, Ca2+, and hydroxyapatite hydroxide are replaced by carbonate, Mg2, and fluoride respectively. Compared with mineral hydroxyapatite, these imperfect crystals are more soluble and easily dissolved during resorption in the acid environment of the brush border of the osteoclastic cells.
The smallest unit of crystalline structure of the apatites contains 18 ions and it appears probable that such a complex structure is formed de novo from ions in solution. Progression through simpler forms has been demonstrated in vitro. However, these forms are unstable and difficult to demonstrate in vivo. The fluid environments of the body are said to be metastable in terms of their calcium and inorganic phosphate concentration. More precisely, that this concentration is below that of the concentration necessary for spontaneous precipitation but well above the concentration needed for the growth of the crystal if apatite crystals are present in the solution.
This therefore leads us to consider two very different phenomena:
the initiation of mineralization or “nucleation”, the growth of the first crystals formed.
Progression of Mineralization
It has been demonstrated in vivo that more than 90 % of mineralization takes place normally by the growth of pre-existing crystals. As far as the growth of the mineral phase is concerned, the problem here is how to control it. Indeed, once mineralization has started in a metastable environment, it should continue until all the ions are used up. If this were the case, we would all be turned into a pillar of salt like Lot’s wife. Mineral growth is therefore tightly controlled and regulated. Three factors play an important role: collagen, certain non-collagenic proteins, and proteoglycan.
Collagen
Initially considered to assist in nucleation, bone collagen essentially of type I helps in the formation of apatite in vitro and in particular organizes crystallization. The crystals are deposited parallel to the axis of the collagen fibrils and denaturing of the collagen disturbs this precipitation. Therefore, although in vivo studies tend to call into question the role of collagen in nucleation, it has an essential organizing role during the growth of the crystals.
Non-collagenic Proteins
Several non-collagenic proteins have been extracted from different calcified matrices. Two large groups have to be distinguished; the phosphoproteins and the GLA proteins (or proteins carrying gammacarboxyglutamic acid). The phosphoproteins have been isolated from bone, dentine, enamel, and calcified cartilage. Some phosphoproteins are more closely bound to collagen. Various roles have been suggested: orientation of the crystals, the control of their shape and size, or even a support role in particular in tissues which do not contain collagen, such as enamel. Osteonectin, a phosphorylated glycoprotein specific to bone tissue, is thought to help in binding calcium to collagen.
GLA proteins have been suggested as being the agent which regulates mineral growth but their role is still unclear and controversial. Their interest lies particularly in the possibility that a radioimmunological assay could be carried out on the serum, which would be a reliable and sensitive marker of bone remodeling activity.
Proteoglycans
These consist of a central protein of hyaluronic acid and of carbohydrate chains formed from the repetition of sulfated disaccharide units. Essential components of cartilage, proteoglycans have also been isolated from mineralized tissues.
Proteoglycans of bone are thought to be smaller and immunologically specific. It has been suggested that they play a role in calcification on account of the fact that there is a lower level of these in calcified tissues than in non-calcified tissues. Furthermore, in epiphyseal cartilage, the proteoglycans are thought to become smaller and fewer in number close to the calcification front. Moreover, proteoglycan aggregates inhibit the formation of apatite. The idea that proteoglycans indispensable to nucleation are transformed has therefore also been suggested. However Blumenthal has shown that the subunits, like the aggregates, inhibit mineralization. Poole et al., using immunofluorescence techniques, challenge the classical ideas of proteoglycans being reduced during endochondral ossification. In their view proteoglycans continue unchanged when mineralization starts and are only modified during immature primary bone modeling.
Bone Remodeling
Bone resorption and formation take place in a perfectly organized manner. The phenomena are most stereotypical in cortical bone. In old bone, and under influences which are currently little understood but which are certainly biochemical in nature, a population of osteoclasts appears which hollows out a resorption cavity which grows 7–9 microns a day up to a diameter comparable to that of a haversian osteon, and in particular advances into the bone, in a direction determined in particular by the mechanical constraints at a rate of 40–60 microns per day, thus producing a tunnel-like structure. After an intermediate phase (reversal phase), the osteoblasts appear on the walls of the cavity which initially deposit 8–10 lamellae of osteoid tissue and then, owing in particular to the osteoblastic alkaline phosphatases, cause the mineralization of this osteoid. Approximately 10 % of the osteoblasts remain in the bone tissue formed in this way and, when they mature they become osteocytes, reunited with each other and communicating with the cells remaining on the surface of the residual canal by prolongations using a rich and anastomotic canalicular system. The end structure created in this way is the haversian osteon.
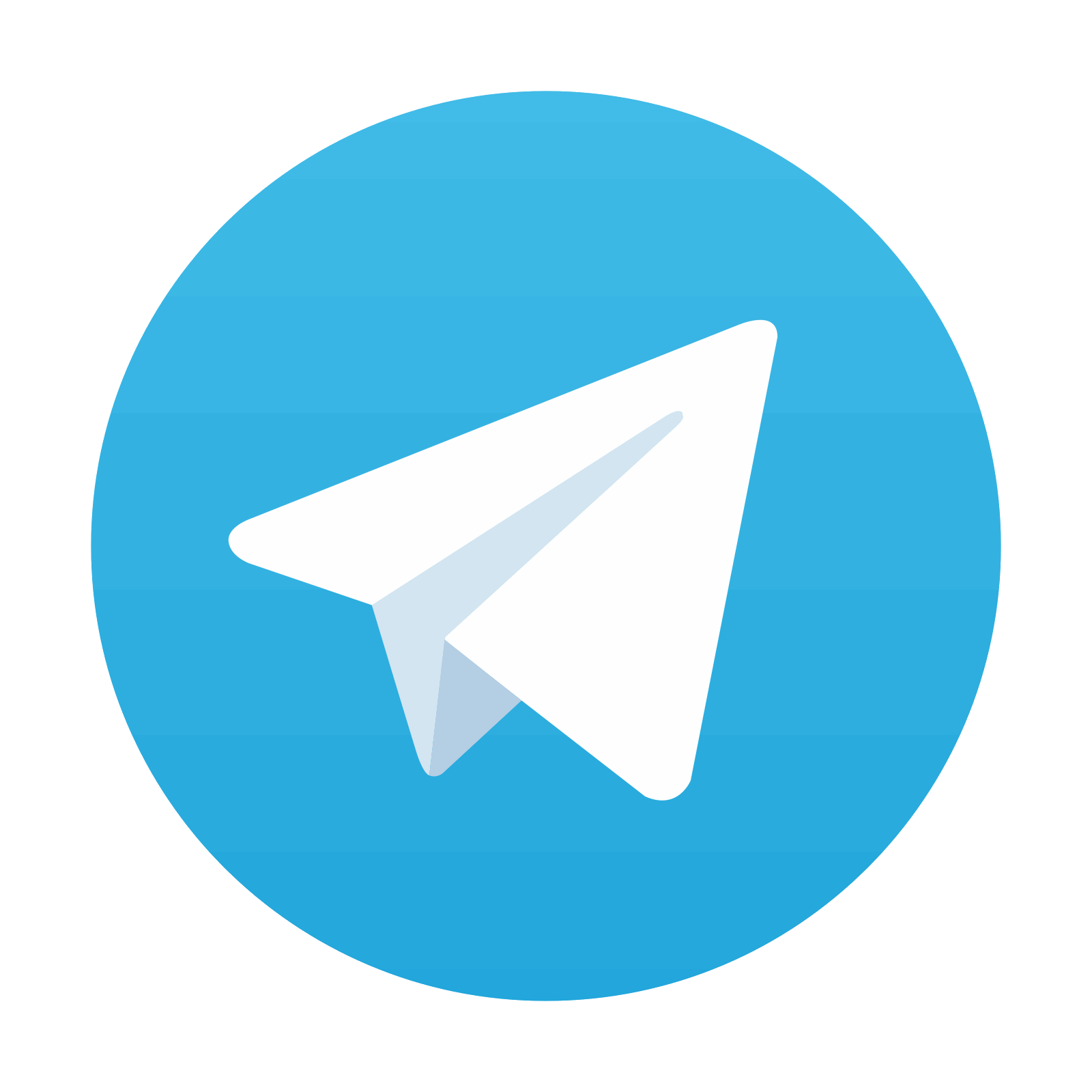
Stay updated, free articles. Join our Telegram channel

Full access? Get Clinical Tree
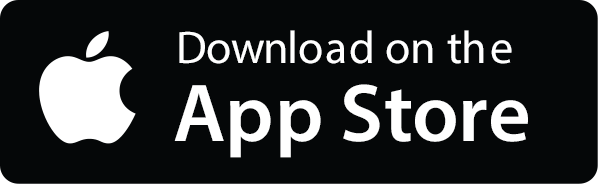
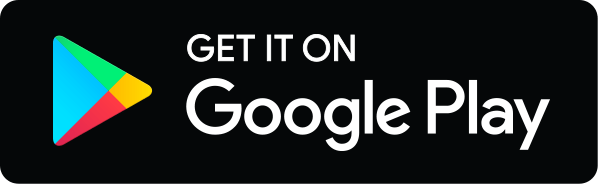