21
Cartilage Injury in the Skeletally Immature
Chondral and osteochondral injuries are being diagnosed with increasing frequency in skeletally immature patients.1 This is likely due to a combination of factors, including increased awareness of chondral injuries in general, increased participation of young children in organized sports at higher competitive levels, and improvements in magnetic resonance imaging (MRI) techniques for articular cartilage. Although chondral injuries in skeletally immature patients generally do not carry as grave a prognosis as similar injuries in skeletally mature patients, they are not inconsequential lesions. The consequences of childhood chondral injury have important long-term significance due to the young age of the patients.
This chapter reviews the diagnosis and management of juvenile osteochondritis dissecans, osteochondral fractures, and chondral injuries in skeletally immature patients.
Juvenile Osteochondritis Dissecans
Definition
Juvenile osteochondritis dissecans (JOCD) is a disorder involving the articular surface of a joint in a patient with open physes. Although it shares a similar pathophysiology with the adult form of osteochondritis dissecans (OCD), it is a distinct disease process that has a more favorable prognosis than the adult form and requires different management strategies.
Epidemiology
The onset of symptoms in JOCD can occur at any age during skeletal immaturity; however, adolescents are typically involved. The average age of patients at the time of diagnosis in published reports has ranged from 11.3 to 13.4 years, and there is some indication that this mean age is decreasing.2,3 This may reflect the increased involvement of children at younger and younger ages in competitive sports. The prevalence of JOCD was found by Linden4 to be 18/100,000 in females and 29/100,000 in males. A multicenter review found JOCD to affect 60% males and 40% females, although most previous reports have a male to female ratio of 2.5 to 4:1.5 This ratio may continue to decrease because more young girls are participating in athletics today than in previous generations. The most commonly affected joints in JOCD are the knee (distal femur), elbow (capitellum), and rarely the ankle (talus) with the majority of cases (75–85%) involving the knee.6,7 Both the right and left sides appear to be affected equally and lesions are bilateral 12 to 30% of the time.6,7 This disease appears to be rare in African Americans with only two cases in Cahill’s2 series of 240 patients. JOCD has also been associated with many other musculoskeletal abnormalities including Legg-Calvé-Perthes, skeletal dysplasias, tibia vara, patellar malalignment, Sinding-Larsen-Johansson disease, Osgood-Schlatter disease, and Stickler’s syndrome. These conditions may predispose the patient to developing JOCD by causing stress accumulation on the femoral condyles, which could provoke subchondral changes.
Pathology
Etiology
Since its initial description over 100 years ago, there has been controversy regarding the etiology of OCD. Various hypotheses have been proposed, but there is no universally accepted cause of the disease process and no theory completely explains its occurrence. The primary causes that have been proposed are trauma, genetic predisposition, defects of ossification, and circulatory disturbances causing osteonecrosis.
The most commonly accepted cause of OCD is trauma, with most authors believing that a single acute episode is not at fault but rather multiple episodes of microtrauma. These accumulated stresses over months or years result in crushing or fissuring of the subchondral bone with subsequent bone necrosis. One theory to explain this is that each child has a different stress acceptance threshold in each joint related to his/her tissue maturity. If the child is given a large enough repetitive stress over enough time on his/her immature tissue, this threshold can be exceeded resulting in JOCD. This theory is supported by the fact that many children with JOCD have a long history of exercise or sports-related activity and do not recall a single traumatic event. Another theory is that the tibial spine repeatedly impinges on the lateral aspect of the medial femoral condyle, causing a subchondral stress fracture. This theory, however, does not explain how lesions can occur in other aspects of the knee or in other joints.
Direct trauma, such as a blow to the knee, certainly could create an osteochondral injury, and biomechanical studies have shown that there is a significant amount of shear force in the region of the lateral aspect of the medial femoral condyle when the loaded knee is flexed. The symptoms of OCD in an adolescent are preceded by a history of trauma to the knee in 40 to 60% of cases. However, because JOCD lesions have a predilection for the posterolateral portion of the medial femoral condyle and because affected sites are located at specific locations rather than randomly distributed, it is more likely that indirect microtrauma is responsible for the majority of JOCD lesions than direct trauma. Although most evidence seems to support direct or indirect trauma as the primary contributory factor, the lack of traumatic history in many instances and a reported incidence of bilaterality in up to 30% of cases prevents trauma from adequately explaining every lesion.
Unlike OCD in the skeletally mature, OCD in the skeletally immature must be differentiated from variations of ossification or hereditary epiphyseal abnormalities. The difference in clinical picture between JOCD and OCD has led some to conclude that OCD in young patients may simply be a variant of normal growth.8,9 Some authors have proposed that OCD in young patients results from an abnormality of ossification that fails to heal. They believe that JOCD represents a basic disturbance of epiphyseal development with small accessory islets of bone being separated from the main osseous nucleus of the epiphysis. Because their blood supply is inadequate, minimal trauma may cause avascular necrosis of these bone islets leading to JOCD. Ribbing9 wrote that the mechanism of OCD was an accessory bone nucleus that separates in childhood with subsequent partial reattachment. Caffey et al8 also found marginal irregularities to be common in the regular epiphyseal outline of the distal femoral epiphysis with 66% of boys and 41% of girls having irregularities in the centers of ossification. Irregularity or roughening of the epiphyseal outline of the distal femoral physis may be a normal variant associated with periods of rapid skeletal growth. This seems to argue against the theory that abnormal ossification is a primary cause of OCD, as the epiphyseal defects seen in many patients with early lesions may have been a result of normal development.
Families with a predisposition for OCD in various joints have been documented in several studies but to date genetic factors have not been definitively shown to play a role in the development of OCD. Petrie10 found no definite genetic etiology for OCD in his review of 34 families. The isolated reports of familial involvement may actually be unrecognized cases of multiple epiphyseal dysplasia. Multiple epiphyseal dysplasia must be considered in all patients with JOCD, especially if there is a family history of OCD. Because multiple epiphyseal dysplasia has auto-somal dominant and recessive hereditary patterns, patients thought to have a familial form of OCD may actually have a variable expression of multiple epiphyseal dysplasia.
A final theory for the etiology of JOCD has been ischemia. Arterioles supplying small segments of subchondral bone become compromised by embolic phenomena, resulting in necrosis. This theory, although attractive, was refuted by the findings of Rogers and Gladstone (as reported by Cahill2), who revealed that the blood supply to the lower end of the femur is rich with numerous anastomoses in the cancellous bone, and that the subarticular areas of the distal femur are not supplied by end arteries.
The Juvenile Osteochondritis Dissecans Knee
Presentation
The most commonly affected joint in JOCD is the knee, with up to 85% of all cases being found there. The symptoms of OCD of the knee depend on the stage of presentation. Lesions early in their course are associated with poorly defined symptoms, and it may be difficult to differentiate a patient with osteochondral damage from a patient who has some other knee pathology, such as a meniscal tear, synovial problems, or extensor mechanism dysfunction. The presentation is often with vague, nonlocalized pain and ache. The initial disability often is only slight, but progresses before the diagnosis is made. The pain described is variable and intermittent, and typically increases with activity, especially running and jumping, but improves with rest. Parents may report a history of intermittent knee swelling associated with activity. Any degree of effusion after exercise should not be regarded as normal in this age group. As the lesion progresses, mechanical symptoms of catching, locking, and giving way are noted. The presence of loose bodies is associated with more constant symptoms of catching, locking, swelling, and pain. A patient rarely presents with an acutely locked knee due to a loose fragment as the initial manifestations of OCD.
Examination
The physical examination findings in JOCD also depend on the stage of the lesion at presentation. In the early stages, the physical examination can be nonspecific. There may be crepitus in the medial tibiofemoral compartment, and tenderness to palpation can be diffuse and poorly localized. As the lesion progresses, point tenderness may be present over the area of the lesion. The examiner should palpate both the medial and femoral condyles as well as the patella facet joint in an attempt localize the lesion. Axhausen described a sign of tenderness to palpation at the affected femoral condyle as the knee is brought into progressive degrees of flexion. The patient may also show loss of thigh circumference, antalgic gait, diminished range of motion, or an effusion. In a child with a lesion of the medial femoral condyle, careful examination of the child’s gait may reveal that the affected leg is held in a position of external rotation. This is a learned behavior in an attempt to avoid impingement of the tibial spine on the lateral aspect of the medial femoral condyle. The classic physical examination finding associated with OCD was described by Wilson (as reported by Cahill2). The sign is elicited by flexing the knee to 90 degrees, internally rotating the tibia, and then slowly extending the knee. A positive Wilson sign is pain at ~30 degrees of flexion that is relieved by external rotation of the tibia. Although this maneuver is well known, it should not be relied on as a pathognomonic finding. In a series of 509 affected knees, this sign was positive in only 16% of patients.2
Radiographic Imaging
If the history and physical examination are suspicious for JOCD, then radiographic studies are necessary to confirm the diagnosis. Initial radiographic evaluation should consist of standing anteroposterior, lateral, and tunnel views of the affected knee. A tunnel view with the knee in flexion commonly demonstrates a lesion that is not as easily seen on a standard weight-bearing anteroposterior film (Fig. 21–1). If a lesion is suspected to involve the patella, then the skyline view is helpful to identify lesions.
Radiographically, the lesion typically appears as a well-circumscribed area of sclerotic subchondral bone separated from the remainder of the epiphysis by a radiolucent line. Many JOCD patients present with radiographic findings only in the lateral view. The classic location for JOCD of the knee is in the lateral non—weight-bearing mid- to posterior portion of the medial femoral condyle. This location accounts for ~75% of JOCD lesions in the knee. The remaining 25% of lesions are divided between the lateral femoral condyle and the patella. Multiple sites have been noted up to 40% of the time. Harding described two lines drawn on the lateral radiograph: one along the posterior femoral cortex and the other along the Blumensaat line (intercondylar notch) (Fig. 21–2). These lines divide the articular surface of the distal femur into three sections, and the arc of the articular surface between these two lines is the area where JOCD of the medial femoral condyle is commonly found. Lesions involving the lateral femoral condyle tend to be slightly more posteriorly located.
FIGURE 21–1 Anteroposterior (AP) (A) and notch view (B) radiographs of the knee in the same patient with juvenile osteochondritis dissecans (JOCD). The typical lesion involves the posterior weight-bearing portion of the lateral aspect of the medial femoral condyle and can be better appreciated on the notch view.
FIGURE 21–2 Lateral radiograph is useful in detecting osteochondritis dissecans (OCD). One line is drawn along the posterior cortex of the femur (A), and another along Blumensaat’s line (B). (Adapted from Harding .)
Prior to the introduction of MRI, a technetium-99m phosphate compound bone scan was used as the next step in the investigation of OCD (Fig. 21–3). The bone scan is highly sensitive, can be positive as early as 12 hours after injury, identifies the presence of bilateral involvement, and is relatively inexpensive.2 It is a helpful test in patients suspicious for OCD with negative radiographic findings. Cahill2 recommends using the bone scan as the method of choice for following the progress of the disease. He has noted that the degree of osseous uptake on bone scan is related to the regional blood flow, and, therefore, the potential for healing of the fragment. He advises scanning the patient at 4-month intervals to monitor changes in the lesion’s activity. Parallel-hole scans are to be obtained only with the initial scan with the much more sensitive and detailed pin-hole technique used thereafter. Paletta et al11 also found the bone scan to be predictive for prognosis of nonsurgical treatment in patients with open physes. The patients with increased activity on bone scan went on to heal their lesions nonoperatively.
FIGURE 21–3 Bone scan of bilateral lateral femoral condyle JOCD demonstrating increased uptake within bilateral lateral distal femoral epiphyses.
FIGURE 21–4 Magnetic resonance imaging (MRI) of JOCD of the knee. AP (A) and lateral (B) radiographs. Corresponding coronal (C) and sagittal (D) MRI images demonstrating an intact articular surface with clear demarcation of the underlying bony lesion.
The disadvantages of a bone scan, however, are that it cannot quantify the exact size of the lesion or determine the condition of the articular cartilage. This is where an MRI can supply valuable information either as a supplement to the bone scan or as a primary study (Fig. 21–4). MRI is useful not only for identifying the location and extent of the suspected lesion, but also for determining the involvement and integrity of the articular cartilage.12 It facilitates determining whether there is detachment of a fragment. If there is a joint effusion, the presence of fluid behind the fragment can clearly identify partial or complete detachment. If there is not an effusion, then intraarticular gadolinium can be used to enhance a detached lesion. This information is important clinically, because it can change the management of the patient. An MRI also provides important supplemental information such as the detection of subtle bone injuries, the integrity of the ligaments and menisci, and the presence or absence of any associated intraarticular osseous and cartilaginous bodies. Over the past 10 to 15 years, improvements in the techniques of acquiring images with MRI have increased our ability to diagnose articular cartilage injuries in the knee noninvasively. Loredo and Sanders13 feel the optimal technique for evaluation of cartilage is a spoiled gradient-echo sequence using fat suppression and three-dimensional acquisition. With this sequence, spatial resolution is high, owing to the ability to obtain thin contiguous slices; the images can be reformatted in multiple planes, and there are high contrast-to-noise ratios between cartilage and fluid and between cartilage and bone. Despite these advancements, however, MRI is still not as sensitive or specific as arthroscopy in assessing articular cartilage injury.12 Arthroscopy remains the gold standard and may be especially useful in the management of patients for whom MRI scans of good quality or intraarticular gadolinium arthrography is not available.
Classification
Radiographic studies have been used in an attempt to classify JOCD lesions. There have been multiple classification systems developed for JOCD, but there is no universally accepted system. Cahill2 and Berg devised a classification based on the appearance of the lesion on bone scan (Table 21–1). MRI has also been used as the basis for a classification scheme with increasing stages indicating worsening of the lesion (Table 21–2). Finally, the lesions can be classified on the basis of surgical findings.5 This classification is based on visual and palpable observations at surgery and is designed to allow accurate description of the lesion. The lesion is first classified under one of three categories: (1) intact cartilage, (2) disrupted cartilage, and (3) macerated cartilage. If there is intact cartilage, then one must determine if it is a stable or unstable lesion. If there is disrupted cartilage, then one can again examine to see if it is stable or unstable. If it is deemed to be unstable, then it falls into one of three types: predetachment, hinged, or loose body. Finally, if the cartilage is found to be macerated, then it can be subclassified as discolored, blistered, abraded, or fragmented.
Stage 0: Normal radiographic and scintigraphic appearance. |
Stage I: The lesion is visible on plain radiographs, but bone scans reveal normal findings. |
Stage II: The scan reveals increased uptake in the area of the lesion. |
Stage III: In addition, there is increased isotopic uptake in the entire femoral condyle. |
Stage IV: In addition, there is uptake in the tibial plateau opposite the lesion. |
Management
The management of JOCD is controversial. The data in the current literature regarding the ability of JOCD-involved femoral condyles to heal and be restored to normalcy is divided between those saying that no treatment is necessary and those believing that most patients need surgery.14–17 Although it is true that all recent authors agree that children with open physes have a much higher frequency of spontaneous resolution of this condition than adults, JOCD is not an entirely benign condition.14–17 The overall success rate of nonoperative treatment is ~50%.14–17 Once the diagnosis of JOCD is confirmed, the race for healing of the lesion before the distal femoral physis closure begins. If the contest is lost, and JOCD evolves into OCD with the potential for nonunion and detachment, then the favorable prognosis is lost. Consequently, for conservative treatment to have the best prognosis, JOCD must be diagnosed early. Two additional factors besides the age of the patient that have been found to affect the prognosis of JOCD are the size and location of the lesion. The larger the size of the lesion, the higher the likelihood of developing osteoarthritis according to Twyman et al.16 Their work also agrees with that of Hefti et al,5 who found that the chances of healing were better when the lesion was located at the classic site (lateral part of medial femoral condyle) rather than at other sites.
Stage I: Small change of signal without clear margins of fragment. |
Stage II: Osteochondral fragment with clear margins but without fluid between fragment and underlying bone. |
Stage III: Fluid is visible partially between fragment and underlying bone. |
Stage IV: Fluid is completely surrounding the fragment, but the fragment is still in situ. |
Stage V: Fragment is completely detached and displaced (loose body). |
Most clinicians agree that initial treatment for patients with JOCD should be nonoperative unless the lesion is unstable, a loose body is present, or the patient is within 8 months of physis closure. The goal of conservative treatment is to obtain lesion healing before physis closure to prevent potential early-onset gonarthrosis. Provided the patient is compliant and the lesion remains stable, the success of conservative treatment of JOCD, as judged by radiographic healing of the lesion, has been reported to vary between 50% and 91%.14–17 Therefore, a trial of adequate conservative treatment is appropriate. Nonoperative treatment options range from “watchful waiting” and activity modification to non—weight-bearing and immobilization with trials lasting from 6 to 18 months. The basic principle of nonoperative treatment is reduction of exercise to a level where symptom-free activities of daily living are possible.18 In a report by Sales de Gauzy et al,3 30 of 31 lesions healed over an average of 11 months by simply discontinuing the child’s involvement in sports activities until the pain had stopped.
If there is pain with ambulation, then crutches are used for 6 to 8 weeks with weight bearing as tolerated. During this time, no competitive sports or running is allowed. Because it can be difficult to restrict the activity of a child, removable splints or a knee immobilizer with intermittent knee motion may be used. Some clinicians utilize casts routinely; however, the deleterious effects of immobilization on cartilage nutrition and the potential for stiffness and atrophy should be considered. The results of unloader braces have not been established. Close clinical observation with serial radiographic studies is important. The choice of which radiographic study to use is clinician dependent. Radiographs or MRIs can be used to follow healing of the lesion. Cahill2 recommends using a limited bone scan every 8 weeks in combination with physical examination until clinical and scintigraphic examinations demonstrate that healing has occurred.
Once the child has reached the symptom-free level, the crutches can be discarded. As healing progresses, activities such as recreational cycling, swimming, and lower extremity closed kinetic-chain strength training are added, provided the child remains asymptomatic. Successful conservative treatment is indicated by a normal orthopaedic examination, especially the absence of swelling. If the child remains compliant, most lesions will heal within 10 months. However, some slow-healing lesions can take up to 18 months of nonoperative treatment to heal. Patient compliance with such prolonged activity restriction can be problematic. In Cahill’s2 experience, many of the 50% of patients that failed conservative treatment were noncompliant. He stresses the importance of educating the patient and the patient’s family about the disease process and makes the point that both nonoperative and surgical treatments require similar periods of time restriction from sports participation. It is exceedinly difficult, however, in a society that demands instant gratification to convince young athletes and their parents to remain out of competitive sports for such an extended period of time. To suggest conservative treatment that could last for 1 year, and would eliminate competitive sports for that time, often surprises and disheartens the family. Consequently, many clinicians compromise and continue nonoperative management of stable JOCD lesions for approximately 6 months. At that point, if the child is still symptomatic, surgical intervention can be used. It can also be argued that excessive delay in operative intervention may allow some adolescents to approach skeletal maturity, thus giving them a worse prognosis for healing and eventual outcome.
Operative intervention is warranted if loose bodies are present, if detachment or instability of the fragment occurs while the patient is under conservative treatment, if there is persistence of symptoms for 6 to 12 months in a compliant patient undergoing conservative treatment, if epiphyseal closure is approaching, or if healing is unlikely as determined on the basis of bone scans, MRI, and clinical judgment.14–17
The basic tenets of operative treatment include anatomic restoration of the congruity of joint surfaces, enhancement of revascularization to the fragment or crater, and fixation of unstable fragments to permit early joint motion.14–17 Surgical options depend on the lesions involved and include drilling (retrograde transarticular or antegrade through the distal femoral epiphysis), curettage, bone grafting, internal fixation, open or arthroscopic reduction of a loose fragment with internal fixation, fragment removal, autologous or allogenic osteochondral grafting, and autologous chondrocyte implantation.
Arthroscopy has become a valuable tool for evaluating the size, location, and stability of the fragment and is less invasive than open arthrotomy. Direct probe of the affected area under arthroscopic control reveals the character of the articular surface at the lesion margins and permits evaluation of the underlying bone’s stability. The development of arthroscopic probes that measure the viscoelastic or electrostatic properties of articular cartilage holds promise. Each case should be decided on an individual basis depending on the lesion size and location. If at arthroscopy, the lesion is found to be stable, arthroscopic drilling without fixation is the treatment of choice. This treatment method theoretically offers the potential to create channels for revascularization and healing (Fig. 21–5). Transarticular drilling is accurate and technically straightforward. Kocher et al14 reported significant improvement in functional outcome after transarticular drilling, with radiographic healing occurring at an average of 4.4 months after drilling. The one drawback with this technique, however, is that it creates channels in the articular cartilage that heal with fibrocartilage, not hyaline cartilage. For this reason, the technique of antegrade drilling through the epiphysis has been advocated as a way to avoid this problem. This technique, unfortunately, has its own disadvantages, in that it is much more technically difficult, it tends to be inaccurate, and it can be hard to gauge the depth of penetration while drilling. Whichever drilling method is chosen, range of motion with protected weight bearing should be instituted immediately after surgery.
FIGURE 21–5 Arthroscopic transarticular drilling for a stable JOCD lesion of the knee with an intact articular surface.
If the lesion is found at arthroscopy to be unstable or is partially detached, then the recommended treatment should be curettage of the femoral defect, drilling of the defect, and stable internal fixation of the lesion. Cancellous grafting may also be necessary to increase vascularity or to fill subchondral cavity defects. When the lesion has been partially detached or loose for months, a sclerotic rim of bone is common. In this instance, drilling alone will not improve the outcome. The crater base should be curetted to bleeding bone and then drilled. The subchondral bone of the fragment may be sclerotic and require curetting, as well. These lesions should then undergo mechanical stabilization to increase the likelihood of maintaining joint congruity during healing, and to allow the potential benefit of early motion. Selection of the optimal device for stabilization of the lesion is debated. The options for internal fixation for JOCD include Kirschner wires, variable pitch compression screws, cannulated screws, bone pegs, absorbable compression devices, and absorbable pins (Fig. 21–6). Postoperatively, range-of-motion exercises are started at once, but protected weight bearing is necessary for 3 months.
Skeletally immature patients with loose bodies should be managed in a manner similar to adults. If the lesion is from a weight-bearing area, it is imperative that it be replaced. For chronic loose bodies there may be a size mismatch between the loose body and the crater, making replacement challenging (Fig. 21–7). The results of removal in long-term follow-up have been poor. The fibrous and fibrocartilaginous tissue that ultimately fills the JOCD crater after treatment is not hyaline cartilage and has a decreased ability to withstand weight-bearing stresses. If internal fixation of the lesion is impossible, either because it is too macerated or because the articular cartilage has been destroyed, then the lesion should be removed and chondral resurfacing should be considered. Resurfacing techniques include abrasion arthroplasty, microfracture, mosaicplasty, osteochondral allograft, and autologous chondrocyte implantation. To date, there are no published data regarding the outcome of allografts, autografts, or chondrocyte implantation specifically in skeletally immature patients. These methods, however, are recommended in salvage situations.
Epidemiology of Osteochondritis Dissecans of the Elbow
Osteochondral injury of the capitellum affects two different age groups in the skeletally immature. In children between the ages of 4 and 8 years, Panner’s disease, an osteochondrosis often involving the entire capitellum, is seen. In adolescent athletes, the more traditional OCD lesion can be found.
Panner’s Disease
Patients with Panner’s disease present with a history of dull, aching pain in the elbow not associated with trauma.19 There may also be decreased range of motion, swelling, and tenderness of the elbow. On exam, there are no specific findings, but an effusion, limited motion, and tenderness over the lateral aspect of the elbow may be noted. The key to making a diagnosis is the radiographs, which reveal rarefaction and fragmentation of the entire ossific nucleus of the capitellum. The disease is thought to share a similar pathophysiology with Legg-Calvé-Perthes disease. Fortunately, Panner’s disease is generally a self-limiting process that resolves with rest and splinting. In most cases, reconstitution of the capitellum occurs without late sequelae or limitations.
FIGURE 21–6 Surgical management of JOCD of the knee with absorbable implants (A), wires (B), variable-pitch compression screws (C), and autologous chondrocyte implantation (D).
Osteochondritis Dissecans of the Capitellum
The typical patients are adolescent males involved in throwing sports and female gymnasts. The mechanism is thought to be repetitive microtrauma. Valgus overload results in tensile forces in the medial elbow and compressive forces in the lateral elbow.19–23 The mechanical disparity of compressive stiffness between the radial head and the capitellum causes increased strain on the softer capitellar surface. This is thought to lead to breakdown and avascularity of the subchondral bone of the capitellum producing an OCD lesion.19–23 Ischemia has also been hypothesized to be a possible etiology of capitellar JOCD.19–23 The blood supply to the capitellar chondroepiphysis enters posteriorly and has no communication with the metaphyseal vessels. This fragile arrangement may become compromised with the resulting ischemia producing OCD of the capitellum.
FIGURE 21–7 Loose body associated with juvenile osteochondritis dissecans of the knee.
FIGURE 21–8 Osteochondritis dissecans of the capitellum: AP radiograph (A) and sagittal MRI (B).
The presentation of capitellar OCD is one of insidious onset with poorly localized, diffuse joint pain. Symptoms are intermittent, increasing with activity and improving with rest. In patients who have more advanced irregularity of the articular surface, more dramatic symptoms, such as catching, clicking, giving way, and grinding may be reported. If loose bodies are present, true locking of the joint may occur. Physical examination can reveal an effusion and tenderness to palpation over the lateral elbow and capitellum. A decreased range of motion may be present with a lack of full extension being the most common finding.
Plain anteroposterior and lateral radiographs reveal the characteristic rarefaction or radiolucency of the lateral or central portion of the capitellum (Fig. 21–8). In contrast to Panner’s disease, only a portion of the ossific nucleus of the capitellum is involved. Hypertrophy of the radial head and loose bodies are sometimes seen in the later stages. MRI can help make the diagnosis if there is still suspicion after obtaining plain radiographs. MRI also allows evaluation of the stage of the lesion (Fig. 21–8).
Treatment consists of rest and activity modification. Lesions that have not fragmented may heal if protected from additional trauma. The prognosis is improved if the patient is less than 13 years of age and if the lesion is not advanced. Loose fragments can be removed by arthroscopic or open methods, and the capitellar defect can be drilled. The results from fragment fixation have been disappointing.
Osteochondral Fractures
Osteochondral fractures in skeletally immature patients are more common than once thought. They most frequently involve the knee joint and are typically associated with acute patellar dislocations. The prevalence of osteochondral fractures associated with acute patella dislocation ranges from 25 to 50%.24–29 Matelic et al26 found 67% of children presenting with an acute hemarthrosis of the knee had an osteochondral fracture. The most common locations for these fractures are the medial patellar facet (Fig. 21–9) or the lateral femoral condyle.24–29
Etiology
A histopathologic study by Flachsmann et al30 helps to explain the occurrence of osteochondral fractures in the skeletally immature at a cellular level. They noted that in the joint of a juvenile, interdigitating fingers of uncalcified cartilage penetrate deep into the subchondral bone, providing a relatively strong bond between the articular cartilage and the subchondral bone. In the adult, the articular cartilage is bonded to the subchondral bone by the well-defined calcified cartilage layer— the cement line. When shear stress is applied to the juvenile joint, the forces are transmitted into the subchondral bone by the interdigitating cartilage, with the resultant bending forces causing the open pore structure of the trabecular bone to fail. In mature tissue, the plane of failure occurs between the deep and calcified layers of the cartilage—the tidemark—leaving the osteochondral junction undisturbed. Although the juvenile and adult tissue patterns are different, they both provide adequate fracture toughness to the osteochondral region. As the tissue transitions, however, from the juvenile to the adult pattern during adolescence, the fracture toughness is lost. The calcified cartilage layer is only partially formed and the interdigitating cartilage fingers are progressively replaced with calcified matrix. Consequently, the interface between the articular cartilage and the subchondral bone becomes a zone of potential weakness in the joint, which may explain why osteochondral fractures are seen frequently in adolescents and young adults.
There are two primary mechanisms for production of an osteochondral fracture.24–30 First, a direct blow to the knee with a shearing force applied to either the medial or lateral femoral condyle can create an osteochondral fracture. The second mechanism involves a flexion-rotation injury of the knee in which an internal rotation force is placed on a fixed foot, usually coupled with a strong quadriceps contraction. The subsequent contact between the tibia and femur or patella and lateral femoral condyle causes the fracture. An example of this mechanism is an acute patellar dislocation. As the patella dislocates, the medial retinaculum tears but the remaining quadriceps muscle-patellar ligament complex still applies significant compressive forces as the patella dislocates laterally and shears across the lateral femoral condyle. The medial border of the patella then temporarily becomes impacted on the prominent edge of the lateral femoral condyle before it slides back tangentially over the surface of the lateral femoral condyle due to pull of the quadriceps. Either the dislocation or the relocation phase of this injury can cause an osteochondral fracture to the lateral femoral condyle, the medial facet of the patella, or both. Interestingly, osteochondral fractures are uncommon with chronic, recurrent subluxation or dislocation of the patella. In this situation, the laxity of the medial knee tissues and decreased compressive forces between the patella and the lateral femoral condyle prevents development of excessive shear forces.
History and Physical Examination
Osteochondral fractures present with severe pain, swelling, and difficulty with weight bearing. On examination, tenderness to palpation over the medial femoral condyle, lateral femoral condyle, or medial patella is exhibited. The patient usually resists attempts to flex or extend the knee and may hold the knee in 15 to 20 degrees of flexion for comfort. The large hemarthrosis is due to fracturing the highly vascular subchondral bone. A joint aspiration reveals a supernatant layer of fat, if allowed to stand for 15 minutes, indicating an intraarticular fracture. Late examination findings may be similar to those of a loose body with intermittent locking or catching of the knee.
Radiographic Imaging
Radiographic visualization of the osteochondral fracture should begin with anteroposterior and lateral plain radiographs. A skyline view of the patella is mandatory if the patient has suffered an acute patellar dislocation. However, a roentgenographic diagnosis can be difficult because even a large osteochondral fragment may contain only a small ossified portion that is visible on plain radiographs. Matelic et al26 report that standard radiographs failed to identify the osteochondral fracture in 36% of children who had an osteochondral fracture found during arthroscopy. For this reason, supplemental studies such as MRI or computed tomography (CT) arthrography may be necessary in cases where there is a high suspicion of osteochondral fracture despite negative radiographs.31,32 Arthroscopic examination can also be done as the definitive diagnostic (and potentially therapeutic) test. With regard to plain film findings, two studies have reported that having a high-riding patella seems to have a protective effect against associated intraarticular osteochondral fractures. Patients with an Insall index >1.3 have a decreased chance of sustaining an osteochondral fracture compared with patients who have an Insall index within normal limits.31
FIGURE 21–9 Osteochondral fracture of the medial patella facet associated with acute patella dislocation in a 12-year-old boy. Skyline radiograph (A) and axial MRI (B) demonstrating lateral patella subluxation with osteochondral fracture. (C) Arthroscopic appearance of osteochondral fragment. (D) Appearance of the medial patella defect through an open lateral retinacular release. The osteochondral fragment (E) was replaced and fixed using two 3.5-mm cannulated screws (F,G). Healed osteochondral fracture seen through the lateral arthrotomy at the time of screw removal, 6 weeks after fixation (H).
Management
The recommended management of acute osteochondral fractures of the knee is either surgical removal of the fragment or fixation of the fragment to its anatomic location.33 If the lesion is large (>1 cm), easily accessible, involves a weight-bearing area, and has adequate cortical bone attached to the chondral surface, then fixation should be attempted (Fig. 21–9). This can be done via arthroscopy or arthrotomy with smooth or threaded Steinmann pins or screws countersunk below the articular surface. If the fracture fragment is small, loose, and from a non—weight-bearing region of the knee, then arthroscopic excision is recommended. The fragment’s crater should be debrided to stable edges, and the underlying subchondral bone should be perforated to encourage fibrocartilage formation. In patients with an osteochondral fracture after acute patellar dislocation, concomitant repair of the medial retinaculum at the time of fragment excision or fixation produces the best results. Postoperatively, patients treated by excision of the fragment can begin range-of-motion exercises immediately. Crutches may be necessary in the immediate postoperative period but patients can progress to weight bearing as tolerated. If the patient has the fragment fixed, initial immobilization with protected weight bearing is necessary. Weight bearing is gradually progressed and full weight bearing is allowed when the swelling has subsided and radiographs show evidence of fracture healing. Return to athletic activities is permitted when full range of motion is recovered and quadriceps strength is symmetric.
Chondral Injuries
Chondral injuries can occur in skeletally immature patients although the exact incidence of these injuries is unknown, because they can be difficult to diagnose. Chondral injuries are caused by major compression and shear forces at the articular surfaces such as occurs in acute patellar dislocations. Stanitski and Paletta25 used four-view radiographs and arthroscopy to evaluate 48 adolescent patients with acute patellar dislocations and found only two purely chondral injuries (4%).
If after a complete history, physical exam, and plain radiographs, a chondral injury is suspected, then an MRI is recommended to evaluate the status of the articular cartilage. Gradient-echo sequences appear especially helpful in identifying chondral lesions and should be obtained when available.31 Despite the improvements in imaging of articular cartilage, arthroscopy still remains the gold standard for identifying chondral injuries. Arthroscopy has the potential to be both diagnostic and therapeutic and can be used in situations where MRI is not available.
Treatment of chondral injuries in the skeletally immature generally uses the same management strategies as in adults, but there are a few differences that must be taken into consideration when managing a child with this injury. First, although the surgical procedures developed to repair cartilage damage in skeletally mature patients (abrasion chondroplasty, microfracture, transarticular drilling, articular cartilage autografting, autologous chondrocyte implantation, and allografting) have the same potential benefit in skeletally immature patients, there is little data in the literature to support one procedure over another in this age group. Our preference is to attempt microfracture first, and then, if this is unsuccessful, to use autologous chondrocyte implantation as a salvage procedure.
Second, when operating on the skeletally immature, there are a few technical points that must be remembered that are not ordinarily addressed in adults. When performing marrow stimulation techniques, such as drilling or microfracture, the surgeon must be cognizant of the proximity of the growth plate to prevent potential growth disturbances from inadvertent violation of the physis. Similarly, when autologous chondrocyte implantation is attempted, it is important not to disrupt the tibial tubercle apophysis while harvesting periosteum. Another difference in the management of skeletally immature chondral injuries is that most of these procedures require a period of protected weight bearing postoperatively. This can be difficult to enforce in younger children because they may not be able to follow complex rehabilitation programs. Consequently, the surgeon may need to modify the rehabilitation program to fit the patient’s capabilities. Finally, although it is generally believed that children have greater reparative abilities than adults, they also have a longer remaining life span than adults. This means the implications of a full-thickness chondral injury and its repair are more important. Furthermore, the longevity of chondral resurfacing techniques is of utmost importance. It is especially important to attempt to re-create an anatomic articular surface with hyaline cartilage in a young patient who will need a lifetime of use from the joint.
REFERENCES
1. Kocher MS, Micheli LJ. The pediatric knee: evaluation and treatment. In: Insall JN, Scott WN, eds. Surgery of the Knee, 3rd ed. New York: Churchill-Livingstone, 2001:1356–1397
2. Cahill BR. Osteochondritis dissecans of the knee: treatment of juvenile and adult forms. J Am Acad Orthop Surg 1995;3:237–247
3. Sales de Gauzy J, Mansat C, Darodes PH, et al. Natural course of osteochondritis dissecans in children. J Pediatr Orthop 1999;8:26–28
4. Linden B. The incidence of osteochondritis dissecans in the condyles of the femur. Acta Orthop Scand 1976;47:664–667
5. Hefti F, Beguiristain J, Krauspe R, et al. Osteochondritis dissecans: a multicenter study of the European Pediatric Orthopaedic Society. J Pediatr Orthop 1999;8B:231–245
6. Schenk RC, Goodnight JM. Osteochondritis dissecans. J Bone Joint Surg 1996;78-A:439–456
7. Clanton TO, DeLee JC. Osteochondritis dissecans. Clin Orthop Relat Res 1982;167:50–64
8. Caffey J, Madell SH, Royer C, et al. Ossification of the distal femoral epiphysis. J Bone Joint Surg 1958;40-A:647–654
9. Ribbing S. The hereditary multiple epiphyseal disturbance and its consequences for the aetiogenesis of local malacias—particularly the osteochondrosis dissecans. Acta Orthop Scand 1955;24: 286–299
10. Petrie PW. Aetiology of osteochondritis dissecans. Failure to establish a familial background. J Bone Joint Surg Br 1977;59:366–367
11. Paletta GA Jr, Bednarz PA, Stanitski CL, et al. The prognostic value of quantitative bone scan in knee osteochondritis dissecans. A preliminary experience. Am J Sports Med 1998;26:7–14
12. Kocher MS, DiCanzio J, Zurakowski D, et al. Diagnostic performance of clinical examination and selective magnetic resonance imaging in the evaluation of intraarticular knee disorders in children and adolescents. Am J Sports Med 2001;29:292–296
13. Loredo R, Sanders TG. Imaging of osteochondral injuries. Clin Sports Med 2001;20:249–278
14. Kocher MS, Micheli LJ, Yaniv M, et al. Functional and radiographic outcome of juvenile osteochondritis dissecans of the knee treated with transarticular arthroscopic drilling. Am J Sports Med 2001;29:562–566
15. Carroll NC, Mubarak SJ. Juvenile osteochondritis dissecans of the knee. J Bone Joint Surg 1977;59-B:506
16. Twyman RS, Desai K, Aichroth PM. Osteochondritis dissecans of the knee. J Bone Joint Surg 1991;73-B:461–464
17. Cain EL, Clancy WG. Treatment algorithm for osteochondral injuries of the knee. Clin Sports Med 2001;20:321–341
18. Cahill BR, Ahten SM. The three critical components in the conservative treatment of juvenile osteochondritis dissecans. Clin Sports Med 2001;20:287–298
19. Kocher MS, Waters PM, Micheli LJ. Upper extremity injuries in the paediatric athlete. Sports Med 2000;30:117–135
20. Baumgarten TE, Andrews JR, Satterwhite YE. The arthroscopic classification and treatment osteochondritis dissecans of the capitellum. Am J Sports Med 1998;26:520–523
21. Pappas AM. Elbow problems associated with baseball during childhood and adolescence. Clin Orthop 1982;164:30–41
22. Bauer M, Jonsson K, Josefsson PO, et al. Osteochondritis dissecans of the elbow: a long term follow-up study. Clin Orthop 1992;284:156–160
23. Ruch DS, Cory JW, Poehling GG. The arthroscopic management of osteochondritis dissecans of the adolescent elbow. Arthroscopy 1998;14:797–803
24. Nietosvaara Y, Aalto K, Kallio PE. Acute patellar dislocation in children: incidence and associated osteochondral fractures. J Pediatr Orthop 1994;14:513–515
25. Stanitski CL, Paletta GA. Articular cartilage injury with acute patellar dislocation in adolescents. Am J Sports Med 1998;26: 52–55
26. Matelic TM, Aronsson DD, Boyd DW, et al. Acute hemarthrosis of the knee in children. Am J Sports Med 1995;23:668–671
27. Farmer JM, Martin DF, Boles CA, et al. Chondral and osteochondral injuries. Clin Sports Med 2001;20:299–319
28. Alleyne KR, Galloway MT. Management of osteochondral injuries of the knee. Clin Sports Med 2001;20:343–363
29. Birk GT, DeLee JC. Osteochondral injuries. Clin Sports Med 2001;20:279–287
30. Flachsmann R, Broom ND, Hardy AE, et al. Why is the adolescent joint particularly susceptible to osteochondral shear fracture? Clin Orthop Relat Res 2000;381:212–221
31. Bohndorf K. Imaging of acute injuries of the articular surfaces (chondral, osteochondral, and subchondral fractures). Skeletal Radiol 1999;28:545–560
32. Wessel LM, Scholz S, Rusch M, et al. Hemarthrosis after trauma to the pediatric knee joint: what is the value of magnetic resonance imaging in the diagnostic algorithm? J Pediatr Orthop 2001;21: 338–342
33. Menche DS, Vangsness CT, Pitman M, et al. The treatment of isolated articular cartilage lesions in the young individual. Instr Cours Lect 1998;47:505–515
< div class='tao-gold-member'>
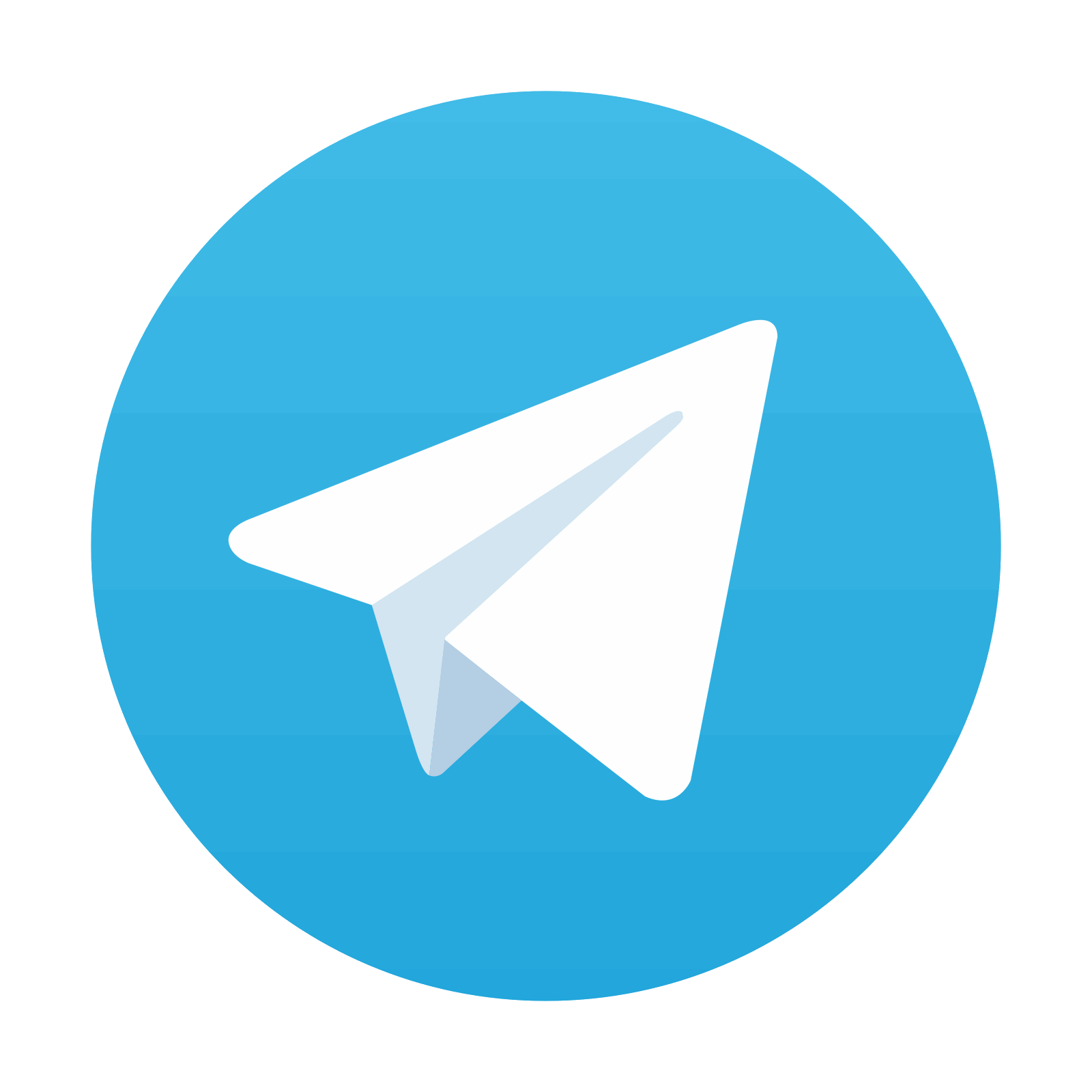