For additional ancillary materials related to this chapter, please visit thePoint.
Body composition describes the amount and relative proportions of fat mass (FM) and fat-free mass (FFM) in the human body. Once considered as only descriptive, the study of body composition is evolving into functional body composition as evidence emerges that there is “cross-talk” between body components acting as regulatory systems that affects body functions and metabolic processes via hormones, cytokines, and metabolites (5,43).
Measurement of body composition is a standard component of testing and evaluation for exercise professionals (28,59). Valuable information regarding percent body fat (PBF), fat distribution, body segment girth, and bone density may be gained through body composition assessment and utilized for reducing health risks associated with disease (63) and for designing safe and effective training programs that optimize athletic performance (39). This chapter discusses body composition assessment and compares commonly used measurement techniques.
![]() |
The assessment of body composition in children and adolescent (70), adult, older adults (15), special populations such as pregnant (76) and postmenopausal women (32), and individuals with intellectual disability (10) has many benefits. PBF estimation provides vital information concerning health and fitness. An excess amount of body fat, or obesity, is linked to several diseases including Type 2 diabetes mellitus, hypertension, hyperlipidemia, cardiovascular disease (CVD) (44), associated increased morbidity and mortality from CVD (69), and certain types of cancer (6). In addition, increased PBF has been linked to increased risk of musculoskeletal symptoms due to increased mechanical stress (71) and to osteoarthritis possibly due to proinflammatory mediators activated by an increased level of adipokines secreted by adipose tissue (54). Because of the current epidemic of obesity in adults 18 years and older (~35%), in children and adolescents (~17%) in the United States (47), and on a global scale more than half a billion adults (46,77), detection of obesity is of primary importance for health and exercise science professionals (43,55). Assessment of body composition also plays a critical role in determining if interventions such as exercise may be effective in modifying disease risks associated with accumulation of excess adipose tissue without requiring significant weight loss (55).
Body composition assessment is useful for those individuals with a low, as well as high, PBF. During times of malnutrition (e.g., eating disorders) and in some weight-sensitive sports, body fat levels and water content can fall to dangerously low levels (1,39). Meyer et al. (39) state that weight-sensitive sports “are those in which extreme dieting, low PBF, frequent mass fluctuation and eating disorders have been reported in both literature and practice” (p. 1046). These include gravitational sports (e.g., long-distance running, road cycling, high jumping, pole vaulting), weight class sports (e.g., wrestling, judo boxing, weightlifting), and aesthetic sports (e.g., figure skating, rhythmic and artistic gymnastics, and bodybuilding) (39). Such sports require athletes to compete at either low weight or with minimal body fat. Measurement of FFM and bone mineral density (BMD) has several important ramifications. In the clinical setting, assessment of FFM and BMD can be used to assess the effects of aging (15) and disease (4,18,63). A progressive reduction in BMD, that is, osteopenia and osteoporosis, may occur with aging, physical inactivity, and overtraining. In addition, sarcopenia, the degenerative loss of skeletal muscle mass and strength as a result of aging, reduced physical activity, and certain diseases, can be assessed (43,51). Sarcopenia reduces the ability to perform activities of daily living (ADL); alters metabolism, muscle, and bone function; and increases the risk of musculoskeletal injury. Thus, body composition assessment in the clinical setting can be used to monitor the progression of disease or muscle/bone enhancement as a result of therapeutic or pharmaceutical intervention (43,63).
Also, assessment of body composition may assist in the diagnosis of diseases obscured in individuals with a normal weight but a high PBF and decreased FFM (e.g., sarcopenic obesity) and/or decreased BMD (43). In hospitalized patients, nutritional status is assessed on a regular basis. Body composition assists in documenting the efficiency of nutritional support (63). Assessment of body composition is also an essential tool in helping manage the treatment of cancer survivors who frequently experience extremely altered body composition such as muscle wasting, a central feature of cancer cachexia (4,18,43,63).
Furthermore, in apparently healthy individuals, assessment of body composition can be used to quantify changes in FM, FFM, and BMD as a result of weight training and weight loss and weight gain (25,52). These measurements may be used for individualization of exercise prescriptions and for the evaluation of an exercise conditioning program. In the athletic population, body composition assessment can be used to quantify changes in FFM and BMD as a result of physical training and aid in distinguishing healthy from unhealthy weight loss and weight gain (1,39). In this regard, overtraining combined with low energy availability in both women (31) and men (62) can negatively affect the athlete’s body composition and health acutely and over the long term. The International Olympic Committee’s consensus statement on relative energy deficiency in sport (RED-S) addresses the complexities involved as they pertain to both female and male athletes (42). In addition, concern has been expressed that the focus on body mass and composition in sport may have unintended negative effects on the athlete (e.g., body image issues, disordered eating/eating disorders, dehydration) (39).
![]() |
Body composition methods can be categorized as being direct, indirect, or doubly indirect (1,59,60). The direct method initially (~1945) employed cadaver dissection and the subsequent chemical analysis in vitro (13). More modern approaches at the direct method use in vivo methods such as magnetic resonance imaging (MRI), computed tomography (CT), and neutron activation analysis (13,22). Indirect methods measure one parameter to estimate another (1). For instance, hydrostatic weighing (HW) is used to measure body volume and then PBF is estimated based on assumed values for the density of FM and FFM (1,13,28). Doubly indirect methods (e.g., skinfolds), which uses one indirect measure to predict another indirect measure, were primarily derived from the indirect method, HW. Due to technological advances over the past two decades, equations to convert skinfold thickness to PBF are being derived from methods that directly measure values of body composition in vivo and thus are theoretically more accurate (22).
Different models have been proposed for characterizing human body composition by discrete compartments with the sum totaling the individual’s body mass. The two-compartment (2C) model partitions body mass into FM and FFM (1,22,28) and has the widest application to body composition analysis. This model is limited by the assumptions that water and mineral contents of the body remain constant throughout life and between all individuals and that the density of FFM is constant among all individuals. However, FFM composition is known to be altered by age, sex, pregnancy, weight loss/gain, race/ethnic differences (22,52), some states of disease (e.g., osteoporosis, sarcopenia), and in highly trained athletes (22,40).
Multicompartment models have been developed because of violation of the inherent assumptions of the 2C model. These models divide the body into more than two compartments and require fewer assumptions about the composition of the FFM. Thus, multicompartment models provide more accurate results (1,22,24,28,59,63). The 3C model includes FM but also partitions FFM into total body water (TBW) and dry FFM. The 4C model partitions body mass into FM, TBW, BMD, and the residual dry FFM. Thus, the addition of BMD (via dual-energy x-ray absorptiometry [DXA]) is added to the 3C model. In addition, 4C models often serve as the criterion model for body composition measurement in validation studies (1,22,52). The measurement of soft tissue mineral content (via in vivo neutron analysis or by using a prediction equation based on neutron analysis) has been added to 4C models to generate a criterion 5C model. The choice of an appropriate model depends on the component of FFM that is expected to vary the most from population norms.
![]() |
Direct in vivo methods are used on a limited basis due to the paucity of specially trained personnel, high costs associated with instrumentation, and, in general, lack of portability. Therefore, most body composition measurements involve indirect assessment or estimation. The decision on which method to use depends on several factors, including the needs of the individual, purpose of the evaluation, cost of the measurements or equipment needed, availability of each assessment tool, and the technician’s expertise (1,22,24,28,37,57,59,65). In regard to the latter, body composition assessment certification for anthropometric techniques only exists from the International Society for the Advancement of Kinanthropometry (ISAK) (38,39,59). The following is a brief summary of basic and selected advanced methods to assess body composition.
Height, Body Mass, and Body Mass Index
Anthropometry is the measurement of the human body using simple physical techniques. Height should be assessed with a stadiometer (a vertical ruler mounted on a wall with a wide horizontal headboard). Standard procedures for height measurements include no shoes, heels together, erect posture, head level looking straight ahead, after a deep inhalation, and holding breath. Height can vary slightly throughout the day with variations in fluid content of the spine’s intervertebral discs and is affected by activity level (upright, weight-bearing exercise increases spinal disc compression, which can slightly reduce height). Height is typically greatest in the morning (when intervertebral disc fluid content is highest), so selecting a standard time and monitoring preactivity level increases reliability when multiple measures are taken from the same individual over time (38,59).
Body mass is best measured on a calibrated scale. Ideally, measurements should be completed with minimal clothing. Facilities should adopt the most reasonable clothing policy for their population (60). Body mass can change at various times of day because of meal/beverage consumption, urination, defecation, and potential dehydration/water loss. Thus, a standard time (e.g., early in the morning) relative to exercise and nutritional intake will increase the consistency of measurements (38,59).
Body mass index (BMI) is used to assess an individual’s mass relative to height (BMI = body mass / height squared; kg ∙ m−2). The primary advantages of BMI are that it is a relatively easy measure to obtain and it is useful for categorizing the extent of overweight and obesity in large populations (30,49,70). In this regard, the American College of Cardiology and the American Heart Association’s classification of overweight and obesity is based on BMI (29). Adult BMI is a reasonably generalizable height-independent measure of body shape and composition for comparisons across sex and race/ethnic groups (27). In a prospective study of approximately 1 million African Americans and White men and women, the risk of premature mortality was higher in those classified as underweight and overweight/obese categories (3, Table 4.1) when compared with the lower end of normal weight (50). Recently, a 15-year longitudinal study with approximately 30,000 participants found BMI to be significantly better than PBF in predicting mortality from CVD (49). The data from this study also suggest that “excess body weight is a stronger predictor of CVD than is an excess of body fat” (49). One should be cautious when using BMI as the sole indicator of cardiometabolic health, a practice some employers have selected to determine employee health insurance costs. An analysis of National Health and Nutrition Examination Survey (NHANES) data from 2005 to 2012 determined that 29% of individuals with obesity based on BMI were metabolically healthy, whereas 30% of normal weight individuals classified by BMI were cardiometabolically unhealthy (64). The former finding is supported by an extensive review of the metabolically healthy but obese (MHO) phenotype (48). The review reported that the percentage of adults in the United States and Europe combined that are obese (BMI ≥30) and meet 0 of the metabolic syndrome (MetS) criteria is estimated to be ~12%–17% (48).
Recent findings indicate that the association between all-cause mortality and BMI may be affected by race. For instance, BMI and mortality are strongly related among White Americans but not very robust in African Americans (34). However, African Americans’ mortality risk increased with an elevated waist circumference (WC) compared with those with a low WC (34).
BMI is a relatively poor predictor of PBF (2). Additionally, BMI may result in inaccurate classifications (normal, overweight, obese) for some individuals particularly those who are muscular (51). This shortcoming is because BMI presumes that excessive weight is solely due to increased adiposity (38). However, misclassification of body weight is not prevalent in nonathletic adult populations who are weight stable or who have gained weight in their adult years. Thus, BMI should provide a reasonably accurate classification of weight status in these individuals (38). Finally, BMI can be used for estimating ideal body weight and body weight (49): Wt (lb) = [5 ∙ BMI + (BMI ∙ 5−1)] ∙ (Ht − 60 in). For application of the equation to weight loss management and for the metric version of the equation, see Peterson et al. (51).
Circumferences, or girths, are used to estimate body composition and provide specific reference to the distribution of fat in the body. The pattern of body fat distribution is an important predictor of the health risks associated with obesity. Increased fat distribution on the trunk (android obesity) increases the risk of hypertension, MetS, Type 2 diabetes, dyslipidemia, coronary artery disease, and premature death when compared with individuals whose fat is distributed in the hip and thigh region (gynoid obesity) (16,17).
Using circumference measures as a means for estimating body composition has the advantage of being easy to learn, quick to complete, and inexpensive. Various translational equations are available for men and women to convert girth measurements to body fat estimations across a range of ages and percentage of body fat (22,65,66,75). Accuracy of circumference measures vary but can range within 2.5%–4.0% of the body composition derived from hydrostatic densitometry. Circumferences also provide information about growth and frame size (24,28). Briefly, measurement technique consists of using an inelastic tape measure placed in a horizontal plane or perpendicular to the length of the segment being measured. Tension on the tape should be snug but not so tight as to compress the subcutaneous fat layer (24,28,59,65). Duplicate measurements are obtained in a rotational order instead of consecutively (3, Box 4.1) (24,28).
The WC has been advocated for use as a “vital sign” in clinical practice because research has demonstrated that the distribution of body fat is more important in determining CVD risk than excess total body fat (17). In this regard, WC represents subcutaneous adipose tissue (SAT) as well as visceral adipose tissue (VAT). VAT is closely associated with increased cardiometabolic risk, although the exact mechanism(s) have yet to be fully elucidated (16). As such, the relationship should not be considered a cause-and-effect one (16). Furthermore, racial differences exist in abdominal depot-specific body fatness with African American men and women having lower amounts of VAT for a given amount of total body fat than White Americans (9,33). Thus, WC alone as a simple marker of visceral adiposity is limited (16). However, WC alone has been used an indicator of CVD risk (3, Table 4.2) (7,29). Ethnic- or country-specific WC cutoff points for risk of metabolic complications are available from the World Health Organization (WHO) and the International Diabetes Federation (77). It is more effective to use the WC and the BMI together as factors indicative of cardiometabolic risk (Table 6.1) (3, Table 4.1) (17,29) as well as mortality risk (11,56). Additionally, WC and BMI together may also be used in public health to assist an individual in determining his or her PBF by a simple charting method (35).
Cardiometabolic Risk Score Values across Tertiles of Waist Circumference within Each of the Three Body Mass Index Categoriesa |
Men | WC Tertiles | |||||
T1 | T2 | T3 | ||||
BMI <25 kg ∙ m−2 | WC ≤84 cm | 2.1 ± 0.1 | 84 cm < WC ≤ 90 cm | 2.5 ± 0.1** | WC >90 cm | 2.7 ± 0.1*** |
25 kg ∙ m−2 ≤ BMI < 30 kg ∙ m−2 | WC ≤95 cm | 2.7 ± 0.1 | 95 cm < WC ≤ 101 cm | 3.3 ± 0.1** | WC >101 cm | 3.6 ± 0.1*** |
BMI ≥30 kg ∙ m−2 | WC ≤108 cm | 3.7 ± 0.1 | 108 cm < WC ≤ 116 cm | 4.1 ± 0.1* | WC >116 cm | 4.5 ± 0.1**† |
Women | WC Tertiles | |||||
T1 | T2 | T3 | ||||
BMI <25 kg ∙ m−2 | WC ≤76 cm | 1.5 ± 0.1 | 76 cm < WC ≤ 83 cm | 1.9 ± 0.1** | WC >83 cm | 2.7 ± 0.1***††† |
25 kg ∙ m−2 ≤ BMI < 30 kg ∙ m−2 | WC ≤87 cm | 2.5 ± 0.1 | 87 cm < WC ≤ 93 cm | 3.3 ± 0.1*** | WC >93 cm | 3.8 ± 0.1***†† |
BMI ≥30 kg ∙ m−2 | WC ≤100 cm | 3.4 ± 0.1 | 100 < WC ≤ 108 cm | 3.8 ± 0.1 | WC >108 cm | 4.6 ± 0.1**†† |
*p < .05.**p < .01.***p < .0001 denote significantly different from the first WC tertile group within the same BMI category, and †p < .05. ††p <0.01. †††p < .0001 denote significantly different from the middle WC tertile group within the same BMI category. T1, T2, and T3 are the WC tertile groups. All statistical analyses were adjusted for age, ethnicity, physician’s specialty, smoking status, and educational level.
aData are given for men and women separately, as means ± standardized error of the means (SEMs).
Source: Nazare JA, Smith J, Borel AL, et al. Usefulness of measuring both body mass index and waist circumference for the estimation of visceral adiposity and related cardiometabolic risk profile (from the INSPIRE ME IAA study). Am J Cardiol. 2015;115:307–15.
The exact site used for measurement of the WC has been reported to vary considerably (52) with one study finding 14 different descriptions of the WC measurement sites in the literature (72). Wang et al. (72) compared WC measurements at four commonly used sites (Table 6.2) with body fat measured in 74 subjects using DXA. They found that the four WC measurements were not all similar in value because the narrowest waist was significantly less than the WC values at the other three sites. However, they found that WC values measured at the four sites are almost equally associated with total body fat and trunk fat in both females and males (72). Ross et al. (53) convened a panel of experts to systematically review the WC measurement protocol in 120 studies. They determined that WC measurement protocol had no substantial influence on the association of WC with all-cause and CVD mortality, CVD, and diabetes. Based on practical considerations for reliable measurement of WC, the expert panel recommended using either the WHO guidelines or the National Institutes of Health (NIH) guidelines (see Table 6.2). Of the two protocols, the panel singled out the NIH protocol for adoption. The NIH method uses an easily located landmark, the superior border of the iliac crest, to aid in accurately locating the waist and thus eliminates potential errors (53). Interestingly, Wang et al.’s (72) study found the NIH WC site to have the highest correlation with percentage of body fat. These investigators also noted that the NIH WC site is close to L4–L5 which is where MRI and CT single-slice measurements are performed to measure VAT (72).
The Four Most Commonly Measured Waist Circumference Sites Defined by Specific Anatomic Landmarks |
Measurement Sites | Comment |
Immediately below the lowest rib | |
At the narrowest waist | ASM sitea |
Midpoint between the lowest rib and the iliac crest | WHO siteb |
Immediately above the iliac crest | NIH and NHANES III sitec |
aRecommended in the Anthropometric Standardization Reference Manual (ASM).
bRecommended in the WHO guidelines.
cRecommended in the NIH guidelines and applied in the third National Health and Nutrition Examination Survey (NHANES III).
Source: Wang J, Thornton JC, Bari S, et al. Comparisons of waist circumferences measured at 4 sites. Am J Clin Nutr. 2003;77:379–84.
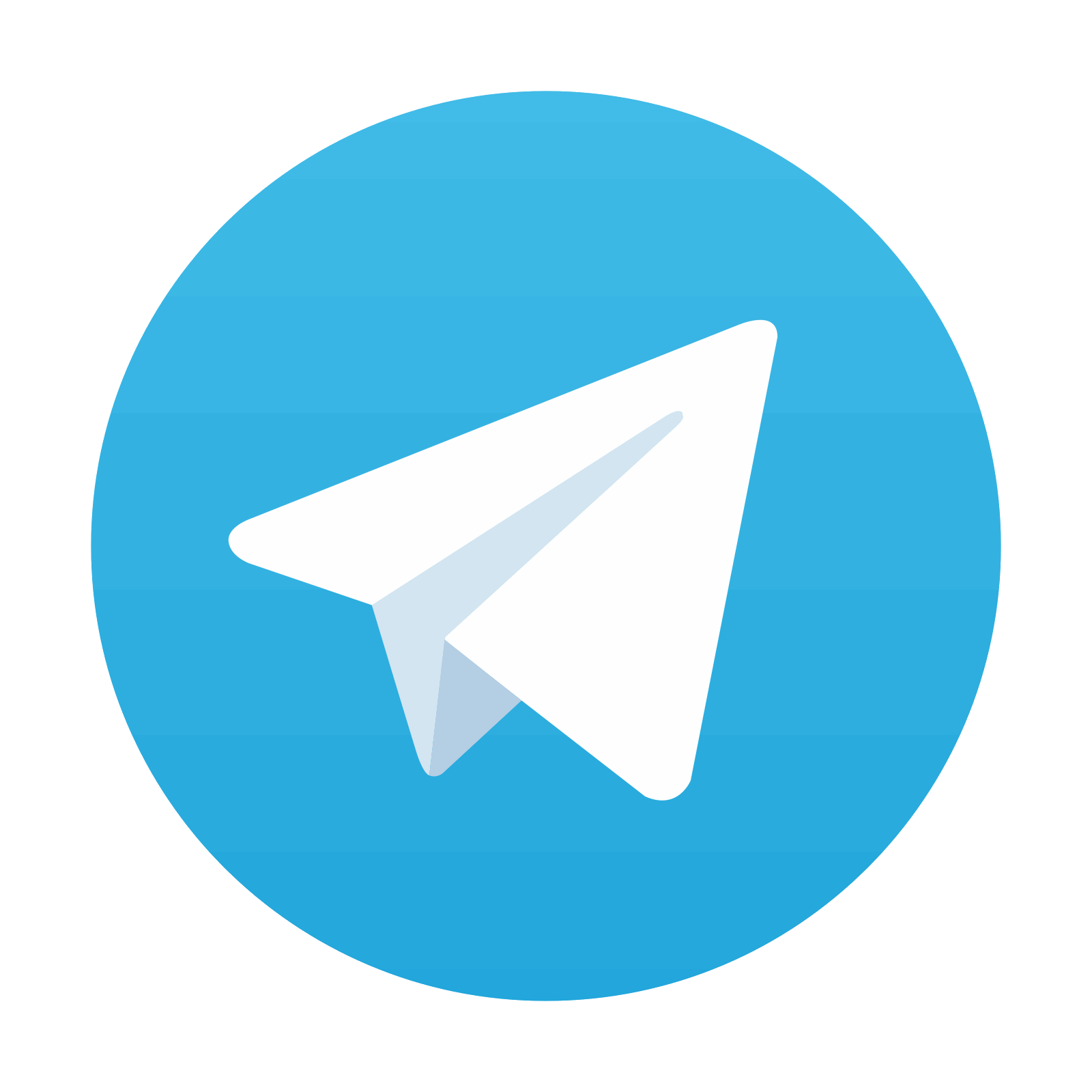
Stay updated, free articles. Join our Telegram channel

Full access? Get Clinical Tree
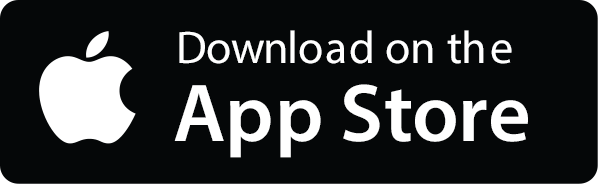
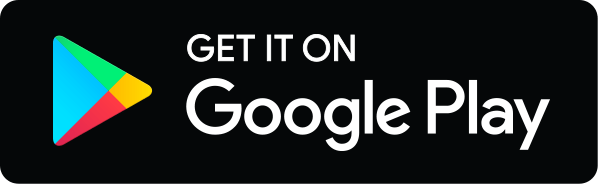