CHAPTER 2
Body Maintenance, Conditioning, Strengthening, and Injury Prevention
Jeff Young, Kinesiologist, CSCS, ACSM-EIM
A close connection exists between proper conditioning and injury prevention (Lauersen, Bertelsen, and Andersen 2014, Vetter and Symonds 2010). The more efficient and better-conditioned athletes are, the less likely it is that an injury will occur, and the more prepared they will be for their sport. Thus, an exercise program must balance the goal of conditioning the body with the goal of preventing injury. Athletes must have a purpose for entering and performing a specific exercise program, but they must also have an appreciation of the essential components of conditioning.
Unfortunately, many athletes take an impractical and poorly planned approach to exercise. They focus on training and pushing their bodies in the hope of reaching a certain result, be it to lose weight or to improve sport-specific speed. But in training this way they overlook the long-term consequences of this type of approach—that is, until an injury occurs.
To reach an ideal balance of conditioning that will allow them to achieve their goals while preventing injury, athletes must learn how to design a program that effectively conditions the body while simultaneously incorporating injury prevention guidelines (Gamble 2006, Lauersen, Bertelsen and Andersen 2014, Vetter and Symonds 2010). Research has shown that an exercise program that includes resistance training, conditioning, explosive training, and skill training, as well as monitoring of the total training load of an athlete, reduces the risk of injury (Baechle and Earle 2008, Davies, Riemann, and Manske 2015, Malone et al. 2018, Wing 2018). To state this more simply, a comprehensive program should merge the principles of strength and conditioning with the principles of sports medicine.
This chapter explains the importance of the initial assessments and how to prescribe the aforementioned components to develop an effective program that prepares the athlete for the rigors of sports participation.
Sport Needs and Individual Assessment
Prior to designing a program, athletes should perform a sport needs assessment as well as an assessment of themselves (Howe, Waldron, and Read 2017). Athletes need to understand the demands of their sport as well as their ability to tolerate those demands. The sport needs assessment includes a movement assessment, a physiological analysis, and an injury analysis. The purpose of the movement assessment is for athletes to determine the primary movements involved in their sport (e.g., explosive movements, rotation, cutting, and deceleration) to ensure that their program includes preparing for and training those movements. The physiological analysis serves to determine which physiological component(s) should be prioritized—strength, power, speed, hypertrophy, or muscular endurance. The purpose of the injury analysis is to gain an understanding of common sites for joint and muscle injuries for the given sport and what causes them (Howe, Waldron, and Read 2017). The injury analysis requires an epidemiology research review; and while this takes a little effort, it is an important component of gathering information to develop an efficient and effective program.
Once the sport itself has been assessed, it is time to perform an individual assessment (Baechle and Earle 2008, Howe, Waldron, and Read 2017). Athletes should understand what athletic components they need to improve upon or emphasize in order to advance their sport performance, such as increasing muscle mass, overall strength, or explosiveness. For example, athletes who are preparing to play a certain position in a team sport (e.g., defensive back in American football) will need to understand where they lie on a continuum regarding their height, weight, body fat, strength, speed, and overall skill level for that position. They should then determine which components they can improve upon (e.g., increasing muscle, reducing body fat, and increasing strength and speed).
A well-designed program will also address an athlete’s musculoskeletal weaknesses or deficits. There are a battery of tests that can be used to seek out these deficits in commonly injured areas such as the ankle, hamstring, knee, low back, and shoulder. Once assessed, issues such as joint hypermobility, movement restrictions, and general weakness can then be corrected as part of the strength and conditioning program.
Furthermore, to decrease the risk of injury, the athlete will need to balance strength around each major joint, in three planes of motion, incorporating both bilateral and unilateral single-joint and multijoint exercises (Baechle and Earle 2008, Schoenfeld and Contreras 2012). Balancing flexibility and mobility around each joint will increase efficiency of movement and rid the body of movement restrictions (Witvrouw, Mahieu, and Danneels 2004). Assessing biomechanics (proper form) and improving deficiencies is a crucial component of injury prevention. Increasing overall conditioning to allow the athlete to perform effectively in a state of fatigue and managing the distribution of training load are other components of injury prevention that will be described in more detail in this chapter.
Flexibility Training
Flexibility, the available range of motion (ROM) around each joint, and mobility, the amount of usable ROM in dynamic, loaded conditions, are important components of both movement and injury prevention (Page 2012, Witvrouw, Mahieu, and Danneels 2004). When restrictions exist because of lack of flexibility or mobility, movement is less efficient and the risk of injury increases. Many soft tissue injuries occur during the eccentric (lowering) phase of a movement while the muscles and tendons are under a load and on stretch (lengthening) (Verall and Dolman 2016). If a joint lacks flexibility, maximal or near-maximal lengthening of the muscles surrounding the joint will occur earlier in a movement. If this happens under a load, injury may occur. At a minimum, flexibility should be balanced around each major joint to reduce this risk (see figure 2.1).
To know objectively if a joint has restricted ROM, falls within the norms, or is hypermobile, an assessment should be performed at each major joint. It would behoove the athlete to have a joint ROM assessment performed by a qualified professional. Once this is accomplished, the athlete can be placed on a proper flexibility training program to balance flexibility around each major joint.
Designing a flexibility program adheres to a principle known as FITT. The letters stand for Frequency, Intensity, Time (duration), and Type.
FIGURE 2.1 The hamstring is a commonly injured muscle group, so stretching exercises such as the supine hamstring stretch are important to help increase range of motion. Also, it is important to perform a joint range of motion assessment, and if the athlete lacks sufficient range, place the athlete on a proper flexibility training program to reduce the risk of muscle strain.
© Human Kinetics
Frequency
A stretching program should be initiated even if the athlete has normal joint ROM at each joint. In that case, the minimum recommendation of twice per week will be enough to maintain joint ROM. Increased tightness, or movement restriction, calls for an increase in volume. This can be accomplished by increasing the frequency, or sets, or both—the tighter an athlete is, the more stretching is needed. Therefore, joints that fall short of normal ROM (i.e., restricted or “tight” areas) should be stretched three to seven days per week (Garber et al. 2011). Stretching must be done properly and safely. Improper stretching has been shown to increase injury.
Intensity
Intensity lies on a subjective continuum of discomfort or pain that ranges from a “gentle” stretch to “mild discomfort,” “moderate discomfort,” “very uncomfortable,” or “painful.” Interestingly, all levels of discomfort, to include stretching to a point of pain (Muanjai, Jones, and Mickevicius 2017), have been shown to increase flexibility. Logically it is not recommended to stretch to the point of pain, and current evidence recommends stretching to the point of mild to moderate discomfort (Garber et al. 2011).
Time
Stretching durations span a continuum that ranges from 10 to 15 seconds up to several minutes in length. When the goal is to increase joint ROM, the total stretch time across multiple sets is most important. Therefore, athletes have the option of holding a stretch for a longer period of time (e.g., 90 seconds) or dividing the total time into multiple sets (e.g., three sets of 30 seconds) (Frietas et al. 2014).
Type
The several types of stretching include active, passive, dynamic, static, isometric, and proprioceptive neuromuscular facilitation (PNF). Dynamic stretching is the preferred mode of stretching as part of a warm-up prior to a workout or event. Among the other types of stretching, PNF has been shown to be superior in the short term, but all types of stretching can improve joint ROM when performed properly (Garber et al. 2011, Guissard and Duchateau 2006, Page 2012).
It is important to note that muscles are viscoelastic (Ryan et al. 2009, 2012). The viscoelastic property of muscle is what allows it to be deformed (stretched); and although its elasticity will also allow it to return back to its original form, through flexibility training joint ROM can increase above baseline values. If the goal is to maintain joint ROM, athletes should stretch until they reach an “end-range feel” for the given stretch (i.e., the feeling that it would be painful if they proceeded further into the stretch), hold, and release. But if the goal is to increase joint ROM, they should stretch until they reach their end range, hold, mentally relax into the stretch, and then increase the stretch to a new end range. This is known as muscle creep, or “creeping further into the stretch,” and the viscoelastic property of muscle, along with relaxation of the nervous system during the stretch, allows for this. The point: If athletes want to increase joint ROM but stretch only to their first end range (never taking advantage of muscle creep and neural relaxation that allows for an increase in ROM), the body is not signaled to progress, and the stretching will be fruitless. It is imperative to remember to find a second, and if possible, a third end range within each set of a stretch when the goal is to improve flexibility.
Self-myofascial release (SMR) is another method of increasing joint ROM, and while SMR will not directly decrease the chance of injury, because it assists in improving ROM and may increase performance as well, it should be included as part of a flexibility training program and warm-up (Beardsley and Skarabot 2015, Schroeder and Best 2015). While it is beyond the scope of this chapter to provide significant detail, SMR follows the FITT protocol and should be used concurrently with stretching, typically just prior to performing various stretches. Please see Cheatham and colleagues (2015) for more information.
Periodization
Periodization is a term that denotes the systematic planning and manipulation of the variables involved in exercise and training program design (Baechle and Earle 2008, Gamble 2006). During the span of a year, a periodized approach is typically broken down into four phases (Baechle and Earle 2008, Gamble 2006).
Off-Season (Base Training)
The priority in off-season training is to improve the athlete’s work capacity and maximize adaptations. The off-season begins with higher volume and low-intensity workouts, and as the off-season continues, intensity is increased and volume is decreased—progressing from strength endurance, to strength, to power. However, the increase in training intensity should not exceed 5 to 10 percent per week.
Preseason
As the athlete begins to transition into the competitive phase, the focus should be on incorporating training that is specific to the physiological profile of the sport and in which sport-specific biomotor abilities (e.g., speed, strength, agility) are perfected. The strengths and weaknesses of the individual athlete should determine the amount and frequency of each type of training.
In-Season (Competition)
The in-season training program needs to be designed to include competition in the training schedule (i.e., the need to monitor total training load). Low-intensity and short-duration training days should precede scheduled competitions so that the athlete is fully recovered and rested prior to play. The types of training employed during the in-season are based on the continued goal of improving weaknesses and maintaining strengths of the athlete.
Postseason (Active Rest)
During the postseason, the primary focus should be on recovering from the previous competitive season. Low training duration and intensity are typical for this active rest phase, but enough overall exercise or activity should be performed to maintain a sufficient level of aerobic fitness, muscular strength, and lean body mass. During the postseason, the athlete should focus on rehabilitating injuries incurred during the competitive season and improving the strength of weak or underconditioned muscle groups.
For more information on periodization, please see Turner (2011) and Gamble (2006).
Aerobic and Metabolic Conditioning
From an aerobic and metabolic standpoint, most sports are intermittent in character, having bursts of fast or explosive movements (e.g., running, sprinting, jumping) followed by periods of rest or reduced intensity (e.g., returning to a huddle in between plays, backpedaling to return to defense). Therefore, in most cases, high-intensity interval training, sprint interval training, or some other form of metabolic conditioning should be included in the athlete’s exercise program (Malone et al. 2018) (see figure 2.2).
Designing aerobic and metabolic conditioning programs also follows the FITT principle.
Frequency
Depending on the time of year, frequency can range from two to as many as six or seven days per week. Higher frequency usually occurs during the preseason when the goal is to increase aerobic capacity and reduce body fat, with lower frequency usually occurring during a competitive season. The frequency of metabolic conditioning normally does not exceed two days per week and needs to coincide with the overall training load, with the understanding that high frequency combined with high intensity increases demand on the central nervous system and leads quickly to burnout (Buchheit and Laursen 2013, Vetter and Symonds 2010, Wing 2018).
FIGURE 2.2 Sprint interval training, which includes work-to-rest ratios that mimic the demands of the sport, is an important conditioning component within the athlete’s overall program.
Pete Sloutos/Getty Images
Intensity
Similar to frequency, intensity can range from low or moderate, which is usually in the form of continuous, rhythmic, steady-state aerobic exercise (e.g., jogging, cycling) at an intensity of 50 to 85 percent of V.O2max, to high-intensity and very high-intensity intermittent exercise. When intensity moves beyond the aforementioned aerobic training zone it is anaerobic, meaning that oxygen is not being consumed at a rate fast enough to handle the workload. This type of training is known as high-intensity interval (HIIT) or sprint interval (SIT) training and includes work-to-rest ratios. Work-to-rest ratios during HIIT are usually 1:1, and work-to-rest ratios during SIT can range from 1:4 to 1:1 (Buchheit and Laursen 2013). More information is provided further on.
Time
When training aerobically using continuous aerobic exercise, duration varies between 20 and 60 minutes per session. When using HIIT as the mode of training, the work portion is normally two to four minutes in length, with the rest portion matching the length of the work portion. For example, following a warm-up of three to five minutes, the athlete would increase the intensity to 85 to 100 percent of V.O2max for three minutes, and then reduce the intensity to 50 percent of V.O2max for three minutes, cycling through each work:rest portion four to six times.
When using SIT as the mode of training, the intensity is such that the work portion can be sustained for only 10 to 30 seconds because it is either an all-out sprint or a near all-out sprint. Because the intensity is so high, in most cases the rest portion needs to be longer (with the exception of Tabata training, which usually has a 1:1 ratio of work to rest). As mentioned earlier, work-to-rest ratios can range from 1:4 to 1:1. For example, following a three- to five-minute warm-up, the athlete would begin an all-out sprint for 15 seconds, followed immediately by decreasing the intensity to either a passive rest (complete stoppage of exercise) or a very light active rest (e.g., slow walk) for 60 seconds. This would equate to a 1:4 work-to-rest ratio. The athlete would cycle through these four to six times, followed by a cool-down.
There are many other ways to perform metabolic conditioning, such as with the use of various types of resistance (e.g., free weights, body weight, bands, machines, hill sprints, and so on), all of which follow the same interval protocol, strategically utilizing work-to-rest ratios to increase the conditioning, aerobic, and work capacity of the athlete. Since many injuries occur under conditions of fatigue, this type of training simultaneously increases overall conditioning while reducing the risk of injury. As it relates to a sport needs assessment, the program design for metabolic conditioning should match the metabolic demands of the sport. For example, in American football a play usually lasts for 5 to 10 seconds, followed by 25 to 30 seconds of rest (i.e., the huddle and setup for the next play). Therefore, metabolic conditioning work-to-rest ratios should closely mimic the demands for this sport.
Type
There are several types and subtypes of aerobic and metabolic training, with the primary types being low to moderate intensity, steady-state, threshold training that occurs at or just below the anaerobic training zone, along with HITT, SIT, and Tabata training.
Resistance Training
Resistance, or strength, training is a crucial component of both injury prevention and performance enhancement. As stated earlier in the chapter, many soft tissue injuries occur during the eccentric phase of a movement while the muscles and tendons are under a load and are being stretched (Verrall and Dolman 2016) (see figure 2.3). From an injury prevention perspective, increasing the strength of muscles and secondary soft tissue (e.g., tendons and ligaments) increases the capacity of the musculoskeletal system to tolerate various, and increasing, forces and loads, thereby significantly decreasing the chance of injury.
Resistance training improves performance via many mechanisms, including an increase in work capacity, efficiency of movement, acceleration, speed, force production, and rate of force development (explosive power) (McGuigan, Wright, and Fleck 2012). While the need for the magnitude of an increase in strength will vary from sport to sport, there is little doubt that athletes benefit from being placed on an effective, periodized program.
FIGURE 2.3 The descending phase of a barbell squat is an example of tissue’s eccentric loading, which is necessary to decrease risk of injury.
MoMo Productions/Stone/Getty Images
Unlike flexibility and aerobic or metabolic training, designing a periodized resistance training program is not as simple as following the FITT protocol. The variables involved in designing a resistance training program include frequency; sets per exercise or muscle group; repetitions per set; rest periods between sets, exercises, and sessions; objective intensity (load lifted); subjective intensity (effort—proximity to momentary muscular failure); choice of exercise; order of exercise; and tempo (Baechle and Earle 2008).
While it is beyond the scope of this chapter to provide a detailed and comprehensive overview of each variable, a brief description is given in the following sections. For more detailed information, the reader should refer to the National Strength and Conditioning Association’s manual (Baechle and Earle 2008).
Frequency
Ideally, each major muscle group should be trained twice per week. During the off-season and preseason, sessions can occur in a split-routine format (e.g., lower body–upper body, or push–pull) approximately four days per week. An example would be a heavy lower body workout on Monday, heavy upper body on Tuesday, off Wednesday (aerobic and flexibility training can be performed), light to moderate lower body on Thursday, light to moderate upper body on Friday, off Saturday and Sunday (aerobic and flexibility training can be performed). The primary goals during this time are to increase strength endurance, lean muscle tissue, and overall strength and power.
During the competitive season, frequency can be reduced to two per week total-body sessions. An example would be a moderate to heavy session on Monday and a light to moderate session on Thursday. The primary goal during this season is to maintain strength endurance, muscle hypertrophy, strength, and power.
During the postseason, frequency of resistance training is once or twice per week, total body, with volume and intensity significantly reduced. The goal is to allow the body to recover without incurring a significant loss in strength fitness.
Sets
During the off-season and preseason, and depending on the athlete’s goals, sets should total no less than 10 per muscle group per week, up to as many as 25 (e.g., approximately 5 to 12 sets per muscle group per session). This is typically done in a split-routine format. During the competitive season, weekly volume should be reduced, for example, to 6 to 12 sets per muscle group (e.g., 3 to 6 sets per muscle group per session). This occurs in the form of two total-body training sessions per week. During the off-season, weekly volume should be further reduced, for example, to two to six sets per muscle group/week, one or two total-body sessions per week.
Repetitions Per Set
During the off-season, while working on increasing overall conditioning and strength endurance, athletes should spend most of their time in the 12- to 20-repetition range. During the preseasons and competitive seasons, athletes should train primarily within the 4- to 12-repetition range. And during the postseason, repetition training ranges should move back up to the 8- to 15-repetition range.
Objective Intensity
Objective intensity refers to the fact that training loads correspond with the repetition range the athlete is working in and assumes that loads are such that each working set is taken to muscle failure or near muscle failure. Correspondences are as follows:
- 1 to 3 repetitions per set corresponds with “very heavy intensity.”
- 3 to 6 repetitions per set corresponds with “heavy intensity.”
- 6 to 12 repetitions corresponds with “moderate intensity.”
- 12 to 15 repetitions corresponds with “light intensity.”
- 15 to 20 repetitions corresponds with “very light intensity.”
Subjective Intensity
Subjective intensity refers to the effort an athlete puts in to each working set, or more specifically, how close each working set is taken to momentary muscular failure. While it is not necessary to train to muscle failure during each working set, nor is it recommended, the athlete does need to put forth an effort (Rodriguez-Rosell et al. 2018) that will cause a training effect to occur. The best way to measure this is with the use of rating of perceived exertion (RPE) scales (Helms et al. 2016, Lauersen, Bertelsen, and Andersen 2014, Naclerio et al. 2011). On an RPE scale of 1 to 10,
- 10 corresponds with muscle failure,
- 9 means the set was terminated with one repetition left in reserve,
- 8 means the set was terminated with two repetitions left in reserve, and
- 7 means the set was terminated with three repetitions left in reserve.
Anything less than a 7 (i.e., 1 to 6 RPE) is considered a warm-up set. The athlete will know when a 7 is reached because the velocity of movement will begin to slow (Gonzalez-Badillo et al. 2017, Sanchez-Medina and Gonzales-Badillo 2011). Therefore, if the velocity of movement of the last repetition within a set is the same as each repetition that preceded it, the set is technically a warm-up. Once the velocity of movement begins to slow, it continues to slow until it eventually stops, despite an attempt to perform another repetition. With this in mind, the velocity of movement at an RPE of 8 is slower than at one of 7; an RPE of 9 is slower than at one of 8; and at an RPE of 10, the movement has stopped because the athlete has reached momentary muscle failure.
The concept of RPE has been thoroughly researched and validated, and it is very important to understand, because it should be used as the athlete’s guide to determine the loads for each exercise and the rate of progression, as well as to distinguish between a warm-up and a working set (Gonzalez-Badillo et al. 2017, Helms et al. 2016, Lauersen, Bertelsen, and Andersen 2014, Naclerio et al. 2011, Rodriguez-Rosell et al. 2018, Sanchez-Medina and Gonzales-Badillo 2011).
Choice of Exercise
The choice of exercise involves two categories: primary and assistance exercises. Primary exercises include squat and deadlift variations, rows, chest press, and shoulder press. Assistance exercises are too numerous to list, but they are used to either increase strength in the primary exercises, or as “correctives” to improve or negate movement dysfunction.
To reduce the risk of injury, athletes should include both multijoint and single-joint exercises in their programs and strengthen the body in each of three planes of motion: sagittal (push, pull, front, back), transverse (rotation), and frontal (side to side and diagonal). If choice of exercise revolves only around multijoint, compound movements, risk of injury may increase because certain musculature is not adequately strengthened unless isolated via single-joint exercises. Examples include the hip abductors, adductors, hamstrings, rectus femoris (the middle of the quadriceps), shoulder external rotators, and low and middle trapezius (Schoenfeld and Contreras 2012).
Order of Exercise
In most cases, the order of exercise should move from most important to least important, complex movement to simple, multijoint to single joint, large muscle group to small, explosive movement to controlled. The way this manifests itself within the scope of a total-body session, for example, is as follows:
Explosive exercises (e.g., Olympic lifts, plyometric exercises) | |
Multijoint leg exercises (e.g., squats, deadlifts, multidirectional lunges) | |
Back exercises (e.g., pull-ups, low rows, bent-over barbell rows) | |
Chest exercises (e.g., flat and incline dumbbell press) | |
Shoulder exercises (e.g., overhead press, external rotation) | |
Single-joint exercises (e.g., reverse flys for low and middle trapezius, adductors, abductors, calves) | |
Exercises for the core can be inserted in between exercises for smaller muscle groups (e.g., shoulders and single-joint exercises), at the end of training sessions, or on off days (or some combination of these). |
Tempo
In most cases, the lifting (concentric muscle shortening) portion of each exercise should be performed with the intent of maximizing velocity, while the lowering (eccentric muscle lengthening) portion of each exercise should be performed with control. If the goal is to emphasize or increase joint stability, the tempo of the lowering portion of each exercise can be increased from one or two seconds to several seconds, and may also include an isometric hold during the transition phase from lift to lower.
Explosive Training
Another very important component of athleticism in most sports is explosiveness, or the ability to increase and maximize the stretch-shortening cycle and therefore the rate of force development. From a training perspective, a baseline or foundation of strength fitness should be established first, and then the training program must include explosive exercises. This is normally accomplished with the use of Olympic lift variations (i.e., cleans, snatches, jerks) or plyometric exercises (e.g., jumps, bounds, hops, box drills, explosive medicine ball throws) or both (Booth and Orr 2016, Davies, Riemann, and Manske 2015, Haff and Nimphius 2012, Janz, Dietz, and Malone 2008, Stone et al. 2006).
Along with improving performance, plyometric training may play an important role in injury prevention. For example, a growing amount of research shows that plyometric training may help prevent first-time noncontact anterior cruciate ligament (ACL) injuries as well as reduce the risk of ankle sprains (Davies, Riemann, and Manske 2015) (see figure 2.4).
It is important to understand that explosive training is different from resistance training, where the athlete wants to look for a decrease in velocity of movement during each working set (i.e., ensure that neuromuscular fatigue has set in). In order to improve explosiveness, the athlete wants to avoid neuromuscular fatigue during working sets. This means that when fatigue is setting in and velocity of movement begins to slow, sets or sessions (or both) should be terminated. Therefore, both the volume and the intensity are normally kept low (Stone et al. 2006).
FIGURE 2.4 Plyometric exercises can reduce the risk of injury by increasing joint and secondary soft tissue’s ability to absorb ground reaction forces and by increasing their capacity to tolerate loads, especially while tissues are on stretch.
Phoenixns/iStock/Getty Images
Distribution of Training Load
Through accurately monitoring the distribution of training load, athletic performance can be optimized while reducing the risk of injury, and excessive levels of fatigue are also reduced. Athlete load is defined as the physiological work performed by an athlete during both competition and training and the subsequent impact and stress of this load on the athlete. This includes the movement demands of competition, the sum total of training interventions performed by the athlete, and their effect on athlete fatigue and mood status. Athlete load can be divided into two categories—internal and external. External load is the amount of work completed by an athlete during any phase of training and competition. This may include work completed during competition, during resistance training programs and recovery sessions, and if applicable, during the working day (Wing 2018).
Internal training load refers to the physiological or psychological stress experienced by the athlete in response to the external training load. The internal load is commonly measured through self-reporting but can also be measured via physiological and biochemical analysis.
The following are four common methods of collecting athlete load information:
- Session RPE. This is the athlete’s self-rating of each training session on a 1 to 10 perceived exertion scale.
- Global positioning systems (GPS). These devices are becoming more common, and can be used to monitor total distance, maximum velocity, distance at various speeds, work-to-rest ratios, and acceleration and deceleration. This information can then be used to monitor volume and intensity of playing or training time to better understand the demands of the sport. The information can also be used to increase the efficiency and effectiveness of training sessions by devising drills that mimic match or game play or specific periods within it.
- Heart rate-based training impulse (TRIMP). This is a method of monitoring the time spent within five heart rate training zones: 50 to 60, 60 to 70, 70 to 80, 80 to 90, and 90 to 100 percent of maximum heart rate. This information is then used to determine how much time was spent above or below lactate threshold to give a clearer idea of volume and intensity of sessions and play. This method can be used in conjunction with or as an alternative to GPS monitoring.
- Wellness questionnaires. These are another method of monitoring internal athlete load in which athletes score their perceived level of well-being across several parameters. These may include sleep (duration and quality), feelings of fatigue, muscle soreness, energy levels, and amount of psychological stress. These scores are then added together to give a total wellness score for the athlete.
- Heart rate-based training impulse (TRIMP). This is a method of monitoring the time spent within five heart rate training zones: 50 to 60, 60 to 70, 70 to 80, 80 to 90, and 90 to 100 percent of maximum heart rate. This information is then used to determine how much time was spent above or below lactate threshold to give a clearer idea of volume and intensity of sessions and play. This method can be used in conjunction with or as an alternative to GPS monitoring.
By monitoring external and internal training load, both the dose (external) and the athlete’s response (internal) can be measured, and when necessary, strategic adjustments can be made within the training program (e.g., a decrease in frequency, volume, or intensity). This is important because subjecting an athlete to inappropriately increased loads can lead to a decrease in performance and also increase the risk of injury and illness.
For further reading on this subject, refer to Wing (2018).
Warm-Up and Cool-Down
Warming up prior to any workout improves performance by increasing blood flow, warming the muscles, and preventing rapid alterations in body physiology that might occur if an athlete simply started participating at full speed. For any sport or activity, a warm-up should include 5 to 10 minutes of slow jogging or other activity to increase body temperature, followed by 10 to 15 minutes of sport-specific drills (Bishop 2003, Fradkin et al. 2010).
Many experts also advocate 10 to 15 minutes of stretching to reduce muscle stiffness before activity. Those who recommend stretching at this point in a warm-up assert that muscle stiffness is directly related to muscle injury and that stretching should be included in all warm-up routines. If stretching is used as part of a warm-up, it should focus on dynamic stretches that decrease muscle stiffness (see figure 2.5). An example of a dynamic stretch is 8 to 12 repetitions of controlled leg swings, arm swings, or torso twists. Do not confuse dynamic stretching with ballistic stretching, which involves forcing a part of the body beyond its natural ROM. There are no such movements in dynamic stretching.
After a workout or game, a cool-down helps dissipate metabolic waste products (such as lactic acid) from muscle, reduces the potential for muscle soreness, and reduces the chance of dizziness or fainting caused by pooling of venous blood in the extremities. Cooling down should include several minutes of low-intensity aerobic exercise followed by 5 to 10 minutes of static stretching exercises. Static stretches help muscles relax and improve their range of movement. Generally, a static stretch is held for 30 to 60 seconds with continuous tension on the target muscle. Keep in mind that static stretches are best as a cool-down; they do not prepare muscles for activities as well as dynamic stretching and may impair performance if performed prior to an event.
FIGURE 2.5 Dynamic warm-up exercises are an important way to increase blood flow, and stimulate both joint lubrication and neural excitation, which in turn decrease stiffness and reduce the chance of injury.
Hero Images/Getty Images
Using Proper Technique and Equipment
Biomechanics, the study of internal and external forces that affect the body, plays a crucial role in efficient and safe participation in any activity. Faulty biomechanics result either from static anatomical abnormalities or from movement dysfunction. Although static abnormalities can be addressed by compensatory devices such as orthotics, the functional changes that result from both the abnormality and its correction must be addressed in training. Movement dysfunctions are usually easier to change because they are often a result of injury, improper technique, or poorly adjusted equipment.
Two issues should be addressed regarding equipment. The first is a proper fit. Ill-fitting equipment negatively affects biomechanics. The second is protection. Wearing or using proper protective equipment during training and competition significantly reduces the risk of injury.
The sport of cycling illustrates the role of properly fitted equipment in promoting good biomechanics. The bike minimizes the negative effects of both the repetitive motion of cycling and the static position that the body maintains. When seated on a bike with hands on the handlebars, the hands, shoulders, and front axle should all be in line, and the reach should be set so that the rider has slight elbow flexion with hands relaxed on the brake hoods. This allows the rider to hold the wrist in a neutral position. If alignment is not correct and the wrist bears weight in an extended position, the rider might damage the ulnar nerve (the nerve that runs from the upper arm to the pinky side of the hand). This damage can be prevented if the build of the bike is fitted to the athlete. Specification of the bike to the rider also ensures that seat placement is correct. Seat height is critical to proper pedaling biomechanics. If a seat is too high, muscles must work beyond their optimal length–tension range. If a seat is too low, knee flexion is increased as is stress on the knee joints.
Proper footwear has a similar effect on biomechanics. Using our cycling example, shoes need to be both comfortable and rigid to transfer power from the pedal to the leg. If the transfer is inefficient, strain on the lower limb and lumbar spine is increased. In general, footwear should support the foot, absorb impact, and provide traction. The optimal shoe for an athlete is one that matches the individual’s biomechanical features and answers the demands of the sport. When appropriate, a simple foot orthotic can correct anatomical abnormalities.
The use of protective equipment is usually recommended as a result of research by health professionals that identifies a high risk of injury in a particular sport or recreational activity. Protective equipment includes personal equipment such as mouth guards and headgear as well as external equipment such as padding around the goalposts on an American football field. Personal equipment must be used for its intended purpose, fit well, be comfortable, allow unrestricted movement, and be worn or used whether the athlete is at practice or in competition. It should be replaced when worn out or damaged and must comply with the rules of the sport for which it is intended. Protective equipment should not be shared among players of different sizes and should be appropriate to the player’s sex, covering areas most likely to encounter other players or equipment.
Helmets have proven effective in preventing or reducing the severity of brain injuries in sport. Sport-specific helmets are designed to address the different risk factors inherent to each sport. Risks vary from sport to sport because of distance to the ground, playing surface, playing equipment, and speed of movement. In any case, the helmet should be firm, comfortable, and fitted to the athlete. A loose-fitting helmet might obstruct view or cause hyperextension of the cervical spine. Whereas hard helmets reduce the risk of head injury, soft headgear can help prevent serious abrasions to the scalp and ears. Helmets are either mandated or recommended for auto and motor sports, bicycling, boxing, equestrian, football, hockey, lacrosse, in-line skating, rugby, skateboarding, skiing, snowboarding, softball, and wrestling.
Other protective gear includes eyewear and mouth guards. Protective eyewear standards currently exist for racket sports, women’s lacrosse, paintball, and youth baseball. The Protective Eyewear Certification Council (PECC) assists consumers, sports organizations, and eye care professionals in choosing proper eyewear. The PECC seal ensures that equipment has been tested and certified to protect the eye from damage. Mouth protectors help prevent injury to the mouth, teeth, lips, cheeks, and tongue. They can cushion blows that might otherwise cause concussion or jaw fracture. Mouth guards should be worn by all athletes during contact and collision sports.
A concern related to protective equipment is the safety of the playing surface on which a sport is played. A hard surface generates more force to the musculoskeletal system than a soft surface. Additionally, traction plays a key role in the risk of injury. For example, in the case of American football, it has been found that dry fields increase the risk of ACL injury because of the large amount of traction and the resulting forces transmitted to the knee during rapid movement and change of direction. Watering down fields to soften them prior to play could reduce the risk of such injuries. Similarly, placing padding around goalposts to absorb impact and minimize trauma could reduce the severity of some types of injury.
Nutrition Considerations
In March 2016 the American Dietetic Association, Dietitians of Canada, and the American College of Sports Medicine issued a joint position statement on nutrition and performance. This paper covers new perspectives in sports nutrition, and the reader is directed to the paper for more detail.
Key points from the evidence presented in the paper include consuming adequate calories from each of the three macronutrients (protein, fats, carbohydrates); determining optimal intake of carbohydrates based on their sport and periodizing appropriately; meeting at least the minimum USRDA recommendations for vitamin and mineral intake; and remaining cognizant of the importance of pre- and post-competition meals and hydration.
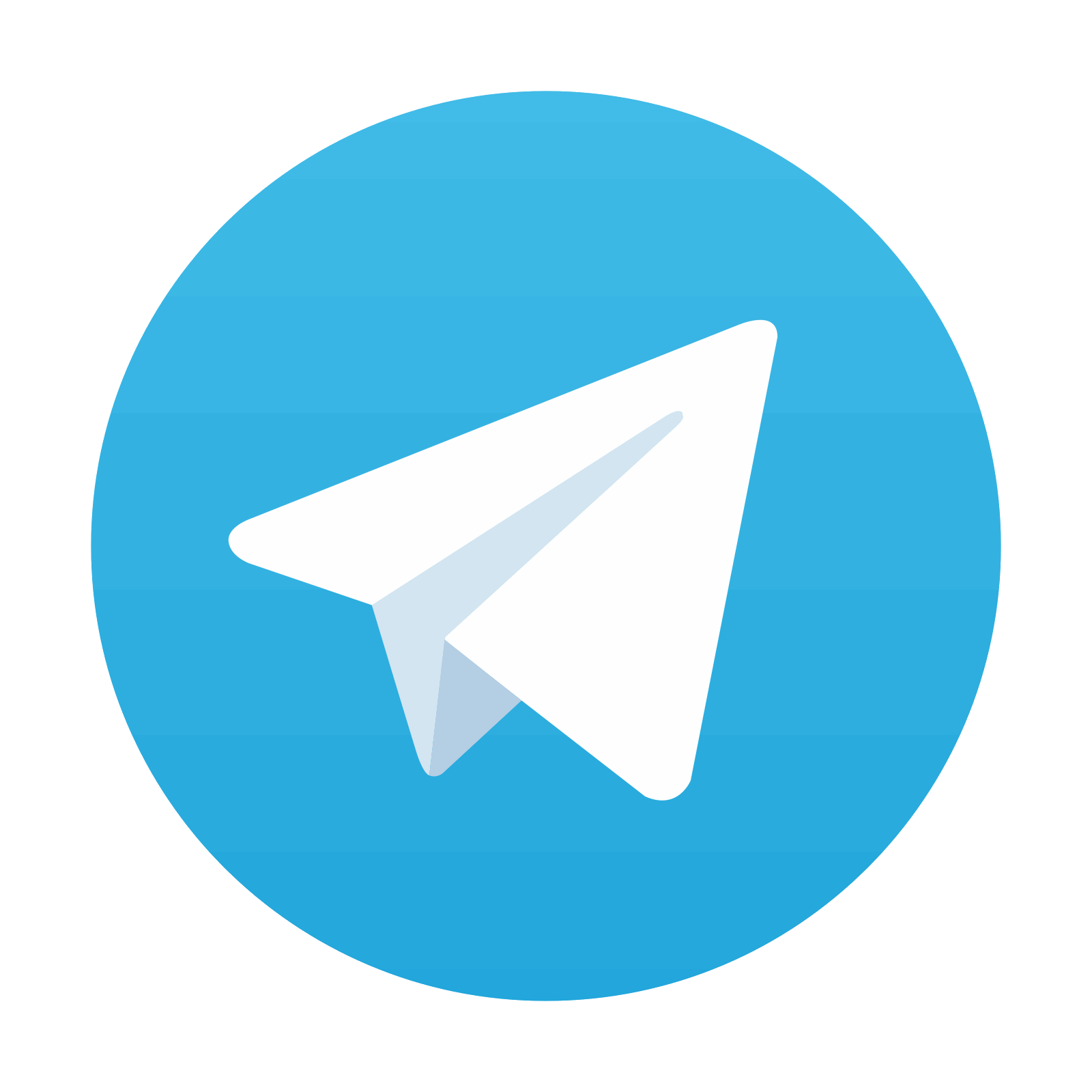
Stay updated, free articles. Join our Telegram channel

Full access? Get Clinical Tree
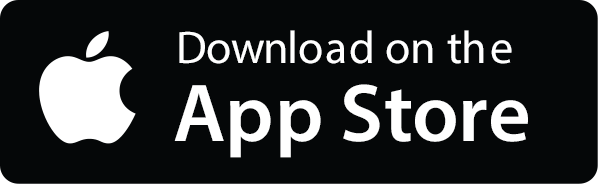
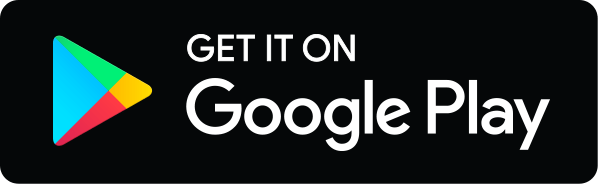