- to 4-in pounds to avoid unwanted skull penetration. In older children, six to eight pins are used and tightened to 4-in pounds. In adolescents, four to eight pins can be tightened with a standard torque wrench to 6- to 8-in pounds. Once the pins are tightened, they must be fastened to the ring by the appropriate lock nuts or set screws. The halo vest and superstructure are then applied, with care to maintain the position of the head and neck. Appropriate positioning of the head and neck can be done by adjusting the superstructure (Fig. 23-19).
FIGURE 23-19 (Left) Custom halo vest and superstructure. (Right) In the multiple-pin, low-torque technique, 10 pins are used for an infant halo ring attachment. Usually, four pins are placed anteriorly, avoiding the temporal region, and the remaining six pins are placed in the occipital area. (From Mubarak SJ, Camp JF, Fuletich W, et al. Halo application in the infant. J Pediatr Orthop. 1989;9:612–613, with permission.)
Daily pin care should consist of hydrogen peroxide/saline cleaning at the pin–skin interface. Retightening of pins at 48 hours should be avoided in infants and children to prevent skull penetration; however, in adolescents, the pins can be retightened at 48 hours with a standard torque wrench. Local erythema or drainage may occur about the pins and can be managed with oral antibiotics and continued pin site care. If significant loosening occurs or the infection is more serious, the pin or pins should be removed. Occasionally, a dural puncture occurs during pin insertion or during the course of treatment. This necessitates pin removal and prophylactic antibiotics until the tear heals, usually at 4 to 5 days.
Outcomes
The complication rate related to the use of a halo in one series of patients was 68%; however, all patients were able to wear the halo until fracture healing occurred or arthrodesis was achieved.56 The most common complications in this series were superficial pin track infection and pin loosening. Other complications that occur less frequently include dural penetration, supraorbital nerve injury, unsightly pin scars, and deep infection.23,71 Prefabricated halo vests are used in adults and are easily fitted to older adolescents. Because of the age and size ranges of children, however, a custom vest or even a cast vest may be needed. Improper fitting of a vest may allow unwanted movement of the neck despite the halo, and any size mismatch requires a custom vest or cast vest (see Fig. 23-19).
Nonoperative Treatment of Spinal Cord Injury
If an acute spinal cord injury is determined by examination, the administration of methylprednisolone within the first 8 hours after injury has been shown to improve the chances of neurologic recovery.33–36 Methylprednisolone in the treatment of acute spinal cord injuries has been shown to improve motor and sensory recovery when evaluated 6 weeks and 6 months after injury35; however, this positive effect on neurologic recovery is limited to those treated within the first 8 hours of injury. The initial loading dose of methylprednisolone is 30 mg/kg body weight. If the loading dose is given within 3 hours after injury, then a maintenance infusion of 5.4 mg/kg is given for 24 hours after injury. If the loading dose is given between 3 and 8 hours after injury, then a maintenance infusion of 5.4 mg/kg is given for 48 hours after injury. Methylprednisolone decreases edema, has an anti-inflammatory effect, and protects the cell membranes from scavenging oxygen-free radicals.33–36
Once spinal cord injury is diagnosed, routine care includes prophylaxis for stress ulcers, routine skin care to prevent pressure sores, and initial Foley catheterization followed by intermittent catheterization and a bowel training program.
Outcomes
In several series,33–36 there was a slight increase in the incidence of wound infections but no significant increase in gastrointestinal bleeding. All of these studies involved patients 13 years or older, so no documentation of the efficacy in young children exists. A combination of methylprednisolone and GM1-ganglioside (GM1) is being studied for its possible beneficial effect on an injured spinal cord.99–102 GM1 is a complex acid-like lipid found at high levels in the cell membrane of the central nervous system that is thought to have a neuroprotective and neurofunctional restorative potential. Early studies have shown that patients given both drugs had improved recovery over those who had received just methylprednisolone. Spinal cord injury remains the most devastating complication after cervical spine fractures. The outcome after a spinal cord injury has been shown to be better in children than in adults and varying and unpredictable improvement in function may occur.225 The role of steroids in minimizing the inflammatory cascade and limiting neuronal injury has been well received after the results of the National Acute Spinal Cord Injury Studies (NASCIS) were published.33–36 Recently, the results of the studies have been called into question, and the evidence remains confusing to the physician taking care of patients with spinal cord injuries. There currently are no randomized clinical trials that have been performed in children with regard to pharmacologic measures to be taken after a spinal cord injury.226 With that being said, many clinicians continue administer steroids to children in the acute setting of a spinal cord injury given the potentially devastating effects of such an injury. The original recommendations to be followed were: methylprednisolone sodium succinate in a dose regimen of bolus 30 mg/kg over 15 minutes, with maintenance infusion of 5.4 mg/kg per hour infused for 23 hours. No clear evidence exists for the administration of steroids after spinal cord injury; however, a recent review on the subject reveals that many physicians continue to practice this in the face of an injury because of the perceived risks of litigation.113 The potential side effects of steroids, namely pulmonary complications and wound complications, should be weighed against both the paucity of evidence regarding their therapeutic value and the institutional protocols that may be in place for spinal cord injury patients.
With incomplete lesions, children have a better chance than adults for useful recovery. Hadley et al.115 noted that 89% of pediatric patients with incomplete spinal cord lesions improved, whereas only 20% of patients with complete injuries had evidence of significant recovery. Laminectomy has not been shown to be beneficial and can actually be harmful270,320 because it increases instability in the cervical spine; for example, it can cause a swan neck deformity or progressive kyphotic deformity.188,274 The risk of spinal deformity after spinal cord injury has been investigated by several researchers.21,25,47,85,158,188 Mayfield et al.188 found that patients who had a spinal cord injury before their growth spurt all developed spinal deformities, 80% of which were progressive. Ninety-three percent developed scoliosis, 57% kyphosis, and 18% lordosis. Sixty-one percent of these patients required spinal arthrodesis for stabilization of their curves. Orthotic management usually is unsuccessful, but in some patients it delays the age at which arthrodesis is necessary. Lower extremity deformities also may occur, such as subluxations and dislocations about the hip. Pelvic obliquity can be a significant problem and may result in pressure sores and difficulty in seating in a wheelchair.
Nonoperative Treatment of Neonatal Injury
Treatment of neonatal cervical spine injuries is nonoperative and should consist of careful realignment and positioning of the child on a bed with neck support or a custom cervical thoracic orthosis. Healing of bony injuries usually is rapid and complete.281
Operative Treatment of Cervical Spine Injury
Indications and contraindications are discussed with specific techniques later in the chapter.
Surgical Procedure(s)
Specific procedures are discussed later the chapter.
Preoperative Planning. The patient with an unstable cervical spine injury must be intubated and properly positioned to avoid further injury.
Stabilization. The injured cervical spine should be immobilized during transport. As discussed early in this chapter, in patients younger than 8 years of age the use of a backboard with and occipital recess or having the trunk elevated approximately 2.5 cm is recommended. This will allow the cervical spine to remain in neutral alignment due to the relative large head size compared with the trunk size in these younger patients. Soft cervical collars provide no significant stability to the cervical spine. Properly fitting cervical collars offer better support. The addition of sandbags and tape immobilization offers even more support.
Airway Management. In a patient with an unstable cervical spine, manipulation during intubation may injure the spinal cord. Axial traction, in particular, has been shown to result in increased distraction during intubation compared with either no immobilization or manual stabilization and is not recommended.172 Manual in-line stabilization (MILS) is the most widely accepted technique for immobilization during intubation. This technique consists of grasping the mastoid processes with the fingertips with no traction being applied and then cupping the occiput in the palm of the hands.104,183 Studies have confirmed the clinical safety of orotracheal intubation by direct laryngoscopy with MILS in patients with cervical spine injury.256,267
Several methods for intubation have been described for the patient with an unstable cervical spine. Awake intubation that is sometimes performed in adult patients is not feasible in the pediatric patient. Direct laryngoscopy with MILS is the method most often used. Fiberoptic intubation with MILS is a popular alternative. This causes minimal cervical movement and facilitates improved visualization of the vocal cords during intubation. However the time to intubation with the fiberoptic technique is twice as long compared with direct laryngoscopy. The GlideScope videolaryngoscopy (Verathon, Bothell, WA) provides an indirect view of the glottis on a screen and has the potential for reduced motion. Nasotracheal intubation can be performed fiberoptically or without direct visualization. This technique is contraindicated in patient with basilar skull fractures or craniofacial trauma, which often is the case in pediatric cervical spine trauma.
Spinal Cord Monitoring. Spinal cord monitoring is usually used during surgical stabilization of the unstable cervical spine. Motor potential and SSEP are used for monitoring (Table 23-2).
TABLE 23-2 Cervical Spine Injury
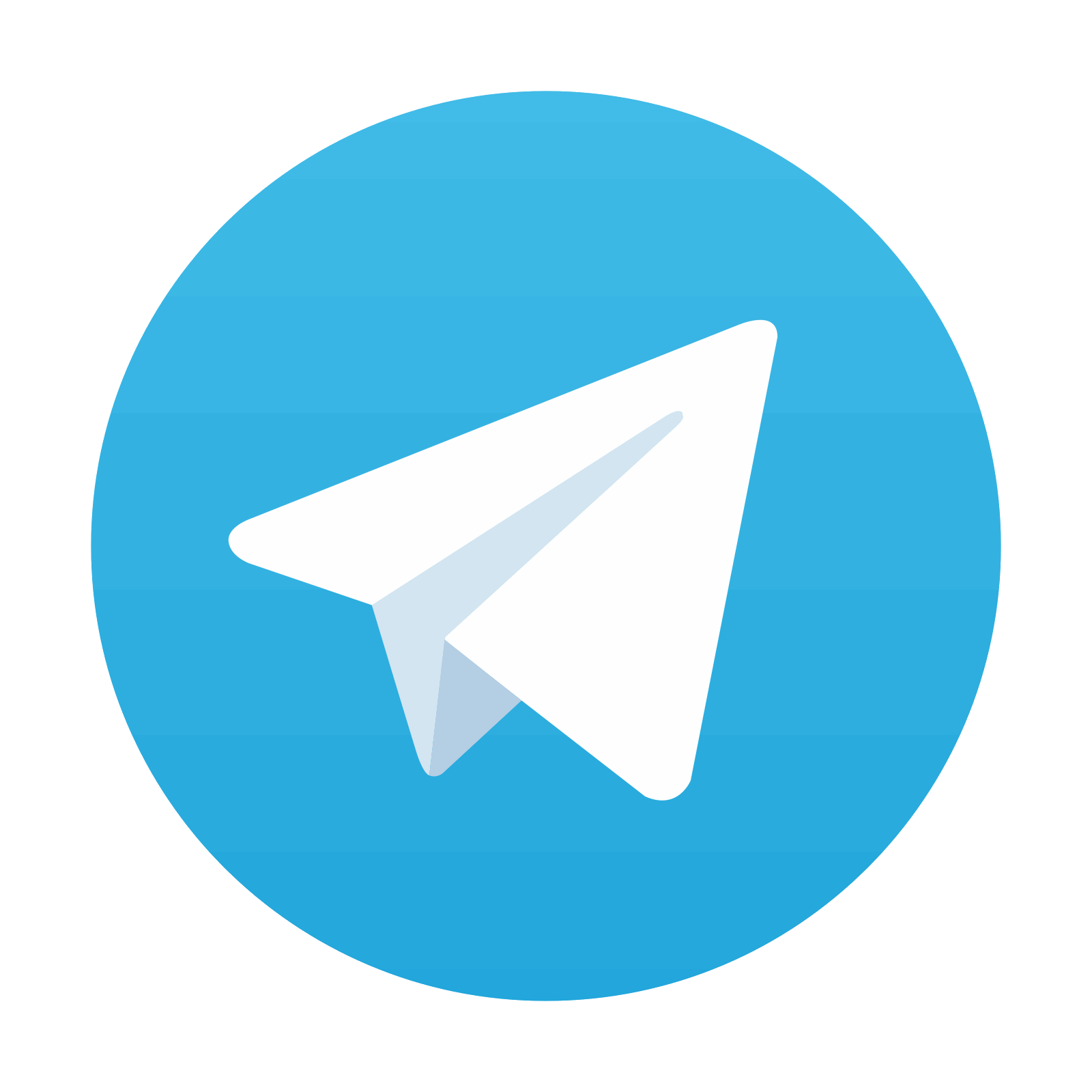
Stay updated, free articles. Join our Telegram channel

Full access? Get Clinical Tree
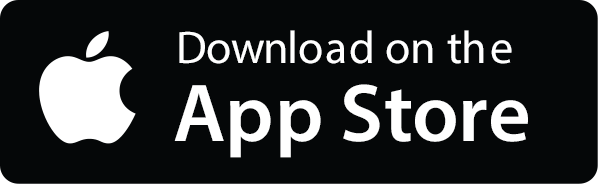
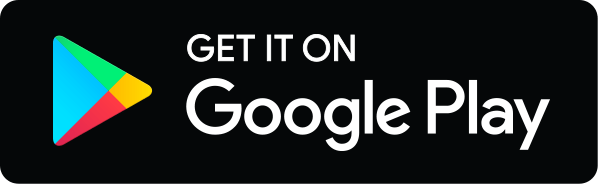