CHAPTER 11
Botulinum Toxin in the Treatment of Lower Limb Spasticity
Alberto Esquenazi
Approximately 700,000 people are affected by a stroke each year in the United States, and there are more than 1,100,000 Americans surviving with residual functional impairment after stroke (1,2). Traumatic brain injury (TBI) is another form of acquired brain injury and continues to be an enormous public health problem in the 21st century even with modern medicine. Most patients with TBI (75%–80%) have mild head injuries; the remaining injuries are divided equally between moderate and severe categories. The cost to society of TBI is staggering, both from an economic and an emotional standpoint. Almost 100% of persons with severe head injury and as many as two thirds of those with moderate head injury will be permanently disabled in some fashion and will not return to their premorbid level of function. In the United States, the direct cost of care for patients with TBI, excluding inpatient care, is estimated at more than $25 billion annually. The impact is even greater when one considers that most severe head injuries occur in adolescents and young adults with long survival rates.
Acquired brain injury affects a person’s cognitive, language, perceptual, sensory, and motor functions (3). Recovery is a long process that continues beyond the hospital stay and into the home setting. The rehabilitation process is guided by clinical assessment of motor abilities. Accurate assessment of the motor abilities is important in selecting the different treatment interventions available to a patient.
Spasticity is a term that is often used by clinicians, and although used frequently, it can have different meanings in its interpretation and presentation. Spasticity is just one of the many positive signs of the upper motor neuron syndrome (UMNS), yet, under the heading of “spasticity,” clinicians often group all positive signs together and sometimes include negative signs as well. Many of these frequently misidentified phenomena fall under the broader heading of the UMNS—a condition that has classically been partitioned into a syndrome of positive and negative signs, including weakness, loss of dexterity, increased phasic and tonic stretch reflexes, clonus, cocontraction, released flexor reflexes, spastic dystonia, and associated reactions or synkinesias.
The issue of terminology is more than semantics and of great clinical importance because, for example, treatment of cocontraction, a phenomenon likely to be of supraspinal origin, will differ from treatment of clonus, a phenomenon of the segmental stretch reflex loop. If clinicians desire a concise, descriptive, utilitarian term that captures the essence of positive UMN phenomena, “muscle overactivity” may be a more suitable term than “spasticity,” especially because the phrase “muscle overactivity” evokes an image of dynamic muscle contraction, the general hallmark of all positive signs of UMNS (4).
Spasticity has classically meant increased excitability of skeletal muscle stretch reflexes, both phasic and tonic, that are typically present in most patients with a UMN lesion. After a UMN lesion, a net loss of inhibition impairs direct descending control over motor neurons. There is also a loss of inhibitory control over interneuronal pathways of the cord that ordinarily regulate segmental spinal reflexes, including stretch reflexes, especially those concerned with antigravity muscles.
Lance characterized spasticity as an increase in velocity-dependent tonic stretch reflexes with exaggerated tendon jerks (5). In Lance’s consensus definition, tonic stretch reflexes referred to the output response of a muscle group that was stretched at different velocities. “Exaggerated tendon jerks” were examples of “phasic” stretch reflexes. In routine practice at the bedside, the two ways of assessing phasic and tonic stretch reflexes are tendon taps and passive stretch of a muscle group at different velocities (6,7).
Although positive signs are a common source of clinical concern and are frequently treated, negative signs may be at times more functionally disabling and difficult to address. Negative signs signify loss or impairment of voluntary movement assembly and production, a kind of “muscle underactivity” that, in effect, can be described as phenomena of absence (5,8).
The clinical picture is made more complex by another phenomenon that has not been classically positioned among the positive signs, namely, contracture or what is better described as the physical changes in the rheologic properties of muscle tissue. Contracture is well recognized by rehabilitation clinicians as a major source of disability for patients with UMNS. Ironically, phenomena of absence and phenomena of presence can both provide a context for the development of contracture (9).
FUNCTIONAL IMPLICATIONS OF SPASTICITY
Fifty years ago, Nikolai A. Bernstein suggested that the basic problem of motor control relates to overcoming redundant degrees of freedom in our multijointed skeletal system, the multijointed limb segments that allow us to interact with the three-dimensional (3-D) world we live in. Commonly, there are multiple “agonists” and “antagonists” for virtually any movement direction. To match a required joint torque even across a single joint, the question regarding which muscles should be activated and at what levels of activity is likely to have a very variable answer without a unique solution. For a given patient, however, there may be a “unique” solution in that equinovarus deformity may be solely attributable to an overactive tibialis anterior in one patient, whereas in another, it may be an overactive tibialis posterior (9).
Patterns of limb dysfunction in the UMNS have an impact on the limb utilization for gait or other functional use. A number of muscles typically cross major joints of the extremities, and identifying the actual muscles that contribute dynamically and statically to a UMNS deformity is an important key to clinical management of the resulting gait or upper limb dysfunctions (10,11). Clinical evaluation is useful to the analysis of movement dysfunction, but gait and motor control assessment laboratory evaluation using dynamic electromyography (EMG) and other assessment techniques is often necessary to identify the particular contributions of offending muscles with confidence. The correct selection of target muscles that contribute to any one pattern of dysfunction may serve as a rational basis for interventions that focus on specific muscles, including chemodenervation with botulinum toxin (BoNT); neurolysis with phenol; and surgical lengthening, transfers, and releases of individual muscles.
This concept, namely, identifying which muscles contribute dynamically and statically to upper motor neuron dysfunction, serves as a conceptual basis for this text. Simply put, identifying muscles that produce deforming maladaptive joint movements and postures statically and dynamically is an important endeavor in aiding clinical interpretation of gait dysfunction and in rationalizing subsequent treatment interventions (12,13).
Dynamic EMG, gait, motion analysis, and diagnostic nerve blocks frequently provide the necessary detailed information about specific muscle groups that will guide decision making for treatment. Before selecting treatment interventions, the clinical team and the patient should explicitly develop functional goals. Functional goals may be classified as symptomatic, passive, or active in nature (9). A symptomatic goal refers to the intent to address clonus, flexor, or extensor spasms, and pain, among others, as some of the targeted goals. Active functions refer to a patient’s direct use of the limb to carry out a functional activity. Passive function has a different context and refers to the passive manipulation of limbs to achieve functional ends, typically through patients’ passive manipulation of the affected limb with the noninvolved limbs or having their caregivers perform the manipulation. Identifying muscles with volitional capacity is important to the achievement of this goal. In broad terms, clinical evaluation focuses on the identification of several factors: Is there selective voluntary control of a given muscle? Is the muscle activated dyssynergically (ie, as an antagonist in movement)? Is the muscle resistive to passive stretch? Does the muscle have fixed shortening (contracture)? In the Gait and Motion Analysis Laboratories, dynamic EMG is acquired and examined in reference to simultaneous measurements of joint motion (kinematics) and ground reaction forces (kinetics) obtained from force platforms. Kinetic, kinematic, and dynamic EMG data augment the clinician’s ability to interpret whether voluntary function is present in a given muscle and whether that muscle’s behavior is also dyssynergic (Figure 11.1). Combined with clinical information, the laboratory measurements of muscle function often provide the degree of detail and confidence necessary to select, aim, and optimize the rehabilitation interventions. In addition, evaluation under the effect of temporary diagnostic nerve or motor point blocks can help the clinician distinguish between obligatory and compensatory limb postures and gait patterns (14).
FIGURE 11.1 Subject instrumented for gait analysis data collection including dynamic EMG and CODA 3-D motion sensors.
EMG, electromyography; 3-D, three-dimensional.
CLINICAL ASSESSMENT OF SPASTICITY
There are many assessment techniques used in routine clinical examination of the patient with spasticity. Motor control, passive range of motion, manual muscle strength, Ashworth, and Tardeau are examples of such techniques that are frequently used.
For more information, the reader is encouraged to review Chapter 7 of this text. Passive range of motion can be used to determine the available movement for each joint but does not provide information on the cause of limitations if present. Spasticity, muscle overactivity, contracture, or pain can all play a role in limited joint passive range of motion.
Manual muscle testing allows grading of available strength if normal control is present; the grading is done using a 6-point scale, where 5 is a normal rating with ability to resist significant force and 0 is unable to move. In the UMNS, testing of strength may be affected by impaired motor control, the presence of synergistic patterns, and cognitive deficits.
The Ashworth Scale allows assessment of muscle tone; in the Modified Ashworth, the rating uses a 5-point scale. The scale has only been validated for the elbow and requires the movement of the joint through its available range in 1 second. Ideally, the test should always be done in the same position and under similar conditions (15). One disadvantage is that this test does not take into consideration the presence of contracture or other factors that may limit joint motion.
The Tardeau Test was developed in the pediatric population in the mid-1960s. It attempts to assess spasticity by varying the speed of joint motion available from very slow (V1) to as fast as possible (V3). The difference between the parameters permits an estimation of the effect of spasticity (16) (Figure 11.2).
Unfortunately, none of these assessments provides a functional perspective, such as during walking, and cannot precisely determine the source of the problem. Based on our clinical experience, methods based on a functional perspective such as those described in the following can be more helpful in this regard.
The Impact of Gait
Gait is a functional task performed by most humans. The three main functional goals of ambulation are to move from one place to another, to move safely, and to move efficiently. These three goals are frequently compromised in the patient with residual UMNS. Most patients will be able to perform limited ambulation, but they will often have problems because of inefficient movement strategies, the presence of instability or pain due to abnormal limb postures, and decreased safety. Some generalizations can be made about the gait of patients with acquired brain injury. These include a decrease in walking velocity with a reduction in the duration of stance phase and impairment of weight bearing in the affected limb with an increase in the duration of stance time of the less affected limb (17). Ochi et al (18) reported on differences in temporo-spatial parameters of locomotion among patients with residual stroke and TBI. From a functional perspective, gait deficiencies can be categorized with respect to the gait cycle. In the stance phase, an abnormal base of support can be caused by equinovarus, toe flexion, or ankle valgus. Limb instability can occur due to knee buckling (sudden flexion) or hyperextension, which may result in knee joint pain or lack of trunk control. This may result in unsafe, inefficient, or painful walking.
FIGURE 11.2 Demonstrating the Tardeau measurement using superimposed images of very slow and very fast PROM. A difference of approximately 20° can be seen between the two measures and indicative of the degree of spasticity.
PROM, passive range of motion.
During the swing phase, inadequate limb clearance caused, for example, by a stiff knee and inadequate limb advancement caused by limited hip flexion or knee extension may interfere with the safety and energy efficiency of walking. To identify the potential source of the problem and to focus more appropriately on the essence of multifactorial gait dysfunction, formal gait analysis in a laboratory may be required. Combining clinical evaluation with laboratory measurements will increase the degree of resolution needed to understand the common patterns of gait dysfunction in the UMNS (17).
Patterns of UMN Dysfunction
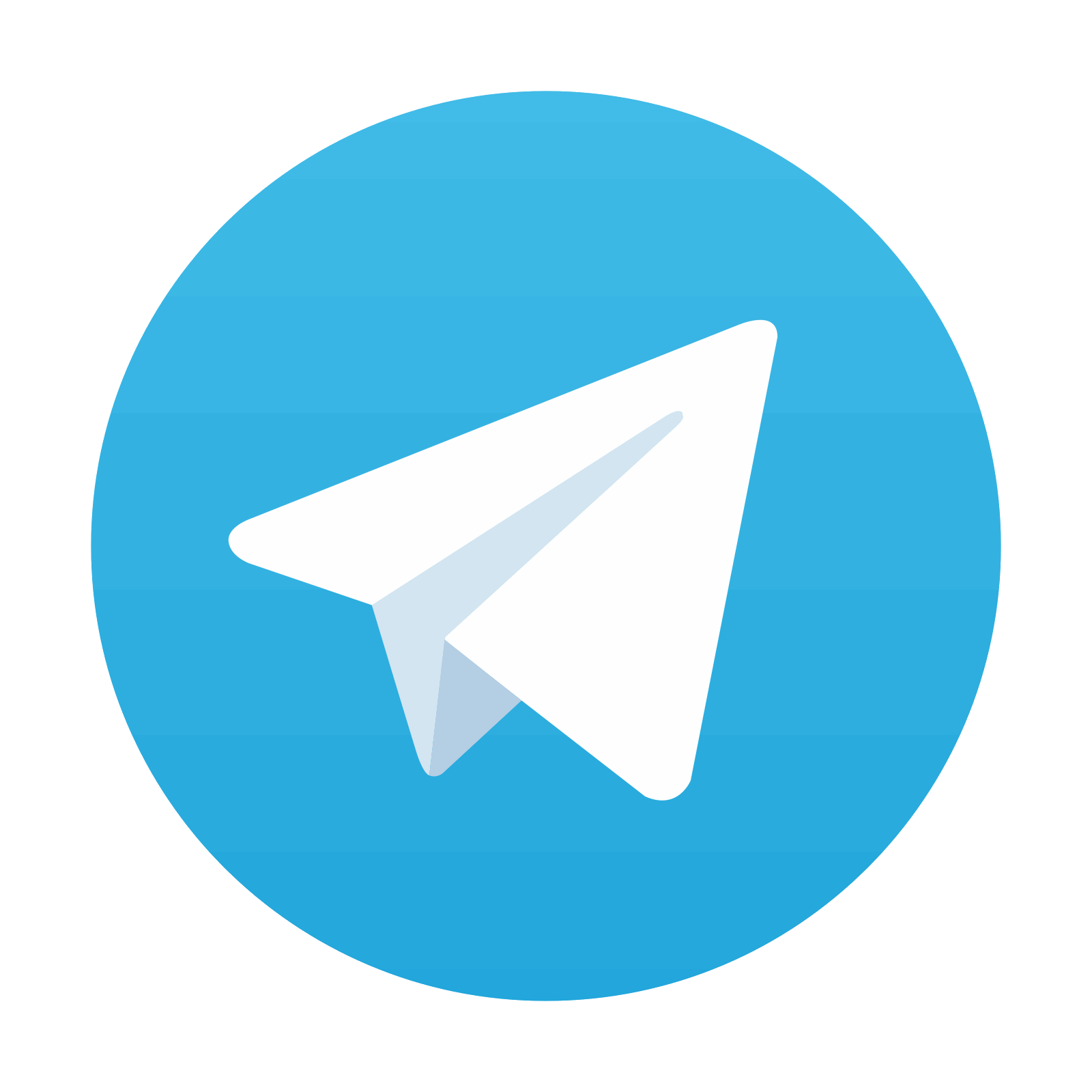
Stay updated, free articles. Join our Telegram channel

Full access? Get Clinical Tree
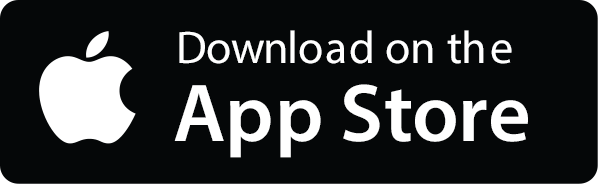
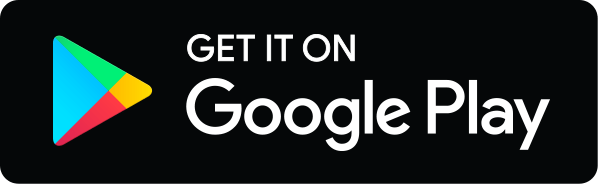