Males
Females
N
Fracture % ≧1
PR
95 % CI
p-value
N
Fracture ≧1 %
PRa
95 % CI
p-value
Leukemia
1229
42.2
0.91
(0.83–1.00)
0.045
1330
30.4
0.99
(0.88–1.12)
0.87
HLb
473
41.0
0.86
0.75–0.98
0.022
489
30.5
0.94
(0.80–1.10)
0.43
NHLc
376
46.3
0.98
0.86–1.12
0.75
176
30.7
0.98
(0.77–1.23)
0.84
CNS tumord
469
30.7
0.66
0.56–0.76
<0.001
436
27.3
0.84
(0.71–1.00)
0.054
Lymphoma
Unlike leukemia, lymphoma originates in the specific white blood cell line of lymphocytes, long established as critical components of the body’s immune system. Lymphoma is divided into two broad types, based on the nature of the abnormal cells identified in a biopsy or on aspiration of the tumor tissue. The least common form, Hodgkin’s disease or Hodgkin’s lymphoma (HL), accounts for only 1 % of all cancers in the United States, and its occurrence is steadily declining; tumors occur in the lymph nodes and the chest area, with the disease progressing downward in an orderly manner from one contiguous lymph node to the next, seldom reaching a stage IV level. Comprised of more than 35 types, the most common form, non-Hodgkin’s lymphoma (NHL), is characterized by tumors in the abdomen and a disorderly, unpredictable progression to any part of the body, with nearly 40 % of cases diagnosed at stage IV [14].
Hodgkin’s Lymphoma
Bone mineral involvement, uncommon in HL and rarely encountered at diagnosis, signifies advanced stage disease and affects treatment and prognosis. As Ozdemirli et al. observe [15], the prognosis in stage IE (the earliest stage) is similar to that of a local nodal disease without osseous effect; by comparison, 5–15 % of stages III–IV HL cases show evidence of diffuse bone involvement. The bone lesions that characterize HL generally respond to combined modality therapy consisting of chemotherapy and radiotherapy, but these interventions are likely to cause further bone deterioration, increasing the risk of vertebral and hip fractures. Whereas BMD deficits in ALL survivors are both serious and well documented, HL survivors examined at five years from therapy appear to have negligible deficits, possibly because of their older age at diagnosis [16]. At the same time, the proportion of HL survivors with BMD at 1.5–2.0 SD or lower is reported to be greater than anticipated, and males have a higher risk of a BMD lumbar Z-score of less than 1.5 % than females, emphasizing the need for subsequent screening as both genders age and encounter the risk of osteoporosis.
Non-Hodgkin’s Lymphoma
Bone marrow involvement and low bone mineral density are more serious in non-Hodgkin’s lymphoma (NHL). In contrast to the 5–14 % of HL patients who show evidence of lymphoma in the bone marrow at diagnosis, the percentage increases to 50–80 % for patients with low-grade (indolent) NHL and 25–40 % for those with high-grade (aggressive) NHL [17]. Moreover, bone marrow involvement is a critical factor in determining the staging of NHL and is generally associated with stage IV; it is treatable, as is the disease itself, with different combinations of chemotherapy, radiation, and bone marrow transplant depending on the nature and severity of the disease [18].
Although NHL therapy is associated with long-term sequelae including osteoporosis and the risk of vertebral and hip fractures, low BMD can also be present at the time of diagnosis. In patients age 50 and over, Westin et al. report that 54 % of men and 40 % of women had baseline osteopenia—a finding that was independent of disease stage or bone marrow involvement yet indicative of the observation that older men with lymphoma may be at higher risk of osteoporosis than those in the general population [19]. The Westin analysis of newly diagnosed patients with lymphoma determined that two doses of the third-generation bisphosphonate, zoledronic acid (ZA), together with calcium and vitamin D supplementation over a period of one year, prevented bone loss in this cohort. A subsequent 2014 study [20] of newly diagnosed adults with lymphoma receiving chemotherapy reported significant BMD loss at the lumbar spine (−2.7 ± 3.9) and total hip (−2.6 % ± 4.5 %) as well as osteoporotic fractures after only one year of treatment, while an earlier study of 13,572 patients (age = ≥65) found increased risk of fracture and osteoporosis at up to 11 years of follow-up [21].
In studies of childhood survivors of lymphoma, estimates of osteoporosis risk is often conflicted due to varied degrees of disease severity, differing treatment strategies, small sample sizes, time from therapy completion, and study heterogeneity. For example, some researchers postulate that a decrease in BMD is time dependent, while others do not. An analysis of the long-term effects of treatment for BMD in both ALL and NHL, in which treatment protocols are similar [18], found that after five or more years of remission, more than one-third of patients treated with chemotherapy alone experienced a long-lasting BMD deficit. Those who received additional prophylactic irradiation had even lower BMD values, while others with bone marrow transplantation, combined with total body irradiation, had significantly reduced BMD at the total hip and femoral neck. The study further confirmed that male gender is a known risk factor for increased susceptibility to osteoporosis and fractures following chemotherapy, radiation, or bone marrow transplantation conditioned by total body irradiation. Yet, one of the few studies focused specifically on bone density in both types of lymphoma reported that childhood survivors evidenced no significant deficits in bone mass and maintained BMD within the normal range when examined 1–5 years following the end of therapy [22]. Because bone density loss is evident in lymphoma at baseline and can be exacerbated by standard treatment, some of the leading researchers in the field strongly advocate that routine screening should be part of the National Comprehensive Cancer Network guidelines, together with the use of prophylaxis in the form of calcium and vitamin D supplementation, zoledronic acid, and other bone-directed therapies [23].
Multiple Myeloma
Myeloma is a cancer that affects a type of white blood cells called plasma cells; as part of the immune system, they produce antibodies that protect the body from infection and disease. The disease is generally called “multiple myeloma” because malignant cells affect multiple areas of bone marrow. The incidence of myeloma increases with age (most people are diagnosed at age 65 or older), is almost twice as common in African-Americans than in Caucasians, and is relatively more prevalent in men than in women [24]. As the second most common hematologic malignancy accounting for about 15 % of these diseases, multiple myeloma has the highest occurrence of bone involvement––nearly 80 % of patients––among all malignant diseases [25].
Concentrated in the bone marrow and in the hard, outer parts of bone, myeloma cells collect in various bones, crowding out normal cells and creating multiple myelomas. As a result, “punched out” lytic lesions occur which weaken and damage bones, primarily the spine, pelvis, and rib cage. As bone density declines due to ongoing disease process, the relative risk for fracture increases [25]. See Fig. 1. Figure 2 shows diffuse myelomatous disease of the thoracolumbar spine in a patient with newly diagnosed multiple myeloma, demonstrating how quickly this disease can progress even in relatively young individuals. In terms of the mechanism [26], the receptor activator of nuclear factor kappa-B ligand (RANKL) is a potent inducer of osteoclast formation that binds to its receptor, RANK, located on osteoclasts. RANKL expression can also enhance the effects of other factors such as macrophage inflammatory protein 1 alpha (MIP-1α) and interleukin 6 (IL-6) in stimulating osteoclast formation and activity in the bone marrow microenvironment. The resulting bone resorption, in turn, leads to the release of growth factors that further increase myeloma cell production and can lead to hypercalcemia—an interaction known as the “vicious cycle of bone metastases” [3].



Fig. 1
Exponential relationship between bone density and fracture risk (Source: Adapted from Faulkner [25], with permission of the American Society for Bone and Mineral Research)

Fig. 2
Metastatic multiple myeloma of the thoracolumbar spine. Image demonstrates diffuse myelomatous disease of the thoracolumbar spine in a 30-year old female, with epidural soft tissue extension of the tumor at T-3. In addition, multiple compression deformities are seen along the thoracolumbar spine, the worst of which is at T-3 where there is vertebral plana (Source: Courtesy of Thomas Jefferson University Department of Radiology)
Precursor Diseases
In assessing the development of osteoporosis and fractures in multiple myeloma, attention must be given to its precursors: (1) monoclonal gammopathy of unknown significance (MGUS) which is generally regarded as asymptomatic but may progress to myeloma, and (2) “smoldering multiple myeloma,” an early stage of multiple myeloma that is absent of bone lesions, hypercalcemia, and kidney damage [27]. Evidence now increasingly supports the theory that myeloma is a continuum of disease, from MGUS to smoldering myeloma to multiple myeloma.
Recent data indicate the presence of bone disease even in patients with these precursor conditions, precipitating a strong recommendation that routine DXA scans be employed to determine affected patients. A population-based retrospective cohort trial, involving 488 MGUS patients, reported a 6.3-fold increase in fracture risk at most axial, but not peripheral sites [28], while a subsequent study of 5326 MGUS patients revealed a 1.6-fold increased risk of any fracture, again with a higher degree of axial as opposed to distal fracture. Bisphosphonates, used in the clinical management of MAGUS and smoldering multiple myeloma, have a marked effect on BMD. A study by Pepe et al. involving alendronate in MGUS demonstrated a 6.1 increase in mean BMD of the lumbar spine and a 1.5 % increase in the total femur [29], while in a trial involving zoledronic acid, Berenson et al. reported a median increase in BMD of +15.0 % in lumbar spine and +6.0 % in total hip, with no new fractures [30]. However, neither alendronate, nor pamidronate, nor zoledronic acid are effective in halting the progression to active multiple myeloma [31].
Active Multiple Myeloma
A number of interacting factors including increased osteoblastic activity and a heightened number of osteoclasts, decreased activity in the surrounding osteoblasts, increased markers of bone resorption with decreased markers of bone production, and persistent lytic lesions that inhibit bone repair all contribute to the severity of active multiple myeloma [32]. In newly diagnosed multiple myeloma patients, some 80 % have bone disease. Over 60 % of the cases of osteopenia, osteoporosis, and pathological fractures involve the spine as opposed to 90 % in metastatic prostate cancer and 75 % in metastatic breast cancer [33]. A study by Dhodapkar et al. found bone density to be significantly decreased at lumbar spine and femoral neck with duration of disease at >12 months as well as an independent association between female sex and lower BMD [34]. In another retrospective population-based cohort of 165 residents from Olmstead County, Minnesota, Melton et al. [35] reported that at the time of initial myeloma diagnosis, 16 times more fractures occurred than were expected and that subsequently a ninefold increase in fractures was observed. Although two-thirds were pathologic fractures of the vertebrae and the ribs, the risk of subsequent osteoporotic fracture was elevated twofold. Admittedly this increase coincides with a time of decreased survival probability.
Treatment
Bisphosphonates, which are used as therapy for osteoporosis, lytic bone lesions, and hypercalcemia, have become the standard of treatment in multiple myeloma. Administered intravenously, pamidronate and zoledronic acid (ZA) have proved particularly effective. For pamidronate, a dosage of 30 mg monthly has been recommended to protect against skeletal complications [36]. In comparison, 4 mg of ZA, administered every 3–4 weeks, has a more rapid infusion time and is more potent, reducing the overall risk of skeletal damage by an additional 16 % over pamidronate [37]. Moreover, despite the side effects including osteonecrosis of the jaw, ZA has been found to reduce bone loss and to independently improve overall survival, indicating a possible synergy with first-line myeloma therapies [38]. If myeloma should affect the spine resulting in a vertebral compression, both vertebroplasty and kyphoplasty (the injection of bone cement into the vertebrae) have been judged to be safe and effective [31].
Finally, daratumumab has recently become the first monoclonal antibody approved by the FDA for treatment of multiple myeloma cases that are refractory to proteasome inhibitors and immunomodulatory drugs. Given its favorable safety profile and its demonstrated efficacy, daratumumab is a much-needed targeted approach and a potentially transformative drug in the armamentarium of multiple myeloma [39, 40]. If this agent proves effective, future generations may not experience the lytic bone lesions and related disability that we now observe.
The incidence of osteoporosis and fractures in hematological malignancies is an active research area. In lymphoma and other childhood cancers affecting bone, further research on survivors should elucidate the impact on BMD of such risk factors as genetic predisposition, adverse treatment effects, poor nutritional status, restricted physical activity, and the natural aging process [41]. In myeloma, the marked improvement in 5-year survival rates from 12 % in 1960–1963 to 46.6 % in 2005–2011 [42, 43] with even significantly higher percentages at many institutions, underlines the need to ensure optimal use of bisphosphonates as well as to explore the treatment potential of newer agents such as recently FDA-approved monoclonal antibody, denosumab [31].
Solid Tumors
Whereas multiple myeloma affects the skeleton by means of bone lesions, solid tumors including breast, prostate, lung, and kidney metastasize to bone. Before examining the effect of solid tumors themselves as well as their treatments on bone, it might be helpful to consider the relation between solid tumor cancer, baseline BMD, and fracture risk, with particular reference to breast and prostate cancer. High rather than low bone mineral density is a known prognostic factor for both postmenopausal breast cancer in Caucasian women and prostate cancer. Zmuda et al. have shown that women 65 years and older with high BMD have a 2 to 2.5-fold increase in breast cancer risk [44], while a study by Zambetti and Tartter of postmenopausal women (median age = 57) with breast cancer confirmed the association between high BMD and high risk of breast cancer [45]. The authors speculate that elevated BMD in those with breast cancer may be related to estrogen levels, which are believed to increase the risk of breast cancer in some women but contribute favorably to bone mass accrual. While some early studies demonstrated a correlation between endogenous androgen levels and elevated BMD, findings were inconsistent based on limited hormonal assessment time points.To address these discrepancies, Farhat et al. examined a cohort of older men with no history of prostate cancer [46]. Unexpectedly, they demonstrated that higher BMD of the total body was significantly related to lower risk for prostate cancer, supporting the findings of the NHANES I Epidemiology Follow-Up Survey. Although not significant statistically, NHANES data found a decline in prostate cancer risk was associated with higher quartiles of bone density [47]. Findings still remain difficult to interpret because total body BMD results in Farhat et al. were associated with lower prostate cancer in terms of high-grade tumors, yet no association with total hip or spine BMD or with low-grade tumors was observed. It remains uncertain if practitioners of rehabilitation patients can take comfort in higher BMD findings of a newly diagnosed patient with solid bone tumors. The above studies do not specifically address fracture risk. Moreover individuals undergoing active cancer treatment may be globally weak, predisposing them to falls that can lead to fracture.
Nonetheless, in patients with newly diagnosed breast and prostate cancer, low bone density and fracture risk resulting in osteopenia and osteoporosis do exist. In such cases, interventions should be taken to address the clinical risk of fracture. Both postmenopausal and premenopausal women with breast cancer may already have low BMD or develop this condition soon after diagnosis, leading to the American Society of Clinical Oncology’s recommendation of routine DXA scans [48]. Moreover a study by Chen et al. demonstrates that both fracture and fall risks are significantly elevated after a diagnosis of breast cancer but not before [49].
In prostate cancer, low bone mineral density is again present at baseline. For example, Berruti et al. found that at baseline, 46 % of prostate cancer cases were osteopenic and 14 % were osteoporotic at lumbar spine, while 40 % were osteopenic and 4 % were osteoporotic at the hip [50].
Physiologic Basis of Bone Metastases
Bone metastasis is generally defined as the spread of cancer cells from their primary site through the blood stream or lymph system to a new organ where they can settle and grow [51]. Metastases are found primarily in bones in the center of the body, particularly the spine. Breast and prostate cancer account for 70–80 % of metastatic bone disease, while metastases occur in 40 % of advanced lung cancer patients [52]. In addition, multiple myeloma and thyroid and kidney cancers metastasize to bone [53].
Metastases manifest themselves in two ways: (1) by affecting osteoclasts and weakening bones without forming new bone, they result in the osteolytic or lytic lesions characteristic of breast cancer; (2) by affecting osteoblasts and promoting bone formation without destroying old bone, they produce abnormally hard bones (sclerosis) with the osteoblastic lesions evident in prostate cancer. As described in the discussion of multiple myeloma, bone metastases are the result of an interaction between tumor cells and the metastatic site characterized as a “vicious cycle”: as tumor cells simulate bone cells to cause either bone destruction or bone formation, the bone microenvironment provides tumor cells with growth factors that stimulate the tumor cells to grow [26]. The resulting skeletal-related events (SREs), including severe bone pain, pathological fractures, spinal cord compression, hypercalcemia, impaired mobility, and the need for radiotherapy or surgery, are often exacerbated by the common risk factors for osteoporosis [54]. In a later section of this chapter, we will cover specific recommendations for fixation of metastatic bone lesions, precautions during physical therapy before and after fixation, and how to approach a patient with bone metastases that are not suitable for fixation.
Cancer Treatment-Induced Bone Loss
The challenges to bone health posed by cancer itself are further compounded by the drugs used to treat it, referred to as cancer treatment-induced bone loss (CTIBL). Although these drugs, specifically aromatase inhibitors in women (anastrozole, exemestane, letrozole) and androgen-deprivation therapy in men, have significantly increased the overall survival rates of cancer patients, they have exerted adverse effects on their bones, decreasing BMD and increasing the risk for osteopenia and osteoporosis. Moreover, these drugs have come into widespread use at the same time as age-related factors, contributing to bone degeneration, are exerting their effect across the cancer spectrum.
Patients under cancer treatment should not only adhere to the standard nonpharmaceutical therapies for osteoporosis, including calcium and vitamin D supplementation, weight-bearing exercise, and modification of such lifestyle factors as smoking and alcohol, but should also consider receiving bisphosphonate therapy with its established potency and known minimal effects [55]. The following section will consider the principal interventions used in treating breast and prostate cancer as well as strategies for maintaining bone health.
Breast Cancer
Early Menopause
Affecting 1 % of women under the age of 40 years [56], early menopause is precipitated by a number of factors ranging from genetics, smoking, and low body mass to autoimmune diseases, chromosome disease (Turner syndrome), and surgery (bilateral oophorectomy). In addition, chemotherapy used to treat breast cancer is likely to cause ovarian failure and loss of estrogen production, leading to sudden or premature menopause and increasing the risk of osteoporosis and fractures. Chemotherapy-induced ovarian failure (CIOF) occurs in more than 50 % of premenopausal women within the first year of treatment [57]. In a trial of 49 premenopausal women with stage I/II breast cancer receiving adjuvant chemotherapy, 39 experienced ovarian failure and, within that group, the median decrease in bone density was 7.7 % in lumbar spine and 4.6 % in femoral neck at 12 months [58]. Other studies have reported similar results confirming that early menopause leads to lower BMD and a higher risk of osteoporosis. The earlier menopause occurs, the greater the BMD loss later in life. In addition, tamoxifen, frequently used in premenopausal women with estrogen receptor-positive breast cancer, has been shown to produce an average annual decrease in lumbar BMD of 1.44 % over a 3-year period. (In comparison, tamoxifen prevents bone loss in postmenopausal women.) [59].
Given their superior efficacy and safety, aromatase inhibitors (AIs) such as anastrozole, letrozole, and exemestane are increasingly used to lower estrogen levels and impede tumor growth, but they too have a deleterious effect on bone. Although indicated for use only in postmenopausal women, recent studies have shown that AIs may have a positive effect in women with premature ovarian failure (POF) if they are combined with other medications. An examination of the effect of endocrine therapy together with zoledronic acid on such women [60] found that after a 3-year period, endocrine therapy alone resulted in a significant BMD loss of 11.3 % at lumbar spine and 7.3 % at trochanter. However, with the addition of zoledronic acid to the treatment regimen, BMD was stable at three years and increased at both lumbar spine and trochanter at five years.
Most recently, the results of two large clinical trials—the Tamoxifen and Exemestane Trial (TEXT) [61] and the Suppression of Ovarian Function Trial (SOFT) [62], indicated that the AI exemestane, combined with ovarian suppression to achieve low estrogen levels, was superior to both tamoxifen–ovarian suppression and tamoxifen alone. Although these results have been termed “practice changing,” an increased use of exemestane, as opposed to the bone-conserving tamoxifen, will have adverse effects on bone.
An increased number of fractures are also evident in early menopause. In a Swedish study of 733 women with a follow-up at 11 years, those with early menopause had 50 % more fractures than those under the age of 70 who experienced a normal menopause [63]. The results of a 2004 Rotterdam population-based cohort study showed that early menopause compared with menopause at older than 50 years resulted in a significant increase in vertebral fractures at a relative risk of 2.4 but that, recognizing its adverse effects, estrogen use for more than three years was highly protective against fractures [64].
Postmenopausal Status
Whereas early menopause signifies that women will spend a longer time with weakened bone, postmenopausal women with breast cancer concurrently face issues posed not only by disease- and age-related bone loss but also by CTIBL. Osteoporosis may occur at any point in breast cancer progression: at the time of diagnosis as well as during cancer treatment and cancer-free survival, while hypogonadism induced by cancer therapy may further compound rapid loss of BMD [65]. Bone metastases and CTRBL require different interventions to prevent and stabilize BMD and fracture risk.
Cancer Treatment: Induced Bone Loss
Aromatase Inhibitors (AIs)
Favorable randomized trial results have led to the increasing use of AIs in breast cancer to the extent that they are now regarded as the first-line adjuvant therapy for postmenopausal women with hormone-responsive tumors. By lowering estradiol levels, both the nonsteroid reversible inhibitor, anastrozole, and the steroid irreversible inhibitor, exemestane, have been shown to reduce risk of breast cancer by at least 50 % [66, 67]. Although AIs have been demonstrated to have a positive effect on breast cancer risk, they have not been approved by the FDA for chemoprevention. Moreover, studies reveal that they exert damaging effects on bone.
With respect to bone loss and fracture risk, results of the Arimidex, Tamoxifen, Alone or in Combination (ATAC) trial indicate a significant loss in BMD of 6.08 % at the lumbar spine and 7.24 % at total hip after five years of anastrozole treatment; 43 % of women with normal BMD at treatment initiation developed osteopenia, compared with 9 % on tamoxifen. However, Eastell et al. have shown that anastrozole treatment-related bone loss does not continue into an off-treatment period: at six years after the ATAC trial, lumbar spine BMD increased by 2.35 % and at seven years by 4.02 %. Following two years of treatment with exemestane, loss of volumetric BMD at the distal radius and tibia was significantly greater than in a placebo group, while cortical thickness and area declined 7.9 % versus −1.1 % in the controls—a notable finding because 80 % of bone mass is cortical [68, 69]. At 24 months, patients on letrozole had a greater decrease in total hip BMD (3.6 %) and lumbar spine (5.35 %) than those on placebos [70]. All three AIs are associated with an increased risk of fractures conditions, and regular monitoring is recommended [71].
Chemotherapy and Radiation
Despite advances in cancer medications, chemotherapy and radiation therapy are still employed to achieve clearly defined objectives. Neoadjuvant (preoperative) chemotherapy is used to shrink or slow the growth of tumors, lowering the mastectomy rate, decreasing adverse events, and enabling researchers to assess tumor behavior in situ [72]. Patients treated with a high dose of radiation are at risk of rib fractures based on dosage: the lower the dosage (less than 50 Gy), the lower the fracture rate. Moreover, when radiation is combined with chemotherapy or surgery, the incidence of rib fractures increases compared to that in patients treated solely with a lower dose of radiation [65].
Selective Estrogen Reuptake Modulations
In terms of hormone therapies, studies indicate that the selective estrogen reuptake modulations (SERMs), tamoxifen and raloxifene, are efficacious and safe in breast cancer patients and do not appear to cause skeletal complications in postmenopausal women. Both agents are FDA approved for preventing breast cancer. As noted above, tamoxifen has different effects before and after menopause. Whereas tamoxifen is associated with bone loss in premenopausal women, it is linked to increased BMD following menopause, not only preserving and increasing BMD at the spine and hip but also reducing fracture risk [73]. A Canadian trial found that osteoporosis-related fracture risk decreased by 32 % and hip fracture risk by 53 % [74].
Originally FDA-approved for prevention and treatment of osteoporosis, raloxifene is now sanctioned for reduction of breast cancer risk in postmenopausal women with osteoporosis or those with elevated risk of breast cancer. As the STAR Breast Cancer Prevention trial revealed [75], raloxifene and tamoxifen are equally effective in reducing the risk of invasive breast cancer by about 50 %, with tamoxifen being somewhat more effective at preventing noninvasive disease. It should be noted, however, that tamoxifen has not gained widespread acceptance because it increases endometrial cancer and thromboembolic events including blood clots, whereas raloxifene leads to far less toxicity and retains its 38 % effectiveness over the long term.
Treatment of Bone Metastases
Several recent studies have elucidated the role of bisphosphonates in the treatment of skeletal-related events in postmenopausal breast cancer, comparing the most frequently used intravenous agent, ZA, with denosumab. A meta-analysis conducted by the Early Breast Cancer Trialists’ Collaborative Group [76] determined that bisphosphonate therapy significantly reduced the development of bone metastasis and the rate of breast cancer recurrence, in addition to protecting bone health, particularly in women receiving aromatase inhibitors. The Zometa-Femara Adjuvant Synergy Trial (Z-FAST) focused on the timing of treatment with ZA in postmenopausal women with early-stage breast cancer receiving adjuvant letrozole therapy and found that upfront treatment with ZA, as opposed to delayed administration, significantly and progressively increased BMD, resulting in a mean difference in lumbar spine and total hip of 8.9 % and 6.7 %, respectively, at the 5-year mark [77]. Comparisons of ZA and denosumab tend to favor the latter, but individual circumstances must be taken into account in determining appropriate personalized treatment. In a randomized, double-blind study [78], denosumab demonstrated greater effectiveness in delaying or preventing SREs in women with breast cancer metastatic to bone; moreover, its benefits include the convenience of a subcutaneous injection and no requirement for renal monitoring. Overall survival, disease progression, and rate of adverse events were similar. Further, a 2012 trial [79] involving more than 2000 patients with advanced breast cancer, reported that fewer patients on denosumab compared with ZA had first SREs, multiple SREs, pathologic fractures, radiation therapy to bone, and hypercalcemia; there was also a “clinically meaningful” improvement in health-related quality of life for those on denosumab. In addition to pamidronate and ZA denosumab was added to list of recommended treatments for bone metastases by the American Society of Clinical Oncologists in 2011 [80].
Treatment of CTIBL
The primary goal of managing CTIBL is to reduce fracture risk with a threefold approach; screening at age 60–65 for women with increased osteoporosis risk, lifestyle changes including calcium and vitamin D supplementation, weight-bearing exercises, and cessation of smoking, together with pharmacologic interventions, specifically bisphosphonates and, more recently, denosumab.
Although proven effective, first-generation oral bisphosphonates (clodronate) have largely given way to more potent second-generation intravenous compounds (pamidronate) and third-generation intravenous drugs (zoledronic acid-ZA) in treating CRIBL. As Blanchette et al. point out [81], oral bisphosphonates remain an affordable and “reasonable” treatment for breast cancer patients with limited bone disease; however, pamidronate, ZA, and denosumab have superior efficacy and are used in more advanced cancer and hypercalcemia. Denosumab is currently the only FDA-approved therapy for the treatment of AI-induced bone loss in women with early-stage breast cancer. Since FDA approval, a randomized, double-blind trial has further determined that denosumab, compared with placebo, has a significantly delayed time to first fracture in addition to an overall reduced number of fractures [82]. In 2015 a new trial showed that denosumab had an 18 % reduced risk of disease recurrence; together with its demonstrated 50 % reduction in fractures caused by adjuvant aromatase inhibitors [83].
Safety, compliance, and cost are also relevant factors in selecting treatment. Similar safety profiles exist for both ZA and denosumab; however, ZA is known to increase incidence of osteonecrosis of the jaw and renal toxicity, while denosumab poses a risk of severe hypocalcemia. Both denosumab, administered at 6-month intervals, and ZA, administered monthly for at least the first year, have greater compliance rates than oral bisphosphonates. Finally the advantages of denosumab must be balanced against its expense, particularly because ZA no longer has patent protection, potentially affecting its cost [84]. The cost of denosumab exceeds that of ZA and of oral bisphosphonates by a significant margin, raising potentially serious insurance issues. Cost-effectiveness studies, hindered by discrepant methodologies, end point variabilities, and changes in drug prices, are difficult to compare and await a more consistent, standardized approach [85].
Research to date clearly indicates that bone loss in breast cancer can be prevented and treated. What is needed is greater awareness of the incidence of concomitant bone loss and its consequences as well as more frequent screening. New findings on effective therapies are also emerging. For example, a recent phase III clinical trial established that following one year of monthly treatment with ZA, patients with metastatic breast cancer can scale back to an every 12-weeks maintenance dosing schedule with equal efficacy, reducing the risk of adverse side effects, patient inconvenience, and cost [86].
Prostate Cancer
Bone loss in breast and prostate cancer is similar in several respects, including time of occurrence, cause, and treatment. Like breast cancer, prostate cancer occurs primarily in the elderly and thus concurrently with osteoporosis. Bone is adversely affected by both the disease itself and by associated treatments, and bone loss responds to treatment with medications such as bisphosphonates and denosumab.
Just as aromatase inhibitors are used to lower estrogen levels in women, so androgen-deprivation therapy (ADT), involving bilateral orchiectomy or gonadotropin-releasing hormone (GnRH) analogues, is used to shrink prostate cancer cells or slow their growth by decreasing testosterone levels. Testosterone reduction, in turn, suppresses estrogen, now recognized as equally or potentially more important than testosterone in prostate cancer bone loss [87]. For many years, testosterone was regarded as the hormone primarily responsible for bone remodeling in men; now reduction in both circulating testosterone and estrogen is known to decrease osteoblasts and increase osteoclasts, leading to the erosion of trabecular bone by osteoclasts and the formation of structurally weak sclerotic-woven bone by osteoclasts [88]. Depending on the specific case, both the WHO Fracture Risk Assessment Tool (FRAX) and DXA testing are recommended to obtain baseline measurements.
Bone Metastases
In both breast and prostate cancer, bone metastases occur in up to 80–90 % of patients with advanced disease [89]. They lead to serious skeletal complications including pain, hypercalcemia, impaired mobility, spinal cord compression, and fractures, particularly in vertebral bones—conditions that are exacerbated in men by androgen-deprivation therapy (ADT), the principal treatment for metastatic prostate cancer [88]. However, it should be noted that only 7–16 % of fractures in prostate cancer are secondary to bone metastases. The others result from pretreatment low bone density, forms of cancer-induced treatment bone loss particularly due to AI or ADT, physical immobility, and nutrient deficiencies.
ADT encompasses (1) bilateral orchiectomy, (2) GnRH analogues, and (3) combined androgen blockade (CAB), which combines either GnRH analogues or orchiectomy with an antiandrogen such as bicalutamide [90]. Its objective is to prevent the production and use of androgens by the body, thereby impeding the growth and survival of cancer cells; however, it does not halt disease progression. Although most men with advanced prostate cancer initially respond well to ADT, the cancer can return, at which stage it is termed “castration resistant,” signifying that ADT is no longer effective. A comparison of several cancer treatment modalities and their associated bone loss in the lumbar spine is presented in Fig. 3 [91].


Fig. 3
Bone loss associated with various cancer therapies. Menopausal women lose bone at a rate of 1–2 % yearly. Cancer treatments, such as aromatase inhibitor therapy and chemotherapy, accelerate this process, leading to significant bone loss and subsequent skeletal complications (Source: Reproduced with permission from Guise [91])
Treatment of Bone Metastases
Bisphosphonates, particularly ZA and denosumab, have become the established therapies to slow or reverse bone loss caused by skeletal metastases. Although oral bisphosphonates cannot significantly reduce metastatic bone pain [92], intravenous forms, particularly ZA, have demonstrated positive results, with a potency of 100–1000 times that of pamidronate in vitro systems [92]. In 2002 the FDA approved ZA use for metastatic prostate cancer, citing a study by Saad et al. which found that fewer patients treated with 4 mg of ZA had SREs (33.2 %) compared with the placebo group (44.2 %); the median time to the first SRE was 488 days compared with 321 days for placebo [93]. As of 2016, several labeling changes have been instituted for Zometa including warnings about subtrochanteric and diaphyseal femoral fractures, acute phase reaction, and osteonecrosis of the jaw [94].
The steadily increasing role of denosumab in prostate cancer is supported by a number of clinical trials that demonstrate its efficacy not only in preventing or delaying fractures in men with metastases but also in slowing the spread of cancer to bone in men without metastases but with rising PSA levels [95]. In a path-breaking comparison of ZA with denosumab, involving 342 medical centers in 39 countries, Fizazi et al. [96] reported that the median time to the first SRE was significantly longer with denosumab (20.7 months) than with ZA (17.1 months); the former also resulted in a decreased rate of multiple SREs.
In cancer, both agents are used more frequently than they are for postmenopausal osteoporosis and studies indicate less frequent dosing with denosumab with respect to cancer patients. With both drugs approved for use, attention is now focused on dosing frequency and reduced dosages; if further randomized trials can confirm no significant difference between treatments every 6–12 months as opposed to every 3–4 weeks, the outcome would be lower costs for both patients and the healthcare system, as well as potentially decreased drug side effects [97]. Still, questions remain about the relative benefits of ZA and denosumab [98]: What is the cost-effectiveness of each treatment, particularly given the expiration of the ZA patent in 2013 and the probable extension of the patent for denosumab for some time in the future? Apart from clear recommendations of agents to treat osteoporosis in persons with impaired renal function, in which denosumab is an option but ZA is contraindicated, are there other reasons why some patients would benefit more from one agent than the other? Can denosumab be used to delay onset of metastasis? What is the effect of denosumab on bone pain and quality of life [99]?
Cancer Treatment-Induced Bone Loss
Whereas ADT was once used primarily in metastatic prostate cancer, it is increasingly administered in less serious stages of the disease. During ADT, circulating levels of testosterone decrease by >95 % and estrogen by >80 % [100] BMD begins to decrease within months of ADT initiation; at the end of the first year of therapy, it has declined by 2–8 % in the lumbar spine and from 1.8 to 6.5 % in the hip, continuing to diminish at a slower rate with long-term ADT [101]. Extended duration of ADT also markedly increases fracture risk, with an estimate of some 3000 excess fractures per year attributable to hormone agents; older age and more advanced stages of cancer may confound these results [102]. A 2015 study of risk fracture reported that patients receiving ADT experienced a 10.8 % fracture rate compared to 3.2 % for those not receiving ADT, results that are possibly confounded by pathologic fractures and spinal cord compression [103].
Two small randomized controlled trials with bisphosphonates have shown limited positive results in men without bone metastases. An investigation of the use of IV pamidronate in patients treated with the GnRH agonist, leuprolide, found pamidronate prevented bone loss in the hip and lumbar spine [104] while a subsequent study of 4 mg ZA as therapy for ADT in men with nonmetastatic prostate cancer demonstrated that, over a period of three months in one year, ZA actually significantly increased BMD in the lumbar spine by 5.6 % as opposed to placebo, with increases also observed in the BMD of the femoral neck, trochanter, and total hip. More recently, a once-yearly infusion of 4 mg ZA was shown to significantly increase the BMD at lumbar spine by 4.0 % in nonmetastatic prostate cancer, indicating that less-frequent dosing may be a more convenient but still effective strategy for preventing osteoporosis in men receiving GnRH [105].
Although these studies did not follow patients long enough to detect fracture risk, trials involving toremifene and denosumab have shown their efficacy in reducing fracture risk in high-risk prostate cancer patients receiving ADT. In a 2013 study comparing toremifene with placebo in such a population [106], the incidence of new vertebral fractures was 2.5 % in the toremifene group compared with 4.9 % in the placebo group—a significant relative risk reduction of 50 %. Toremifene also increased BMD at the lumbar spine, hip, and femoral neck and decreased markers of bone turnover. With regard to denosumab, the Hormone Ablation Bone Loss Trial (HALT-138) (NCT00089674) administered 60 mg of denosumab twice yearly to nonmetastatic patients at high risk for fractures; not only did BMD of the spine and hip increase significantly, but the incidence of new vertebral fractures decreased at 12, 24, and 36 months with a cumulative incidence of 1.5 % in the denosumab group versus 3.9 % in the placebo group at the end of three years—a 62 % reduction. These results were critical in gaining FDA approval for denosumab (Prolia) to increase bone mass and prevent fractures in men receiving ADT as well as in women taking AIs for breast cancer [107]. Routine bone scans every 1–2 years are recommended during treatment and relevant lifestyle modifications should be implemented [108].
Lung Cancer
Although research has concentrated on the impact of breast and prostate cancer on bone, a limited number of studies have examined the lung and, to a lesser extent, the kidney. After breast and prostate, the lung is the third most common site of origin for metastatic cancer deposits in bone, specifically the spine, pelvis, proximal long bones and, uniquely, the bones of the hands and feet. Lung cancer is divided into two categories based on cell size and tumor type (as determined by microscopic examination): non-small cell lung cancer (NSCLC) accounts for approximately 85 % of cases and small cell lung cancer (SCLC) comprises about 15 % [109]. However, SCLCs grow more rapidly and are more likely to metastasize, even to the point where metastasis can be evident at the time of diagnosis. The “vicious cycle of bone metastases,” characteristic of other solid bone tumors, is evident in lung cancer as well.
In terms of the link between preexisting or concomitant osteoporosis and lung cancer, a study by McGlynn et al., one of the largest cancer follow-ups ever conducted among individuals hospitalized with osteoporosis, found that patients younger than 70 years with osteoporosis had an increased risk of NSCLC than those without osteoporosis [110]. A subsequent study confirmed these findings, reporting that a low BMD Z-score was strongly associated with a higher risk of NSCLC [111].
Bone Metastases
About 30–40 % of patients with lung cancer develop bone metastases, with a survival rate between 6–12 months [112]. In the first instance, most patients experience pain, followed by SREs including pathological fractures, spinal cord compression, the need for palliative radiotherapy or surgery to bone, and, less frequently, hypercalcemia. The development of these symptoms has led guideline agencies in the United States and Europe to recommend that bone scans, in the form of a combination of 18F-fluorodeoxyglucose (18F-FDG) positron emission tomography (PET) and computerized tomography (CT), be undertaken at the time of diagnosis [113].
Patients who develop SREs are 20–40 % more likely to die than those without SRE [114], with bone fractures being the most common SRE [112]. A recent Canadian study of 301 patients with metastatic NSCLC reported that 39 % had bone lesions, and 59 % had SREs, with two or more SREs related to smoking and a younger age; fractures accounted for 22 % of the SREs. The overall survival of patients with bone metastases is 5.5 months, compared with 9.9 months for those without bone lesions [115]. Although age, gender, and stage of NSCLC do not appear to influence the occurrence of SREs, a number of factors are associated with their development. Patients with a history of smoking have been found to be 6.7 times more likely to suffer SREs than those who never smoked; patients with poor performance status, less than ≥2, and those with multiple bone metastases were independently 3.3 times as likely to have SREs than those with a performance status of >2 or only a single bone metastasis [116, 117].
Treatment of Bone Metastases
For some time, platinum-based chemotherapies (cisplatin, the most common platinum-based chemotherapy drug, or carboplatin) have been regarded as the standard of care for NSCLC [112]. They are often used in combination with other drugs including third-generation chemotherapy agents (paclitaxel and docetaxel) and tyrosine kinase inhibitors (TKIs) (e.g., gefitinib). However, because the platinum-based components of these combined doses is associated with nephrotoxicity, third-generation agents or TKIs, alone or in combination, are also used, while recognizing that these regimens have lower response rates and are not known to prolong survival rates [116]. Single-agent chemotherapy is preferred in older patients.
As Brodowicz et al. have pointed out [118], unlike the bone loss associated with chemotherapy in breast and prostate cancer, the components of NSCLC therapy may have a beneficial effect on bone resorption. Not only does gefitinib inhibit bone resorption, but clinical trials have shown that a combination regimen of cisplatin, mitomycin C, and vinblastine decreases bone resorption, with patients experiencing less frequent bone metastases [119]. Research to identify less toxic and more effective platinum drugs is ongoing. A study by Park et al. identified phenanthriplatin as 4–40 times more powerful than cisplatin in destroying cancer cells across a range of cancer types and better able to evade cancer cell resistance [120].
Bone-Targeted Agents
In comparison with bone and prostate cancer, data on the use of bone-targeted agents in lung cancer is scarce. In a placebo-controlled, phase III trial of 773 patients with NSCLC, Rosen et al. were among the first to demonstrate the superior efficacy of ZA [121]. They reported that ZA was the first bisphosphonate to reduce SREs in lung cancer: patients taking a 4 mg dose of ZA had a 31 % risk of developing at least one SRE as opposed to 46 % with placebo; moreover, they also experienced a significantly delayed onset of complications as well as a significantly reduced annual incidence of SREs. Not only is ZA effective and safe but its short infusion time makes it more convenient for patient use.
Recent trials have further indicated that ZA may have anticancer effects on lung cancer. As Mahtani et al. have observed, preclinical and clinical studies provide limited evidence about the potential of ZA to exert positive effects on disease progression outside the bone, generally in combination with chemotherapy. But larger, prospective trials are needed to clarify the benefits of ZA therapy and to answer questions such as optimal dosing, initiation, duration of treatment, and the benefits of combination therapy [122].
Within the last decade, a therapy strategy directly targeting RANKL has emerged in the form of denosumab, now approved for the prevention of SREs in patients with solid tumor and bone metastases. The key study in gaining FDA approval was a phase III trial comparing denosumab with ZA in the treatment of advanced cancers and multiple myeloma, excluding breast and prostate cancer [123]. Focusing on a subset of lung cancer patients (both NSCLC and SCLC, n = 81) from that analysis, Scagliotti et al. [124] found that denosumab was associated with significantly improved overall median survival of 8.9 months compared with 7.7 months for ZA, in addition to a statistically significant improvement in SREs and a delayed time to the first SRE of 20.6 months versus 16.3 months for ZA. Moreover, acute-phase reactions and nephrotoxicity occurred less often in the subcutaneous administration of denosumab than with ZA, indicating that despite its high incidence of hypocalcemia, denosumab may be more suitable for combination with platinum-based therapy in NSCLC [116].
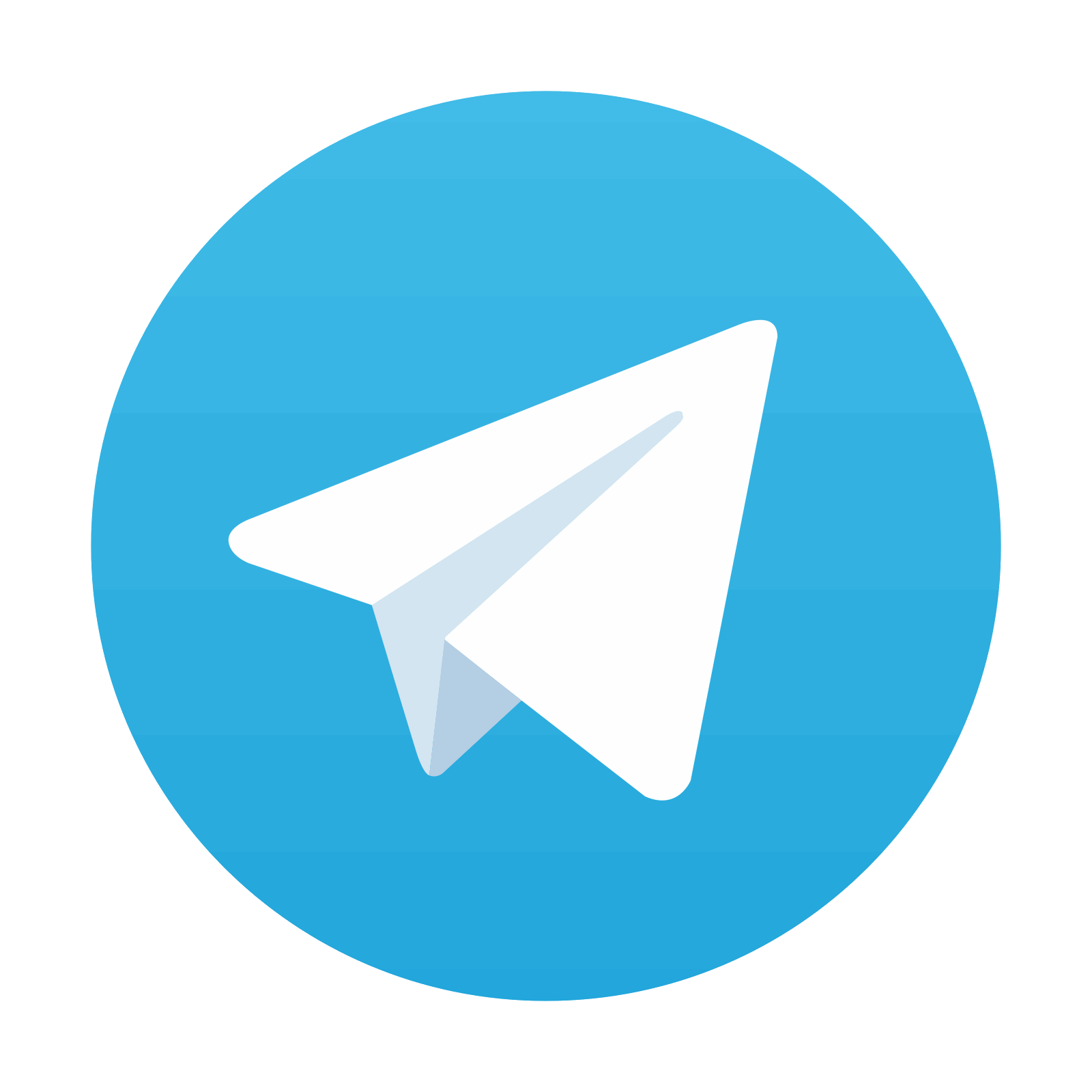
Stay updated, free articles. Join our Telegram channel

Full access? Get Clinical Tree
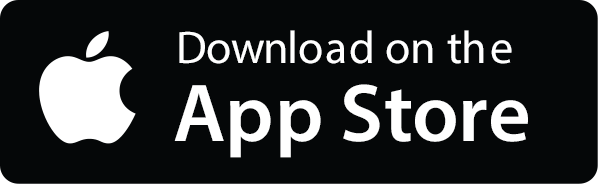
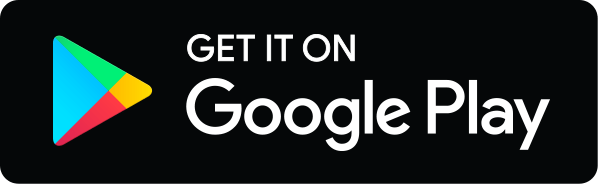