Bone-graft substitutes
Introduction
The number of bone-grafting procedures has increased steadily for years with an estimated 2.2 million procedures carried out per year at a total cost of US$ 2.5 billion. The major indications for bone grafting include bone loss, devitalized bone, loss of structural integrity, and poor healing potential.
The process of bone healing has been extensively studied and is well-understood. The major factors necessary for normal healing to occur include vascularity, stability, progenitor cells, an osteoconductive scaffold, and osteoinductive factors. Failures of bone healing are usually the result of deficiencies of one or more of these factors. Bone-graft materials must be chosen on the premise that the graft must supply that which is lacking by the host and conversely the host must supply that which is not inherent to the graft.
Properties of bone grafts
Bone-graft materials are generally categorized into three main types based on the properties of osteoconduction, osteoinduction, and osteogenic potential (Table 1.4.4-1, Table 1.4.4-2). An ideal graft would possess all of these properties in order to provide the most suitable environment for bone healing. In addition, the bone graft should be biocompatible, bioresorbable, structural, cost effective, and safe. Currently there is only one ideal bone graft that has become the gold standard in grafting, which is the autograft, either in cancellous or cortical varieties. However, donor-site morbidity and the limitations in available volume have driven the market to provide alternative sources.
Graft | Osteoconductive | Osteoinductive | Osteogenic | Structural |
Autograft | ||||
Cortical | ++ | ++ | ++ | +++ |
Cancellous | +++ | +++ | +++ | + |
Calcium phosphate | +++ | No | No | ++ |
Calcium sulfate | +++ | No | No | + |
Coralline | +++ | No | No | ++ |
Allograft | ||||
Fresh | ++ | ++ | No | ++ |
Fresh-frozen | ++ | + | No | ++ |
Freeze dried | ++ | No | No | ++ |
DBM | + | +++ | No | No |
BMP | No | +++ | No | No |
Osteoconductive grafts
Osteoconductive grafts supply a framework for the ingrowth of capillaries and osteoprogenitor cells to assist in the formation of new bone within its structure. They are most commonly used to fill metaphyseal defects in periarticular injuries as well as a stand-alone graft. They may also be used as graft extenders of an osteoinductive substance, such as autograft whenever only an insufficient amount of graft can be harvested. There are many materials on the market that fulfill the criteria for an osteoconductive graft, which include calcium phosphate, tricalcium phosphate, calcium sulfate, coralline grafts, and allograft.
Calcium phosphate is a synthetic osteoconductive scaffold that mimics the mineralized part of bone. It was first used in 1920 to repair surgical defects in rabbits [1]. Calcium phosphate substitutes can be broadly divided into calcium phosphate cements (CPC), tricalcium phosphate, and hydroxyapatites.
The most commonly used preparations of calcium phosphate are the injectable cements for metaphyseal defects and the support of articular fragments. The mechanical compressive strength of CPCs far surpasses that of cancellous bone, however, the strength in tension and shear is much lower. The cement is injected in situ and reaches its final compressive strength of 25–45 MPa after the first 12–24 hours [2]. This limited strength can be augmented by using internal fixation to improve its mechanical properties. In addition, histological studies have shown that the material gradually remodels through osteoclastic resorption and the formation of new bone via vascular ingrowth [3]. The indications for use of calcium phosphate cement as bone graft and for construct augmentation have been extensively studied in the distal radius and tibial plateau. In a randomized multicenter study, 120 patients were randomized to filling a proximal tibial subchondral defect with either autograft or CPC. At the conclusion of the study, it was found that the CPC group had fewer complications and gained greater stability of the articular segment [4]. Other anatomical locations being explored for the use of cement include the proximal humerus, calcaneus, femoral neck, and vertebrae.
Calcium phosphate is also available as ß-tricalcium phosphate, which is commercially available as wedges or blocks. It has a shorter time to incorporation, however, has been shown to be weaker in its mechanical properties. Currently, the indications are similar to those for cements, in addition to its use in osteotomies and as graft extender when coupled with bone marrow or autograft.
Calcium sulfate is also used as a bone-graft substitute for its osteoconductive properties. It is manufactured in many different preparations, such as pellets, powders, and injectables. The indications for calcium-sulfate substitutes are similar to calcium phosphate in that the substance is mostly utilized to fill metaphyseal defects. In contrast to calcium phosphate which is contraindicated in infections, calcium sulfate has a limited role as an antibiotic carrier. In a clinical trial, patients were randomized to antibiotic-impregnated calcium-sulfate bone-graft substitute or antibiotic cement beads. There was no difference in time between eradication of infection and union. However, there were significantly less surgical procedures in the calcium sulfate group [5]. Histologically, calcium-sulfate substitutes have also been shown to resorb calcium phosphates much quicker, which matches the rate of bone integration. However, more complications related to the use of sulfates over other osteoconductive substitutes have been reported. In the literature, complication rates vary from 4–51%, with wound drainage being the most common. Wound drainage does cease as the product is resorbed over time [6].
In addition to synthetically produced substitutes, there is one natural substitute available for use. Coralline grafts are produced by harvesting marine coral exoskeleton and processing the raw materials to convert the calcium carbonate to hydroxyapatite. The hydroxyapatite skeleton provides a surface for vascular and fibrous ingrowth, which eventually converts the hydroxyapatite to lamellar bone [7]. Coralline grafts are available in wedges and blocks and can easily be sculpted for easy use and should be used in fresh fractures to ensure vascular ingrowth. The indications are very similar to those of calcium phosphate and calcium sulfate. In a randomized controlled study, forty patients were randomized to receive either coralline hydroxyapatite or autogenous bone graft after open reduction internal fixation of a tibial plateau fracture. There were no significant differences between the two groups either radiographically or clinically [8]. Biopsies obtained at the time of implant removal demonstrated approximately 25–47% bone ingrowth.
Allografts are commonly used in orthopaedic surgery and comprise about one third of all bone grafts used in North America [9]. Allografts can be processed by a variety of methods including fresh, fresh-frozen, and freeze-dried preparations. Fresh allografts have fallen from favor in trauma surgery due to the time constraints on proper processing, allowing for increased risk of disease transmission. With the use of allograft, there remains a small risk of infection with either hepatitis C, HIV-1, and HIV-2. The risk of transmission of HIV is approximately 1 in 1.5 million while the risk to acquire hepatitis C is 1 in 60,000 [10]. The use of fresh-frozen allografts is common in soft-tissue reconstruction. The grafts are stored at temperatures below -60° C, which decreases the host-immune response by eliminating immunogenic cells and enzymes. Allografts are also supplied as freeze-dried products, which involves removing all the aqueous components and vacuum packing the final product. Essentially, these allografts turn purely osteoconductive as the osteoinductive properties have been eradicated through the sterilization process. The most widely used application of allograft in trauma is cortical or cancellous chips to augment fracture repair and reconstruction of long bone defects.
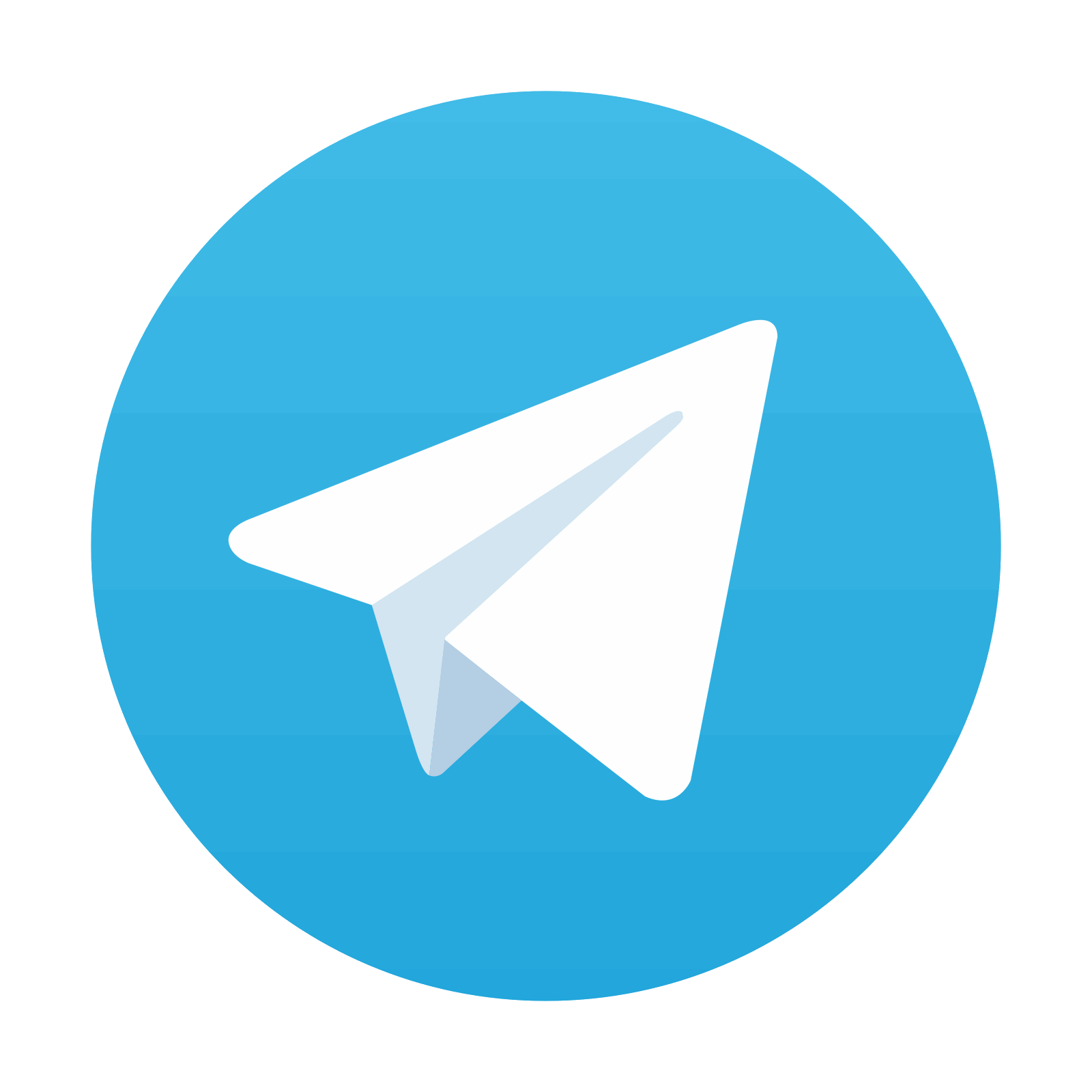
Stay updated, free articles. Join our Telegram channel

Full access? Get Clinical Tree
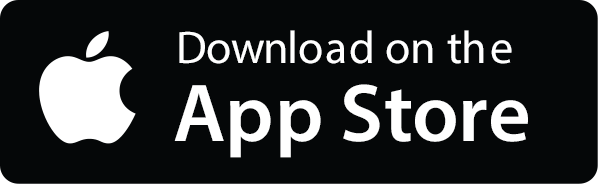
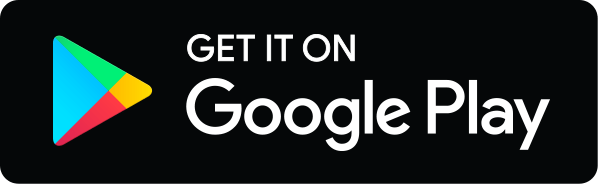