Cemented Femoral Component
John Charity
Rafael J. Sierra
Graham A. Gie
A. John Timperley
Properties of Acrylic Polymer
Cement is not a glue and has no adhesive properties, so that, as with cementless devices, the initial fixation of a cemented implant depends wholly on mechanical interlock. Unlike cementless implants, two interfaces are involved—one between the cement and the bone and one between the cement and the prosthesis. Load-induced, reversible cyclical movement can occur at either or both of these interfaces (1). Polymethylmethacrylate (PMMA) cement used in arthroplasty surgery is strong in compression and relatively weak in tension and has a low modulus of elasticity. Stainless steel and cobalt chrome alloys have a modulus of about 100 times and cortical bone about 10 times the value for PMMA, which is about 2 × 103 MPa. Interestingly, this value is very similar to the modulus of cancellous bone to which bone cement is fixed. To take advantage of its mechanical properties, PMMA bone cement should be loaded in compression as far as possible—the material should be interdigitated with cancellous bone on the endosteal surface of the femur with the interface protected by compressive forces.
Bone cement forms a complex composite material with cancellous bone in the main load transmission areas. The structure starts with 100% bone cement on the inside, through a composite of cement and bone, to 100% bone outside. Cancellous bone supports the cement, enabling it to transmit compression forces at one end by the cement and at the other by the bone. Bending, shear, and tension in bone cement should be avoided if possible. Bone cement has to function in patients for very long periods. Clinical experience with using bone cement extends to over 40 years (2,3). A comparison of the short-term strength of PMMA with the strength of cement samples recovered from patients after 15 to 24 years in vivo use has been reported (4). The tests reported show that cement retains acceptable mechanical properties even after considerable time functioning in vivo and that the time-dependent properties of creep and stress relaxation (described below) are still evident after such a long time in vivo. Combined with the long-term clinical results of hip replacements, these results show that bone cement functions satisfactorily in vivo for many years. The final in vivo life of PMMA cement (if it exists) has not yet been reached. It may be that through the phenomenon of stress relaxation of cement (vide infra) significant fatigue of the material may never become clinically relevant.
The two main long-term viscoelastic properties of PMMA bone cement that have to be considered are creep and stress relaxation.
Creep is defined as change in strain with time in a sample under constant load. Metals generally do not creep until they reach a temperature above 0.4 times their absolute melting temperature (i.e., about 400ºC for stainless steel). However, creep in polymers is significant at body temperature and must be considered when these materials are used in total joint replacements. The structure of the polymer allows creep to occur—PMMA is a linear, amorphous polymer that has its long chain molecules tangled together forming the bulk material. These long chains of PMMA are formed by molecules of methylmethacrylate (MMA) bonded together by strong covalent bonds. The chains are themselves linked by weaker secondary bonds that can be affected by environmental factors such as temperature. As the temperature increases from room temperature, through body temperature to a glass transition temperature (about 90ºC) the secondary bonds between the chains become progressively weaker allowing the chains to slide over each other. As time goes by, the creep rate reduces substantially until postpolymerization hardening is effectively complete at 4 to 6 weeks. Creep of cement does not stop even after extensive periods have passed—as evidenced by continued stem subsidence within the cement mantle at more than 25 years for long-term Exeter total hip stems (2).
Stress relaxation is defined as change in stress with time under conditions of constant strain. The mechanism inside the polymer that allows stress relaxation is, in principle, the same as the mechanism that allows creep. With stress relaxation, the material is deformed (putting the long chain molecules and the links between them under stress) and the deformation is locked into the material. While in the deformed state, the secondary bonds that are strained when the deformation is produced are able to slide over each other, gradually reducing the stress in the material. As with creep, all PMMA bone cements stress relax; stress relaxation occurs most readily under tension (where the secondary bonds are stretched and slide most easily) but can occur in conditions of compression and shear.
Principles of Cemented Implant Fixation and Load Transmission
The contrasting behavior of collarless polished tapered stems and the more conventional collared, rough-surfaced stems was rationalized by Shen (5) when he suggested that cemented femoral components could be divided into two basic types, the “taper-slip” stems (exemplified at that time by the polished flatback Charnley and the polished Exeter) which did routinely subside within the cement and the “composite beam” stems which, by definition, could not. These two stem designs became otherwise known as “force-closed,” in which stability is maintained by a balance of forces across the stem–cement interface, without the existence of a bond between the two, and “shape-closed,” in which stability is maintained by a bond that arises from matching the surface contours of the stem (i.e., its shape) and the cement that is applied to it (6).
In the case of “taper-slip” or “force-closed” stems, distal micromovement of the femoral component occurs solely at the implant–cement interface and is as part of the normal behavior of collarless polished tapered implants. Movement at the stem–cement interface protects the biologic cement–bone interface as the composite and interfaces all loaded primarily in compression. In contradistinction, with a “shape-closed” or composite beam stems the philosophy is to attempt to rigidly lock both the stem–cement and cement–bone interfaces together thereby creating a situation where the strains are identical at each interface. This is extremely difficult to achieve and RSA studies have shown the profound difference in migration pattern at each interface when comparing these types of stems (7,8,9,10
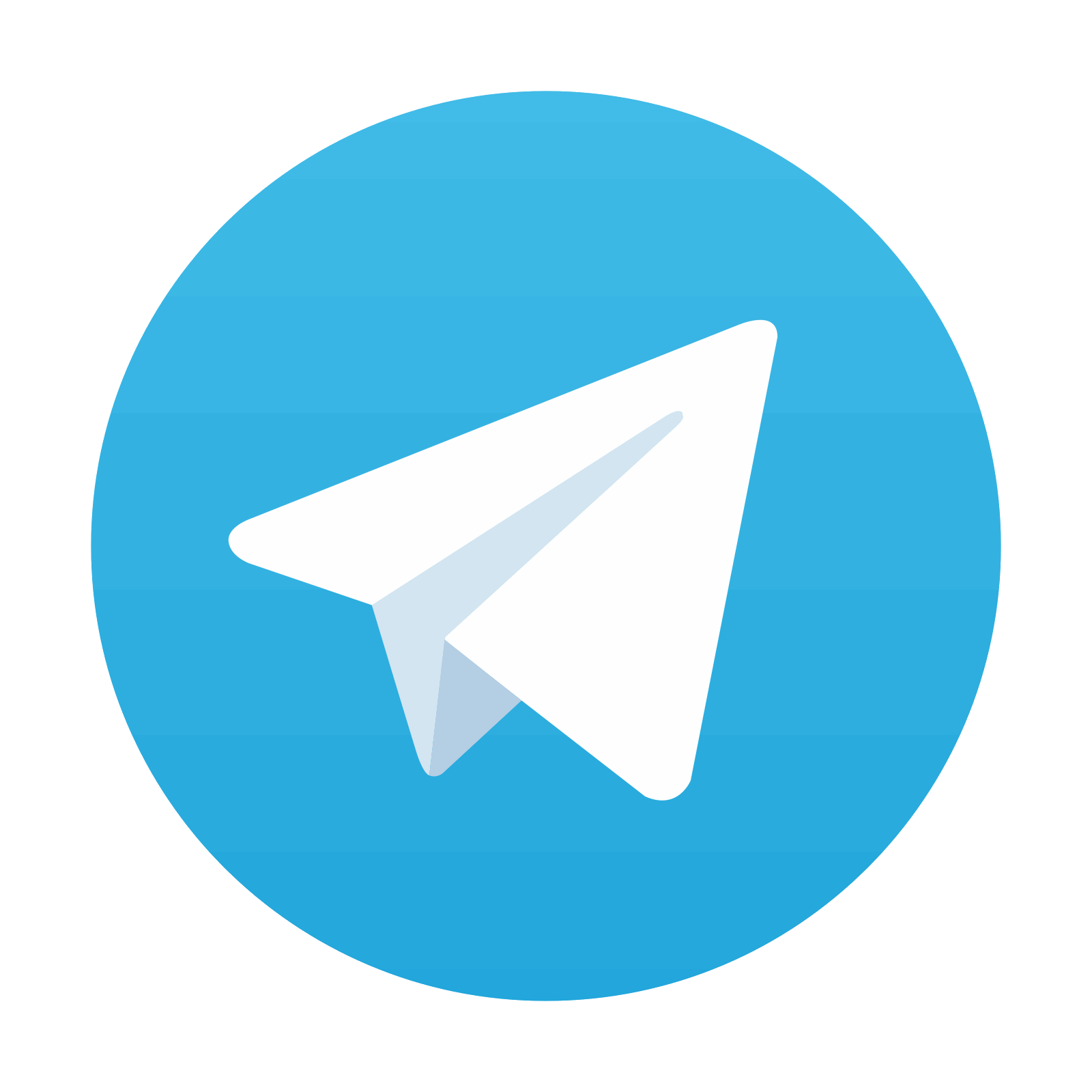
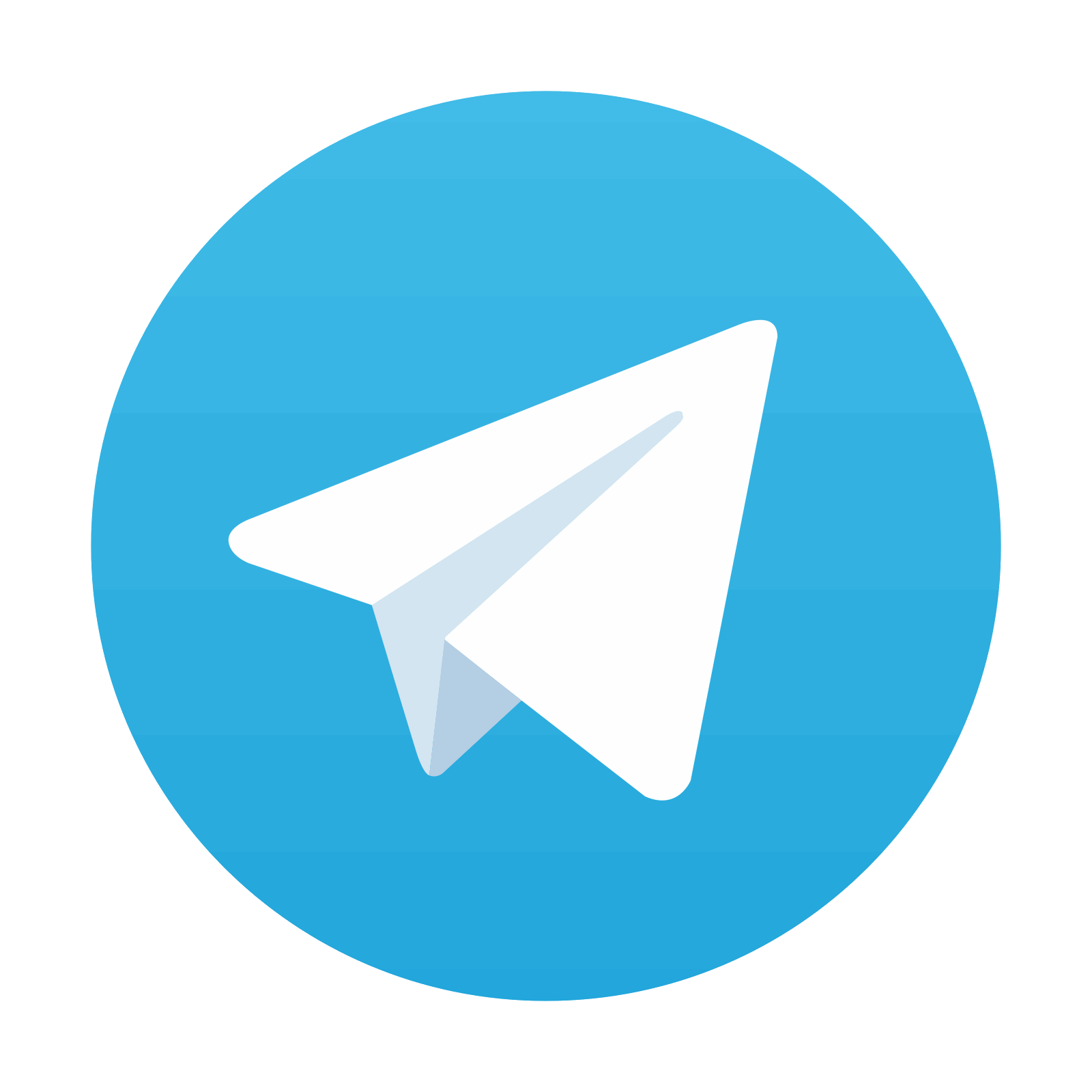
Stay updated, free articles. Join our Telegram channel

Full access? Get Clinical Tree
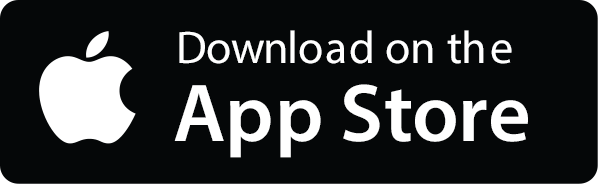
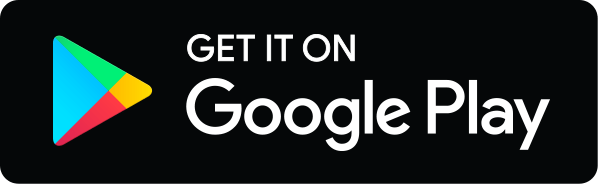