5 Philip Sambrook Bones are extremely dense connective tissue that, in various shapes, constitute the skeleton. Although one of the hardest structures in the body, bone maintains a degree of elasticity owing to its structure and composition. Bone is enclosed, except where it is coated with articular cartilage, in a fibrous outer membrane called the periosteum. Periosteum is composed of two layers, an outer fibrous layer and a deeper elastic layer containing osteoblasts that are capable of proliferating rapidly when a fracture occurs, as will be discussed further in Chapter 10. In the interior of the long bones is a cylindrical cavity (called the medullary cavity) filled with bone marrow and lined with a membrane composed of highly vascular tissue called the endosteum. There are two types of bone: (a) compact or cortical bone and (b) trabecular or cancellous bone. Cortical bone is found principally in the shafts (diaphyses) of long bones. It consists of a number of irregularly spaced overlapping cylindrical units termed Haversian systems. Each consists of a central Haversian canal surrounded by concentric lamellae of bony tissue (Fig. 5.2A). Trabecular bone is found principally at the ends of long bones, and in vertebral bodies and flat bones. It is composed of a meshwork of trabeculae within which are intercommunicating spaces (Fig. 5.2B). The skeleton consists of approximately 80% cortical bone, largely in peripheral bones, and 20% trabecular bone, mainly in the axial skeleton. These amounts vary according to site and relate to the need for mechanical support. While trabecular bone accounts for the minority of total skeletal tissue, it is the site of greater bone turn-over because of its different structure and because its total surface area is greater than that of cortical bone. In addition to its role as a support structure, the bone’s other primary function is calcium homeostasis. More than 99.9% of the total body calcium resides in the skeleton. The maintenance of normal serum calcium depends on the interplay of intestinal calcium absorption, renal excretion and skeletal mobilization or uptake of calcium. Serum calcium represents less than 1% of total body calcium but the serum level is extremely important for maintenance of normal cellular functions. Serum calcium regulates and is regulated by three major hormones: parathyroid hormone (PTH), 1,25-dihydroxyvitamin D and calcitonin (Fig. 5.3). Parathyroid hormone is an 84-amino acid peptide secreted by the four parathyroid glands located adjacent to the thyroid gland in the neck. Calcitonin is a 32-amino acid peptide secreted by the parafollicular cells of the thyroid gland. Vitamin D, from dietary sources (D3) or synthesized in skin (D2), is converted to 25-hydroxyvitamin D in the liver and then to 1,25-dihydroxyvitamin D in the kidney. A number of feedback loops operate to control the level of serum calcium and the two major calcium homeostatic hormones. A calcium-sensing receptor, identified in para-thyroid and kidney cells but also found in other tissues, which senses extracellular calcium levels plays a critical role in calcium homeostasis. Low serum calcium levels stimulate 1,25-dihydroxyvitamin D synthesis directly through stimulation of PTH release (and synthesis). The physiological response to increasing levels of PTH and 1,25-dihydroxyvitamin D is a gradual rise in serum calcium level. To prevent an elevated level of serum calcium, a second set of feedback loops operate to decrease PTH and 1,25-dihydroxyvitamin D levels. These feedback loops maintain serum calcium within a narrow physiological range. Disturbances in these control mechanisms or over/underproduction of these three major hormones can lead to various clinical states, discussed in more detail below. A PTH-related peptide (PTHrP) also plays a role in calcium homeostasis, especially in the fetus and in the growing skeleton. Various cytokines control osteoclast recruitment and activity, including interleukin-1β (IL-1β) and IL-6. A transmembrane protein belonging to the tumour necrosis factor superfamily, plays an important role in osteoclast differentiation and activity (Fig. 5.4A). Its receptor is called RANK (receptor activator of NFкB) since, after binding, a transcriptional factor known as NFкB translocates to the nucleus and appears responsible for expression of genes that lead to the osteoclast phenotype. This process is inhibited by a soluble receptor, osteoprotegerin (OPG), which competes for binding of RANK ligand to produce an inactive complex. Control of osteoblast differentiation and function is achieved by integration of a number of pathways. Bone morphogenetic proteinsand the Wnt signalling pathway are important modulators of osteoblast function and hence bone formation. Sclerostin, a product of the osteocyte, antagonizes the Wnt signalling pathway, which can inhibit osteoblast generation. Bone is continually undergoing renewal called remodelling (Fig. 5.4B). In the normal adult skeleton, new bone laid down by osteoblasts exactly matches osteoclastic bone resorption, i.e. formation and resorption are closely ‘coupled’. Although there is a lesser amount of trabecular bone than cortical bone in the skeleton, because trabecular bone ‘turns over’ between 3–10 times more rapidly than cortical bone, it is more sensitive to changes in bone resorption and formation. Most bone turnover occurs on bone surfaces, especially at endosteal surfaces. Moreover, the rate of remodelling differs in different locations according to physical loading, proximity to a synovial joint or the presence of haematopoietic rather than fatty tissue in adjacent marrow. In clinical practice, it is possible to measure serum biochemical markers of bone remodelling that reflect bone formation and bone resorption (Table 5.1). These markers have been shown to be independent predictors of fracture risk. Table 5.1 Serum biochemical markers of bone turnover Bones develop by one of two processes, either: 1. From a preformed cartilaginous structure (endochondral ossification), or 2. De novo at specific sites in the skeleton (intramembranous ossification). Subsequent skeletal growth involves remodelling of bone. In the growing skeleton, the long bones consist of a diaphysis (or shaft) separated from the ends of the bone (called the epiphyses) by cartilage. The part of the diaphysis immediately adjacent to the epiphysial cartilage is the site of advancing ossification and is known as the metaphysis. Endochondral ossification is a complex process in which the growth plate cartilage is progressively replaced by bone. The growth plate (physis) and bone front steadily advance away from the bone centre, resulting in progressive elongation of bone. Longitudinal growth continues while the growth plate remains open.
BONE STRUCTURE AND FUNCTION IN NORMAL AND DISEASE STATES
Normal skeletal structure and function
Types of bone: cortical and cancellous
Calcium homeostasis and hormonal control
Cellular basis of bone remodelling
Bone formation
Bone resorption
Aminoterminal propeptide of type I procollagen (PINP
Tartrate-resistant acid phosphatase
Bone-specific alkaline phosphatase
Carboxyterminal telopeptide of type I collagen (CTX)
Skeletal development
Stay updated, free articles. Join our Telegram channel

Full access? Get Clinical Tree
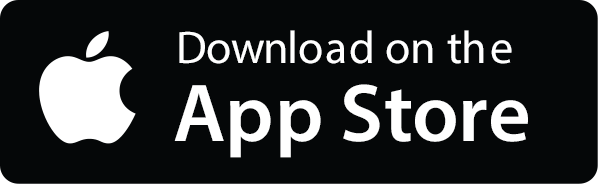
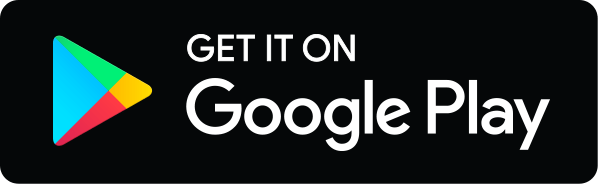