Essential amino acids
Conditionally essential amino acids
Nonessential amino acids
Isoleucine
Arginine
Alanine
Leucinea
Cysteine (cystine)
Asparagine
Lysine
Glutamine
Aspartic acid
Methionine
Histidine
Citrulline
Phenylalanine
Proline
Glutamic acid
Threonine
Taurine
Glycine
Tryptophan
Tyrosine
Serine
Valinea
As previously mentioned, many protein supplements differ in amino acid quantity and composition. Sources of protein are classified based on the adequacy of their essential amino acid profile as complete or incomplete proteins. The source of the protein is also critical as differences exist between animal and plant sources. Animal protein sources contain all essential amino acids and are considered complete, whereas plant proteins fail to contain all eight of the essential amino acids, leaving them as an incomplete protein resource. Evaluating the quality of the protein, or the quantity of essential amino acids present, is a vital criterion when selecting the proper protein supplement. The most common high-quality and complete proteins found in many protein supplements are whey, casein, soy, and egg (ovalbumin). Recently, vegetarian and vegan protein supplements have been introduced to the supplement market. Details concerning each protein type will be discussed in the following sections.
7.4.2.1 Whey Protein
Out of the four most well-known high-quality and complete proteins in the sports nutrition market, whey protein has become the industry and athlete favorite as it can be found in most muscle mass gainer and post-workout supplements. Derived from milk protein, whey protein comprises approximately 20 % of the total protein content in commercial bovine milk, which is significantly less than the 50–90 % (depending on stage of lactation) whey fraction of total protein found in human milk [8, 29–31]. The high fraction of whey protein in human milk is a key nutritional component to child growth and development. Whey protein is commercially categorized and available as whey protein concentrate (70–80 % PRO), whey protein isolate (90 % PRO), or whey protein hydrolysate (90 % PRO). Differences between these three forms of whey protein are exhibited by the processing technique. The various methods of processing affect the concentration of whey protein subtypes and peptides, which have been determined to help counteract various health-related issues beyond enhancement of muscle hypertrophy. Other differences among the forms of whey exist between fat and lactose content (remaining protein nutrient composition), their ability to preserve glutamine residues, and their amino acid profiles [8, 24]. Elliot et al. demonstrated that the greater fat composition within the protein profile may slightly facilitate a postexercise positive net protein balance and amino acid utilization [8, 32]. The most common form of whey protein used in sports nutrition supplements is whey protein concentrate (70 % and 80 % composition) due to its lower raw material pricing and postulated better flavor profile characteristics compared to the other whey forms [8, 33].
Whey tends to be digested quicker due to its sustained solubility and rapid emptying in the stomach, has better mixing characteristics, and is commonly considered a higher-quality protein compared other complete proteins [24, 34, 35]. The digestibility of whey contributes to its more rapid onset of amino acid appearance in the blood compared to casein, resulting in a greater stimulation of muscle protein synthesis [24, 36, 37]. The higher quality of whey protein can be partly attributed to its dominance in branched-chain amino acid composition, particularly leucine [38]. A study conducted by Tipton and colleagues demonstrated peaks in arterial leucine concentration occurred sooner and with greater magnitude following postexercise whey supplementation compared to casein [34]. Additional research has demonstrated that the essential amino acid composition of whey alone, compared to whey protein as a whole, resulted in greater muscle protein balance among the latter at rest [8, 39]. This finding suggests a possible synergistic effect within the protein composition that feeds muscular hypertrophy. Despite research finding that a higher leucine content is essential to muscle protein synthesis stimulation with resistance training in older adults [40], the addition of leucine to whole proteins, such as whey, after bouts of resistance exercise had little to no effect on protein synthesis and net nitrogen balance [8, 41, 42]. Similar to the frequent meal-based protein ingestion concept, consuming whey protein throughout the day is effective for increasing protein synthesis compared to less frequent or erratic intakes of other protein types [43]. In conjunction with resistance training, supplementation with whey protein is beneficial due to its rapid absorption, high branched-chain amino acid content, and health benefits when attempting lean muscle mass accretion.
7.4.2.2 Casein Protein
Casein, a second type of milk protein, is separated from whey protein within skim milk through resolubilizing and drying. Like whey, casein protein is found in commercial sports nutrition supplements in several variations, such as potassium caseinate, sodium caseinate, calcium caseinate, and casein hydrolysate [24]. Compared to whey, casein is a slower digesting protein. This fact is attributed to its coagulation within the stomach, which significantly reduces rate of elimination and increases transit time through the stomach [34, 44]. Tipton et al. compared the acute rate of arterial leucine appearance after post-resistance exercise protein ingestion of whey or casein [24, 34]. The results indicated that casein protein peaked later and to a lesser extent than whey protein, but the release of amino acids into the blood was sustained over a longer period of time with casein intake. A recent 8-week training study conducted by Wilborn and colleagues also assessed post-resistance exercise consumption of either whey or casein protein and demonstrated an increase in total protein synthesis, but no significant difference was exhibited between the two groups [34, 45]. Not all studies have found this response. A post-resistance training study conducted by Cribb et al. found the whey supplementation group had significantly greater gains in strength and lean body mass compared to the casein supplementation group after a 10-week training period [46]. Despite the inconclusive findings in the literature, casein has been shown to improve protein synthesis when combined with resistance training, but unlike whey, casein also has been found to have anti-catabolic properties [47]. Casein is also a moderately inexpensive form of protein, thus the reason why it is commonly found in many protein-based nutritional supplements. Due to the variation in casein formulation, a result of the specific resolubilizing processing technique affecting the amino acid profile, casein proteins are available in different grades based on protein quality (amino acid content), taste, and mixing characteristics [24, 28]. Perhaps the primary disadvantage to casein protein incorporation into a particular nutritional supplement is that it typically does not mix well in liquid, leaving clumps in suspension.
In summarizing the aforementioned protein research findings, whey was found to maximally stimulate protein synthesis, while casein was discovered to have anti-catabolic properties. Currently, most post-workout and lean mass gain supplements contain both proteins as part of their formulation. The rationale for this protein combination was confirmed by a 10-week resistance training study performed by Kerksick et al. in which three different supplement formulations were compared. The combination of both whey and casein protein supplementation groups proved to be the most effective as they demonstrated the most significant gains in lean muscle mass [48]. An interesting practical application of this study is to simply mix whey protein in bovine milk (which is ~80 % casein).
7.4.2.3 Soy Protein
Soy protein is the only plant-based protein source that is considered high quality due to its high concentration of all essential amino acids except methionine. Soy protein is made from legume and soybeans through a water- or water-ethanol-based extraction method [49]. Variations in this extraction method produce a soy protein concentrate or isolate. When compared to the consumption of milk proteins, soy protein was able to induce significant changes in muscle protein synthesis; however, greater net protein balance, fractional synthesis rate (of mixed muscle proteins), and protein synthesis in skeletal muscle were found with milk protein ingestion postexercise [38, 50, 51]. There are currently several studies that have attempted to explain the difference in muscle protein synthesis response between milk and soy proteins. In general, they have concluded that milk protein tends to support peripheral (e.g., skeletal muscle) protein synthesis, while soy protein tends to support greater splanchnic protein synthesis [38, 52, 53].
In addition to promoting muscle protein synthesis, soy protein sources, such as soy milk, contain the omega-3 fat alpha linolenic acid (ALA) and isoflavone phytoestrogens. Soy milk also has less saturated fat and cholesterol and tends to have a lower glycemic index than most bovine milk products [24]. Isoflavone phytoestrogens have been reported to stimulate estrogen receptors and promote nonhormonal estrogen-like properties that could benefit (pre- and post-) menopausal women [24, 54, 55]. More specifically, soy protein isolate contains a myriad of antioxidant compounds such as isoflavones, saponins, and copper, a primary component of many antioxidant enzymes [51]. Recent research has attributed soy isoflavones to improving markers of bone (i.e., protection against osteoporosis), lipid, and thyroid function while decreasing the risk of heart disease and promoting a chemo-protective effect against breast and prostate cancer [24, 56]. Despite the purported beneficial health effects of soy protein with no long-term side effects, there are several studies that question the long-term impact of diets containing high levels of phytoestrogens. Additional research needs to be conducted on several soy-related questions, such as whether soy protein ingestion effects hormone levels or semen quality in men, as it could potentially have a large impact on athletic performance [24].
The variable effects that soy protein supplementation can have on different genders leads to a difference in supplementation recommendation values. With the nonhormonal estrogen-like properties, soy protein can be an excellent source of high-quality protein for both pre- and postmenopausal female athletes. For male athletes, the lower muscle protein fractional synthesis rate of soy protein compared to whey may be less advantageous, but for female athletes, the lower saturated fat and cholesterol content may help to promote more nutrient-dense caloric intake when used as a supplement. Finally, due to the lower glycemic index of soy protein and its moderate rate of digestion between casein and whey, supplementation may be beneficial in isolated situations or in conjunction with the primary milk proteins.
7.4.2.4 Egg Protein
Egg protein has been a staple protein source in many nutritional supplements as it historically was considered the best source of protein due to its wide consumer acceptance as a high-quality protein. When processed for incorporation into a nutritional supplement, egg protein is extracted from chicken egg whites (ovalbumin) or whole eggs using a range of different techniques [24, 49]. Unlike casein protein, egg protein is highly miscible and able to form a homogeneous mixture when incorporated in liquids. Despite their decrease in popularity and regular incorporation into newer nutritional supplements due to its higher cost compared to other protein forms, egg white protein is labeled as the reference standard for comparing protein quality [24, 57]. Due to higher cost and poor taste of egg protein supplements, most athletes choose other sources of complete protein. Several studies compared post-resistance training effects of egg protein supplementation to casein and whey sources and concluded that there is no difference between the three forms of complete protein when assessing promotion of nitrogen retention. Due to its effectiveness and place as a reference standard, egg protein or ovalbumin is still incorporated in some meal replacement and protein power supplements on a minor scale [49].
7.4.2.5 Vegetarian and Vegan Protein Sources
Evidence from the Academy of Nutrition and Dietetics (AND) and other associated research confirms the nutritional needs of athletes can be met through consumption of a vegetarian or vegan diet. Despite this, doubt continues to linger among many nutrition professionals who counsel athletes. There are a growing number of athletes, especially endurance athletes, who have adopted a vegetarian- or vegan-based diet to help control body weight and meet increased carbohydrate needs [58, 59]. In comparison to omnivorous diets, vegetarian and vegan diets contain significantly higher quantities of complex carbohydrates, fiber, antioxidants, and phytochemicals. The high ratio of carbohydrates can help many athletes, particularly endurance or strength-endurance athletes, maintain adequate levels of glycogen storage in the muscle and liver as required fuel for muscular contraction [60]. Maintenance of adequate glycogen stores sustains a physiological state of higher energy availability, directly correlated to an environment primed for muscle mass development. Secondly, the inclusion of various antioxidants (e.g., vitamin C/ascorbic acid, vitamin E/tocopherol, and β-carotene) and phytochemicals from plant sources may positively affect athletic performance, recovery, and lean mass accretion by mitigating exercise-induced oxidative stress [59]. The potential to optimize glycogen stores from increased carbohydrate intake has been shown to have negative effects on the bioavailability of key nutrients such as iron, zinc, and other trace minerals among the general active population. The impaired nutrient bioavailability, particularly suboptimal iron levels, has been speculated in the vegetarian/vegan athletic population, but no connection to impaired muscle mass growth has been demonstrated [60, 61].
All essential and nonessential amino acids can be obtained from a diet strictly fueled from plant sources if enough calories are consumed to match the activity level of the individual and if a variety of sources are used to obtain those calories [59, 60, 62, 63]. Vegetarians (lacto-ovo-vegetarians) are more likely to meet the necessary protein macronutrient requirements due to their consumption of dairy products, eggs, and complementary mixtures of high-quality plant proteins (e.g., soy protein) resulting in approximately 12–14 % of their calories coming from protein [59]. Quality protein consumption can be a large concern for vegans (those who avoid consumption of all animal proteins) because plant-based proteins, making up only 10–12 % of caloric consumption, can be limiting in quantity of lysine, threonine, tryptophan, and/or sulfur-containing amino acids (methionine, cysteine, homocysteine, and taurine) as seen in Table 7.2 [59, 61]. Vegetarian athletes now have a wide variety of high-quality protein food and beverage sources (egg, dairy, and soy) containing all of the essential amino acids necessary to drive protein synthesis. However, vegan athletes may need to consider specific meal planning strategies that focus on plant sources rich in protein such as legumes, nuts and seeds, rice, and other whole-grain products in order to achieve proper nitrogen balance during periods of muscular growth [59, 62]. Additionally, well-planned vegan diets for competitive athletes may meet or exceed the RDA for protein in general, but may be deficient in lysine as most plant protein sources do not provide sufficient quantities [61]. As previously mentioned, recent protein and amino acid research demonstrates that leucine is the primary driving force behind the positive effects of branched-chain amino acids in the development of new muscle. In order to provide sufficient quantities of leucine in the diet of vegan athletes, a focus must be directed toward overall higher protein quantity of which a greater percentage should be provided by beans and legumes [61].
Table 7.2
Essential amino acid content comparison of dietary protein sources
Protein source | Lysine | Threonine | Tryptophan | Methionine/cysteinea |
---|---|---|---|---|
EAA (mg/g protein) | ||||
Beef | 83 | 44 | 11 | 37 |
Egg | 70 | 49 | 16 | 56 |
Wheat | 28 | 30 | 13 | 39 |
Brown rice | 38 | 37 | 13 | 35 |
Almonds | 29 | 32 | 15 | 25 |
Chickpeas | 67 | 37 | 10 | 28 |
Soybeans | 63 | 41 | 14 | 28 |
Compared to omnivores, vegetarians and vegans typically have a lower creatine pool, measured by significant decreases in total and free creatine concentration in addition to muscle creatine content [60, 61]. This decreased creatine pool demonstrates that the diminished dietary creatine intake from meat sources is not completely offset by increases in endogenous creatine production within vegetarian and vegan athletes [60, 64]. Creatine supplementation has been shown to have large ergogenic effects in active and athletic populations, but with diminished endogenous creatine stores, vegetarian and vegan athletes experience greater metabolic, performance, and body composition changes through increases in lean mass when supplementing with creatine [59]. Refer to Sect. 6 of this chapter for additional information concerning creatine supplementation.
7.4.3 Application
High-quality protein through whole foods and supplements, in adequate quantities addressing the intensity, volume, and duration of exercise, is the foundation for muscle hypertrophy. All four sources of high-quality protein, whey, casein, soy, and egg, are viable options for inducing lean muscle mass gains in strength-power athletes. While soy and egg proteins are less common protein sources found in today’s muscle-building supplements, they are great options for lactose-intolerant athletes. In order to maximize the benefits of protein ingestion on lean muscle gains, both quantity and quality have to be considered in addition to proper protein nutrient timing.
7.5 Dietary Considerations and Nutrient Timing
It has been well established that protein ingestion is necessary for muscle hypertrophy. To optimize muscle hypertrophy, carbohydrate-protein combinations and nutrient timing strategies can be used. In the absence of nutrient intake pre- and postexercise, the body will remain in a negative net protein balance state, therefore stimulating muscle catabolism [2, 4, 65]. This section will explain the importance of the carbohydrate-protein combination for muscle hypertrophy, nutrient timing optimization, as well as what happens when nutrition is neglected.
7.5.1 The Absence of Nutrition
As stated previously, the net protein balance determines whether muscle protein synthesis or muscle protein breakdown will occur. To achieve (net) muscle protein synthesis, a positive protein balance must occur in conjunction with a stimulus for muscle growth. Although resistance training stimulates muscle protein synthesis, that alone is not enough to supersede muscle protein breakdown [66].
To highlight this concept, a study was conducted evaluating rates of protein synthesis and breakdown at rest and 3 h following a weight training session among fasted individuals [2]. The individuals in the exercise group had a 108 % increase in protein synthesis and 51 % increase in muscle protein breakdown. Despite this increase in synthesis, it was not enough to result in an anabolic response. Similar studies have demonstrated the same results: increase muscle protein breakdown and synthesis rates but no net anabolic response [3, 4]. This research exemplifies the importance of nutrition for anabolic responses to occur.
7.5.2 Insulin, Amino Acids, and Protein Synthesis
As mentioned previously, weight training exercise provides a stimulus for muscle growth through muscle-specific genes. In conjunction with these genes being transcribed into the mRNA, other factors must be in place in order to convert this mRNA into skeletal muscle protein. Insulin and amino acids are fundamental compounds for protein synthesis. Bolster and colleagues [67] stated, “Without question, investigating the singular role of amino acids or insulin in promoting changes in skeletal muscle protein synthesis with resistance exercise is crucial to elucidating mechanisms regulating muscle hypertrophy.”
Insulin plays an important role in muscle protein synthesis, specifically following a resistance training bout [68, 69]. Following exercise, insulin is instrumental for amino acid incorporation into skeletal muscle [70–72] as well as minimizing the breakdown of muscle protein [71, 73–75]. Much of the early carbohydrate and protein research on the effects of insulin secretion demonstrated that the ingestion of carbohydrates surrounding an exercise session will increase plasma insulin levels, but an increase will also be seen in response to the infusion of free amino acids [76–78]. More recent literature has shown a significant insulin response to the ingestion of a carbohydrate, whole protein, and free amino acid (e.g., leucine and phenylalanine) mixture when compared to only carbohydrate at rest and postexercise in trained athletes [78–80]. As a safety disclaimer, insulin levels should be altered through diet, as insulin injections can lead to adverse effects. Simply consuming carbohydrates to elevate insulin levels will not result in an anabolic response, as amino acids must be present in the blood stream for muscle protein synthesis to occur [81]. This concept supports the importance of nutrient timing and combinations of protein and carbohydrate supplementation surrounding an exercise bout, which are discussed in detail below.
As stated previously, amino acids are the fundamental building blocks for muscle tissue. Thus, the availability of essential amino acids (EAAs) following a workout is a determining factor in creating a positive net protein balance for muscle protein synthesis. While some researchers have demonstrated that amino acid ingestion following a workout shifts net protein balance from negative to positive [9], consuming only amino acids may not provide the optimal nutrition for maximal gains in lean mass. Therefore, a combination of essential amino acids or high-quality protein and carbohydrates may be necessary to initiate maximal muscle protein synthesis following resistance exercise and is further investigated below.
7.5.3 Importance of Combined Carbohydrate-Protein Supplements
By consuming both carbohydrate and protein before and after a resistance training bout, muscle protein breakdown is attenuated and muscle protein synthesis is stimulated [65, 82, 83]. The complimentary roles these two macronutrients play on protein kinetics may help optimize muscle hypertrophy. The primary role of carbohydrate is to replenish glycogen stores, prevent muscle protein breakdown, and increase insulin levels [84–86]. One study provided subjects an amino acid-carbohydrate drink or a placebo after a resistance training bout and found there was an anabolic response at 1 and 3 h postexercise as measured by increased muscle protein synthesis and arterial phenylalanine and insulin concentrations among the treatment group [87].
Studies that have shown anywhere from 20 to 40 g of protein postexercise optimally stimulate muscle protein synthesis, the latter being more beneficial for older adults [86, 88, 89]. According to the International Society of Sports Nutrition, the recommended 0.15–0.25 g/kg/day addition of protein during all instances of carbohydrate intake helps to maximize muscle protein synthesis and glycogen restoration [12]. The addition of a carbohydrate source when supplementing with protein post-workout may be particularly necessary following sessions highlighting goals associated with endurance or muscular strength-endurance training where expedited glycogen restoration is critical. The most success with carbohydrate-protein combination supplementation in terms of maximizing muscle protein synthesis is the addition of carbohydrates to a source of EAAs [87]. However, more recent nutrient timing research with respect to carbohydrate dosage in the early recovery period following a bout of resistance training has found that the addition of carbohydrates post-workout may not be necessary to further increase whole-body protein balance when proper protein supplementation is provided to strength or hypertrophy athletes [90]. These latest findings highlight that the minimum requirements for sustaining gains in muscle mass as a result of resistance training are to supplement with high-quality protein pre- and postexercise along with ensuring that overall daily macronutrient needs are addressed throughout the course of a training day, particularly carbohydrates. Specific training goals, necessary energy systems for performance, and recovery timelines should be considered when determining the proper supplementation combination of carbohydrates and protein to optimize lean mass accrual.
7.5.4 Nutrient Timing
The central premise of nutrient timing is to time the ingestion of protein and carbohydrate to facilitate the greatest muscle protein synthesis. Despite muscle’s ability to ingest protein during the 24 h following a resistance training session [91], the ability to maximize muscle protein synthesis and minimize muscle protein breakdown may rely on the timing of nutrition. As science continues to evolve, The International Society of Sports Nutrition acknowledges the difficulty in specific recommendations, as the research regarding nutrient timing is inconsistent and varies between individuals [84]. This section explores the current literature relating to nutrient timing.
7.5.4.1 Before a Workout
Research suggests that ingesting protein before and after exercise can increase muscle protein synthesis [32, 92–96]. One study compared carbohydrate-amino acid (EAA) consumption before versus after a workout and found that consuming before a workout resulted in greater muscle protein synthesis among the pre-workout group [97]. Tipton and colleagues were interested in comparing net protein balance when ingesting whey protein before or after a workout. They discovered that amino acid availability increased equally pre- and post-workout, unlike a previous study that found a significant increase in muscle protein synthesis with pre-workout carbohydrate-EAA supplementation [98]. More research is needed in this area; however, the current evidence reflects positive outcomes from pre-workout nutrition.
7.5.4.2 After a Workout
The anabolic window, the immediate time period after a resistance training bout, has been thought to be a critical period for preventing protein breakdown, optimizing protein synthesis and muscle hypertrophy, and replenishing glycogen stores via proper nutrition. This section aims to explore the validity of these claims.
By consuming a meal after a workout, muscle protein breakdown is slowed down due to the rise in insulin levels [6, 84, 99]. The combination of both carbohydrate and protein has the potential to elevate insulin greater than just carbohydrates [84, 100], but as mentioned previously, strength and hypertrophy athletes with goals to maximize muscle gain should shift their carbohydrate focus away from a specific post-workout timeline and more toward meeting an overall daily macronutrient need for carbohydrates [90]. However, although theoretically sound, this idea has yet to be demonstrated in practice. Research has demonstrated that the influence of elevated insulin on net protein balance reaches a plateau between 15 and 30 mU/L, approximately three to four times the normal levels [101, 102]. A study conducted by Power and colleagues [103] demonstrated lasting peak insulin concentration, needed for muscle protein balance, for up to 2 h after consuming 45 g of whey protein isolate. Therefore, consuming food immediately postexercise might not be as critical as once thought, unless a pre-workout meal is absent.
Previously, for maximal muscle protein synthesis, it was crucial to include free amino acids (e.g., EAAs or BCAAs) and/or whole protein sources immediately post-workout [9, 82, 84]. To further emphasize the importance of post-workout nutrition, a study demonstrated the effects of consuming 10 g of protein, 8 g of carbohydrate, and 3 g of fat immediately after exercise compared to consumption of the same macronutrient composition 3 h after exercise. There was a 12 % increase in protein synthesis for the group that consumed the meal immediately following the workout [104]. However, studies have also demonstrated there are no timing effects with respect to the anabolic window. Rasmussen and colleagues [87] provided 6 g of essential amino acids with 35 g of carbohydrates to two groups: 1 h vs. 3 h postexercise. The results of the study concluded that there was no significant difference between net leg amino acid concentrations. Similarly, a study conducted by Tipton and colleagues [97] demonstrated that consumption of an essential amino acid-carbohydrate supplement pre-exercise demonstrated greater muscle protein synthesis when compared to postexercise consumption of the same supplement, although there have been disputes on the validity of this study design [99].
Tipton also looked at the physiological response when consuming whey versus casein protein following a weight training session and found that both types of protein led to increases in muscle protein balance and synthesis [34]. The main difference found between the two types of protein was the amino acid response in the blood: a sustained response was elicited by casein, whereas a quick, initial response was elicited by whey. When considering the implications of supplementing whey versus casein protein post-workout, ideally both types of protein should be provided together to optimize muscle protein balance over the course of the extended recovery period to help maximize muscle recovery, synthesis, and regeneration. However, the training program and goals in addition to the training experience should be considered when choosing the optimal type of protein supplementation.
There is conflicting evidence in the literature regarding the effects of nutrient timing post-workout on muscle hypertrophy. A number of studies have been conducted: some demonstrating muscular hypertrophy, whereas others have shown no changes. For a more detailed description on the studies discussed above, please reference Table 7.3 and/or the following text by Alan Argon and Brad Schoenfeld: Nutrient Timing revisited: is there a post-exercise anabolic window?
Table 7.3
Current literature: effects of postexercise supplementation on muscle hypertrophy
Study | Subjects | Supplementation | Matched control group? | Instrument of measurement | Training protocol | Results |
---|---|---|---|---|---|---|
Esmark et al. | 13 untrained elderly males | 10 g milk/soy protein combo consumed either immediately or 2 h postexercise | Yes | MRI and muscle biopsy | Progressive resistance training consisting of multiple sets of lat pulldown, leg press, and knee extension performed 3 days/week for 12 weeks | ↑↑ muscle CSA w/immediate vs. delayed supplementation |
Cribb and Hayes | 23 young recreational male bodybuilders | 1 g/kg of a supplement containing 40 g whey isolate, 43 g glucose, and 7 g creatine monohydrate consumed either immediately pre- and postexercise or in the early morning and late evening | Yes | DEXA and muscle biopsy | Progressive resistance training consisting of exercise for the major muscle groups performed 3 days/week for 10 weeks | ↑↑ lean body mass ↑↑ muscle CSA of type II fibers both w/ immediate vs. delayed supplementation |
Willoughby et al. | 19 untrained young males | 20 g protein or 20 g dextrose consumed 1 h pre- and postexercise | No | Hydrostatic weighing, muscle biopsy, surface measurements | Progressive resistance training consisting of 3 sets of 6–8 reps for all the major muscle groups performed 4 days/week for 10 weeks | ↑↑ total body mass ↑↑ fat-free mass ↑↑ thigh mass all w/protein vs. carb supplementation |
Hulmi et al. | 31 untrained young males | 15 g whey isolate or placebo consumed immediately pre- and postexercise | No | MRI and muscle biopsy | Progressive, periodized total body resistance training program consisting of 2–5 sets of 5–20 reps performed 2 days/week for 21 weeks | ↑↑ vastus lateralis CSA ↔ other 3 quad muscles CSA both w/ protein vs. placebo |
Verdijk et al. | 28 untrained elderly males | 10 g casein hydrolysate or placebo consumed immediately pre- and postexercise | No | DEXA, CT, and muscle biopsy | Progressive resistance training consisting of multiple sets of lat pulldown, leg press, and knee extension performed 3 days/week for 12 weeks | ↔ muscle CSA no significant difference between groups |
Hoffman et al. | 33 well-trained young males | Supplement containing 42 g protein (milk/collagen blend) and 2 g carbohydrate consumed either immediately pre- and postexercise or in the early morning and late evening | Yes | DEXA | Progressive resistance training program consisting of 3–4 sets of 6–10 reps of multiple exercises for the entire body performed 4 days/week for 10 weeks | ↔ total body mass ↔lean body mass no significant differences between groups |
Erskine et al. | 33 untrained young males | 20 g high-quality protein or placebo consumed immediately pre- and postexercise | No | MRI | 4–6 sets of elbow flexion performed 3 days/week for 12 weeks | ↔ muscle CSA no significant difference between groups |
7.5.5 Application
The value of nutrient timing plays an important role when addressing muscle protein synthesis. Despite numerous studies in this area of research, there is no exact science to maximizing the effects of nutrient timing; rather, an individual approach may work best to promote optimal muscle hypertrophy. To optimize overall muscle mass gains, high-quality protein supplementation pre- and postexercise is critical along with ensuring that overall daily macronutrient needs are addressed throughout the course of a training day, particularly carbohydrates. Therefore, a general recommendation for nutrient timing around a workout is 0.4–0.5 g/kg of quality protein per lean body mass at both pre- and post-workout [27, 89, 105]. Ideally, no more than 3–4 h should pass between pre- and post-workout meals, unless a larger meal is consumed, in which 5–6 h is okay [84]. Realistically, nutrient timing and training timing will depend on the individual. In respect to carbohydrates around a workout, the verdict is still unclear as to if carbohydrates post-workout is necessary for optimizing muscle hypertrophy; rather the individual should emphasize daily carbohydrate needs [84].
7.6 Creatine
Creatine is one of the most extensively researched and popular nutrition supplements. Over the last two decades, creatine has been examined in over 500 research studies that have assessed its viability on muscle physiology, body composition, and/or exercise performance in trained, healthy, and various diseased populations. The vast quantity of research conducted supports the claim that creatine supplementation during training, particularly high-intensity exercise, results in promotion of significant strength, fat-free mass, and performance improvements. The majority of scientific evidence available in the literature demonstrates that creatine monohydrate supplementation in athletic, active, and clinical populations is a generally effective nutritional ergogenic aid when paired with various forms of exercise [106]. The laundry list of proven benefits as a result of creatine supplementation coupled with solidified safety, when the recommended dosages are consumed, cements the reason why creatine is considered the gold standard of nutritional supplements.
7.6.1 Supplementation Protocols
Most of the research investigating the effects of creatine supplementation has utilized creatine monohydrate and employed a dosage pattern involving an initial loading phase followed by a maintenance phase. Incorporation of this loading phase, typically 0.3 g/kg/day (15–25 g/day) ingestion of creatine monohydrate for 5–7 days, is the most common method for increasing muscle creatine stores [24, 107]. Loading has also been described as consuming 5 g creatine monohydrate four times per day (20 g total) over the course of a 5–7-day period, an approach that increases muscle creatine and phosphocreatine levels by 10–40 % [24, 107, 108]. Recent studies demonstrate that the loading period may only need to last 2–3 days if taken in combination with carbohydrate and/or protein to maximize creatine stores [24, 109–111]. Many post-workout recovery supplements on the market utilize a combination of creatine monohydrate, protein (whey, soy, and/or casein), and carbohydrate to help maximize muscle growth and development. Beyond the 2–7-day creatine loading/muscle creatine saturation period, the maintenance phase consists of a 3–5 g daily dose (or 0.03 g/kg) of creatine monohydrate for several weeks to a few months, matching the periodization pattern of the training program [107]. One of the alternative creatine loading methods, if the immediate maximization of creatine stores is not an important consideration, is to ingest 3 g/day of creatine monohydrate for at least 28 days resulting in a linear muscle creatine increase. This progressive method has been proven to achieve similar intramuscular creatine and phosphocreatine levels as the aforementioned loading methods [24, 112].
Rather than basing the quantity of creatine supplementation on total body mass or a specific quantity, Burke et al. [113] found successful promotion of muscle growth and performance by basing creatine dosage on the subjects’ lean body mass, more specifically, ingesting creatine monohydrate based on a dose of 0.1 g/kg of lean body mass per day. Other training studies observing the effects of creatine supplementation on performance and changes in lean mass determined that both a high-dose protocol (15–25 g/day or 0.3 g/kg/day, equivalent to a loading phase protocol) and a low-dose protocol (3–6 g/day) during training increased intramuscular creatine levels [24]. The dosing protocol should match the goals of the training program, the anaerobic nature of the sport or event, and/or the anabolic needs for lean mass development. Alternatively, during training, a cyclic loading protocol for creatine may be the most appropriate for athletes as it takes 4–6 weeks for elevated intramuscular creatine stores to return to baseline. This cyclic loading involves supplementation of 0.3 g/kg/day (15–25 g/day) creatine monohydrate for 3–4 weeks and then cycling off for 4–6 weeks to most effectively escalate and maintain elevated creatine stores over time [24, 114].
7.6.2 Effects on Lean Body Mass
In addition to gains in performance, particularly strength, power, and anaerobic capacity, increases in body weight in the form of lean mass have been demonstrated in numerous studies when subjects supplement with creatine. During a typical creatine loading period (first 5–7 days), the literature indicates that individuals will experience approximately 0.6–2.0 kg gains in lean body mass [24, 106, 109, 115, 116]. Research suggests that short-term creatine supplementation, perhaps during a single training cycle, has proven to increase total body mass by approximately 0.7–1.6 kg (~1.5–3.5 lb.) [117]. Specifically, a 28-day creatine supplementation (20 g/day) and resistance training study conducted by Earnest et al. [116] found a 1.7 kg increase in total body mass of which 1.5 kg was fat-free mass [115]. Creatine supplementation over the course of a long-term resistance training program (approximately 6–8 weeks) has revealed 2.8–3.2 kg (~7 lb.) increases in lean body mass [116, 118, 119]. Additional research indicates that 4–8 weeks of resistance training plus supplementation with creatine in combination with glucose, taurine, and electrolytes may promote greater gains in lean mass (~1.03–3.83 kg) when compared to creatine supplementation alone [115, 120]. Similar gains in lean muscle mass with resistance training and creatine supplementation have been reported in women. A 10-week study conducted with females assessed resistance training and creatine supplementation [121]. The females who ingested creatine based on the aforementioned loading and maintenance protocol (20 g/day for 4 days followed by 5 g/day for 65 days) reported a 2.6 kg (~5.7 lb.) increase in lean mass [121]. An increase in lean mass (60 % greater than the placebo in the previous study) is a typical ergogenic benefit seen by women with creatine supplementation; however, gains in overall body mass and lean mass are generally slower than men [24]. The acute (initial 5–7 days) increase in lean mass following creatine monohydrate supplementation coupled with a resistance training program was found to be lower in women (500–700 g) compared to men (1.0–1.5 kg) in a study conducted by Mihic et al. [122].
7.6.3 Physiological Mechanisms for Increasing Lean Body Mass
Some research has addressed the potential physiological mechanisms responsible for the promotion of lean body mass as result of creatine supplementation, but no definitive conclusions have been agreed upon. The initial studies investigated creatine supplementation and associated gains in lean mass, concluding that the primary reason for increased body mass was due to additional water retention in the muscle. Recent research suggests that creatine supplementation increases body mass through greater myofibrillar protein synthesis and overall lean mass accretion [115, 123]. A 12-week resistance training study by Volek et al. [124] demonstrated that resistance-trained males ingesting a creatine supplement accrued significantly greater lean mass compared with those ingesting a placebo. More specifically, significant differences in fiber cross-sectional area were observed in all three muscle fiber types between the creatine supplementation and placebo groups: type I (35 % vs. 11 %), type IIA (36 % vs. 15 %), and type IIX (35 % vs. 6 %). The muscle fiber cross-sectional area growth in the creatine group, for all muscle fiber types, was more than twice the increases observed in the placebo group, with the largest difference found in the most anaerobic fiber type (type IIX) [117, 124].
The physiological mechanisms connecting increases in lean body mass as a result of creatine supplementation were further analyzed by Willoughby and Rosene in a series of two studies examining the effects of oral creatine supplementation on contractile filament gene expression and myosin heavy-chain protein expression [125, 126]. The first of the two studies recruited and divided untrained male subjects into two supplement groups: creatine (6 g/day) or placebo. Both groups participated in a 12-week resistance training program. Similar to previously mentioned studies, the creatine supplementation group had significantly greater gains in lean mass compared to the placebo (~7 lb vs. ~1 lb). Despite similar results in lean mass, this initial study also noted some interesting differences between groups at the cellular level of skeletal muscle. The marker for quantity of intracellular protein content, or myofibrillar protein content, demonstrated significantly greater elevations in the creatine supplementation group compared to the placebo when both groups participated in the same resistance training program. This difference between supplementation groups was associated with the significant increases found in the two isoforms of myosin heavy-chain protein (fundamental units of contractile skeletal muscle) in the creatine group compared to the placebo [125].
The second study conducted by Willoughby and Rosene expanded upon the results of their first study by analyzing the effects of creatine supplementation in conjunction with a similar 12-week resistance training program on changes in myogenic regulatory factor gene expression. Myogenic regulatory factors (e.g., Myo-D, myogenin, MRF-4, andMyf-5) are vital proteins that drive the regulation of gene expression through their function as transcription activators. These myogenic regulatory factors work to regulate gene expression by binding to DNA and subsequently activating muscle-specific gene transcription for important muscular components such as myosin light chain, myosin heavy chain, troponin I, α-actinin, and creatine kinase [127]. At the conclusion of the 12-week resistance training program, subjects supplementing with creatine demonstrated significantly greater myogenin and MRF-4 mRNA expression compared with subjects ingesting the control during the resistance training program [126]. These studies conducted by Willoughby and Rosene demonstrate greater mechanistic insight into the development of lean body mass when supplementing with creatine in conjunction with a resistance training program. Furthermore, these studies indicate that increases in lean body mass from creatine supplementation are not solely attributed to greater water retention in the muscle, but rather demonstrated that creatine supplementation may induce skeletal muscle hypertrophy at the level of gene expression within the muscle environment.
7.6.4 Satellite Cell Activity
In conjunction with all of the aforementioned benefits of creatine supplementation for increasing lean body mass, the literature also demonstrates that creatine ingestion may enhance the increase in skeletal muscle satellite cell numbers, normally attributed to strength training alone. The activation of muscle-specific satellite cells is speculated to be the primary factor associated with amplifying skeletal muscle hypertrophy beyond muscle-specific transcription and translation. Load-induced muscle hypertrophy, as a result of strength training, is a stimulus for a growth process defined by satellite cell proliferation, differentiation, and fusion with existing myofibers or muscle cells [128]. The myonuclear domain theory defines the involvement of satellite cells in the process of skeletal muscle hypertrophy, suggesting that the myonucleus defines the production of mRNA and proteins through transcription and translation for a specific volume of cytoplasm. Hypertrophy of the muscle through increases in fiber size must include a proportional increase in myonuclei, which are typically contributed to the myofibers via fusion of satellite cell populations [129]. If satellite cells are the primary contributor of myonuclei in a growing muscle, then the myonuclear domain theory, if correct, indirectly states that anything promoting increases in satellite cell activity will ultimately lay the foundation for greater changes in skeletal muscle hypertrophy.
The influence of creatine supplementation on satellite cell activity, based on the myonuclear domain theory, was explored by Olsen et al. [130]. These researchers studied changes in satellite cell frequency and the number of myonuclei in human skeletal muscle over a 16-week resistance training program in conjunction with creatine supplementation, protein ingestion, or a placebo cohort. At the end of the 16-week resistance training regimen, all groups demonstrated an increase in satellite cell proportion. Despite increases in satellite cell proliferation across the board, creatine was the only supplement that demonstrated significantly different increases in the myonuclear density of the muscle fiber. As a result, Olsen et al. determined that resistance training in conjunction with creatine supplementation intensifies the strength training-induced increase in both satellite cell number and concentration of myonuclei in human skeletal muscle. Skeletal muscle fiber hypertrophy is amplified when creatine supplementation is combined with the proper resistance training program [130].
7.6.5 Application
Regarded as one of the most highly researched nutritional ergogenic aids from clinical to athletic applications, creatine has proven to be the most effective and safest supplement to increase lean body mass, strength, and performance. Concerns about the safety and potential side effects of creatine supplementation have been put to rest by long-term safety and pharmacokinetic studies that revealed no apparent side effects with supplementation. As a consumer of supplements containing creatine or creatine supplements, it is important to note the type, quality, and/or source of the creatine, as not all forms are equal. Despite numerous attempts by various nutritional companies to develop the latest and most effective form of creatine, creatine monohydrate remains as the gold standard for supplementation. Additionally, it is highly recommended that creatine supplementation be closely aligned with the periodization pattern and goals of the athlete’s training program in order to maximize performance and lean body mass gains.
7.7 Anabolic Hormone Enhancers
Serving as key messengers in the body, hormones play an essential role to building and maintaining muscles. Food, stress, and activity all heavily influence the concentration of hormone levels in the body. Although there are hundreds of hormones in the body, the ones discussed in this chapter are specifically related to muscle building. The first set of hormones reviewed is anabolic hormones, better known as the hormones that directly stimulate muscle growth. The next set of hormones is concerned with preventing catabolism of muscle (Sect. 9). Understanding the role of hormones in the muscular growth process is important for optimizing body composition as well as realizing the positive and negative effects of supplementation. The key anabolic hormones found in the body are testosterone, human growth hormone, insulin, and insulin-like growth factor 1 (IGF-1).
7.7.1 Testosterone
Testosterone is a lipid-soluble hormone that is produced in both males and females. Its role in muscle hypertrophy is to promote protein synthesis, prevent catabolism [131], and promote the release of growth hormone. Testosterone and its synthetic derivatives, known as anabolic-androgenic steroids (AAS), have been used in medicine and recreation to induce muscle hypertrophy and/or reduce muscle atrophy due to disuse and aging. It is a banned substance by the NCAA, the International Olympic Committee, and by all major North American Professional Leagues [132]. It is currently legal to administer testosterone and AAS in a medical setting, but it is also used in sport and competition, despite being illegal.
Many supplements claim to increase testosterone; however it is thought that only bioavailable or free testosterone promotes an anabolic response in muscle tissue. When assessing supplement claims for increasing testosterone levels, one should note changes in total testosterone, free testosterone, estradiol, as well as sex hormone-binding globulin. Of these hormones, only free testosterone can bind to androgen receptors and promote anabolism. A few supplements that claim to increase testosterone are ZMA, tribulus terrestris, and aromatase inhibitors. ZMA and tribulus terrestris are discussed in the sections below, while aromatase inhibitors are further referenced in Sect. 8.
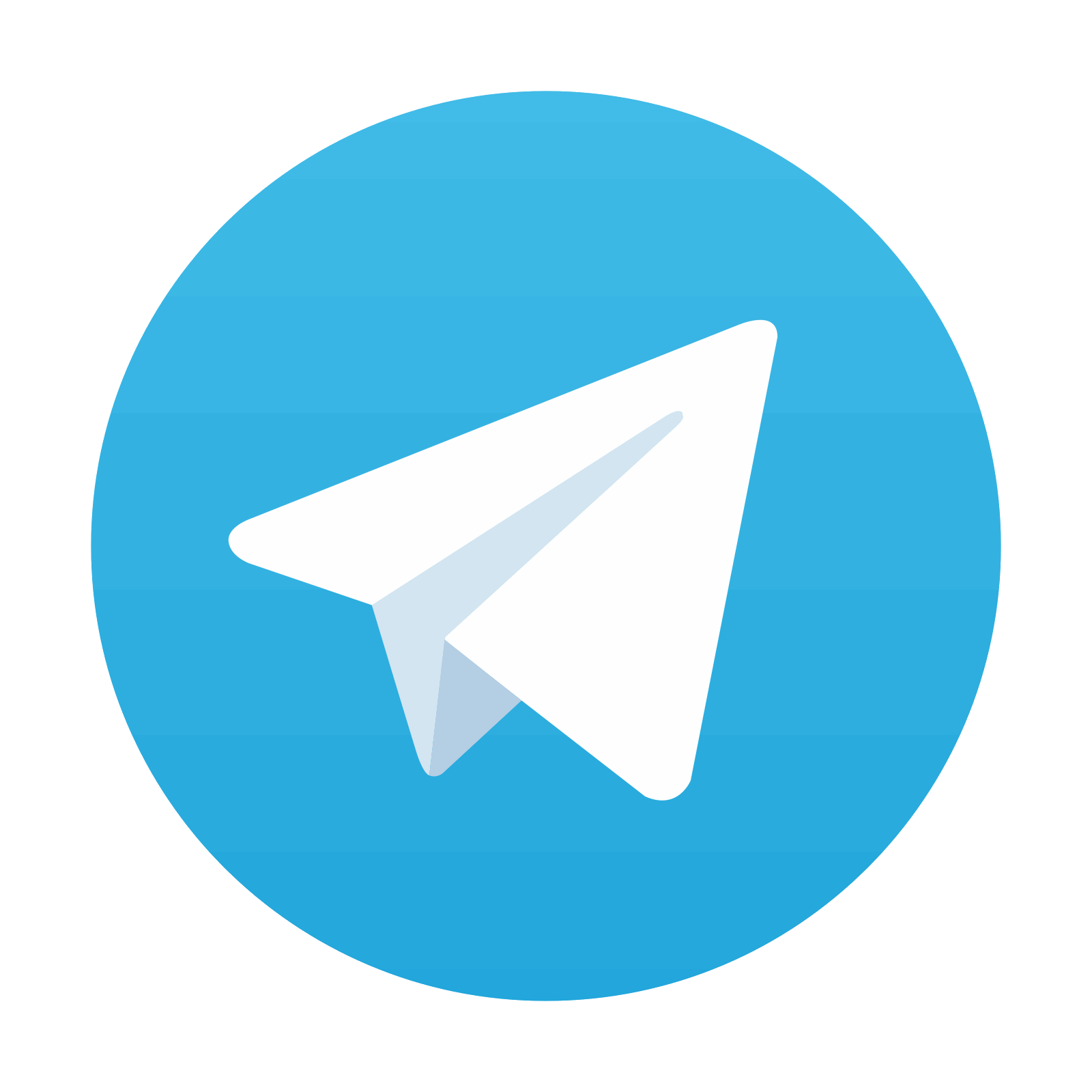
Stay updated, free articles. Join our Telegram channel

Full access? Get Clinical Tree
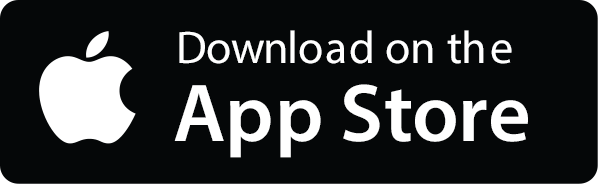
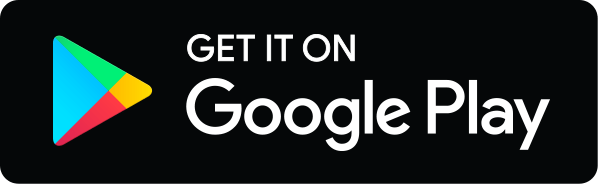