© Springer Science+Business Media New York 2016
R. Lor Randall (ed.)Metastatic Bone Disease10.1007/978-1-4614-5662-9_1313. Biotargeting in Metastatic Bone Disease
(1)
Orthopaedics and Rehabilitation, University of Rochester Medical Center, 601 Elmwood Ave., Box 665, Rochester, NY 14642, USA
(2)
Orthopaedic Oncology, University of Rochester Medical Center, 601 Elmwood Ave., Box 665, Rochester, NY 14642, USA
Keywords
Bone metastasisBiotargetsInterleukinsVEGFEGFBMPOver one million cases of cancer are diagnosed each year in the USA, and more than half of these will go on to involve the skeleton [1]. Bone metastases can be the presenting symptoms of previously undiagnosed malignancy; and, it can also be the harbinger of death in the form of end-stage oncologic disease. The two most common etiologies of metastatic bone disease (MBD) are breast and prostate cancer, which account for 80 % of cases. This association is logical as these two diseases are the most prevalent primary tumors. Additional sources of MBD include lung, kidney, colon, and thyroid cancers. Multiple myeloma and lymphoma/leukemia account for fewer cases but also have a tendency to develop bony lesions.
Several important principles of metastatic disease have been elucidated and accepted as fairly universal across pathologies. The mechanism of metastasis involves four key steps: (1) primary tumor growth and angiogenesis; (2) intravasation and dissemination through the vasculature and/or lymphatic system; (3) tumor cell arrest and extravasation at the secondary site (bone, in our focus); and (4) metastatic tumor cell survival and proliferation.
Animal model studies have shown that millions of tumor cells are released into the circulation by a primary tumor; however, only a few micrometastases are produced. There are many theories for this seemingly inefficient process. One theory suggests that a subset of tumor subclones are responsible for navigating through all of the steps of metastasis and, if they are not present, the cell will undergo apoptosis. Another theory suggests that most of the primary tumor cells have to develop multiple genetic abnormalities, a so-called metastatic signature, before obtaining metastatic potential. Whether it is a small subset or more universal change, research strongly suggests genetic alterations that act primarily on tumor dissemination. For example, increased activation of Ras initiates the Raf-MEK-ERK-MARK pathway , which leads to uncontrolled cell proliferation. Another feature of tumor cell survival is the ability to avoid immunological detection. Acquiring cell properties similar to osteoblasts, osteomimicry, or endothelial cells, vasculogenic mimicry can make tumor cells more invasive and resistant to local host defenses.
The interaction between the tumor cells and the extracellular matrix (ECM) is a critical step in the metastatic process. Invasion of the requires primary tumor cells to detach from each other, interact with the matrix proteins, degrade the matrix, and migrate through it to reach a blood vessel or lymphatic channel.
Bone has high potential for metastatic nesting partly due to its high vascular flow through red bone marrow. Furthermore, bone contains a proliferative quantity of growth factors, especially during bone turnover phases. Transforming growth factor-beta (TGF-b ) , insulin-like growth factors (IGFs) , fibroblast growth factors (FGFs ) , platelet-derived growth factors (PDGFs ) , and bone morphogenic proteins (BMPs ) can aid in tumor cell proliferation, chemotaxis, and avoidance of apoptosis. Multiple chemokines are intricately involved in this process. Chemokine ligand 12 (CXCL12) , known as stromal cell-derived factor 1, is produced by bone marrow stromal cells, as the name suggests. When CXCL12 binds chemokine receptor 4 (CXCR4) on tumor cells, it directs the tumor cell to “home-in” and seed to bone. When CXCR4 is neutralized by a monoclonal antibody, metastasis decreases; this is an example of the therapeutic potential in elucidating the specific molecular pathways of the metastatic process.
Similarly to the process of detaching from the primary tumor, metastatic tumor cells must regulate the expression of cell adhesion molecules (CAMs) to attach to the host organ’s vascular endothelial cells in preparation for invasion and seeding. Cadherins, selectins, and integrins are classes of CAMs shown to be involved in this process. Once into the secondary organ, metastatic tumor cells cause dysregulation with matrix-degrading enzymes called matrix metalloproteinases (MMPs) . Increasing the basement membrane permeability, this process in addition to activation of motility factors, such as IGF-1 and interleukin-8 (IL-8), permits tumor cell migration. Lastly, the metastatic tumor cells require a vascular supply and activate unregulated angiogenesis.
Biotargeting in Breast Cancer
A novel and promising target in the prevention of breast cancer metastasis to bone is IL-8. Elevated levels of IL-8 have been observed in a variety of breast cancer cell lines in vitro, as well as the plasma of patients with bone metastases [2–4]. Breast cancer cell lines that express high levels of IL-8 have been shown to grow aggressive bone tumors in vivo, while lines with reduced levels of IL-8 expression do not. In addition, patients with high serum levels of IL-8 have been shown to have a poorer relapse survival [5]. IL-8 has also been shown to be a direct stimulator of osteoclastogenesis and bone resorption [6], and this mechanism is independent of the RANKL pathway. IL-8 directly stimulates osteoclasts, as well as their precursor cells via activation of CXCR1 an IL-8 receptor located on mature osteoclasts and osteoclast precursor cells [3]. Therefore, anti-IL-8 therapy may prove to be of benefit in prevention of breast cancer metastasis to bone.
Another promising target in the prevention of breast cancer to bone, especially in patients with concomitant inflammatory conditions, such as rheumatoid arthritis, is interleukin-17A (IL-17A) . Studies have shown that chronic inflammation can lead to the development of various types of malignancies [7, 8] and in fact patients with breast cancer and inflammatory arthritis have a poorer prognosis and decreased survival when compared to patients without concomitant autoimmune disease [9, 10]. IL-17A is a pro-inflammatory cytokine, which can in turn stimulate the release of several other cytokines including interleukin-6 (IL-6) and IL-8 to help mediate, its pro-inflammatory effects [11]. IL-17A has been linked to an increased incidence in metastasis in breast cancer as well as other cancers. Antibodies directed at IL-17A have been shown to decrease the incidence of breast cancer metastasis to bone in vivo, as well as invasiveness of breast cancer cell lines in vitro [12–15]. This is thought to be due to the downregulation of CXCL12/SDF-1, which is known to be involved in cancer metastasis [15–17], and is actively being targeted as a treatment strategy for a variety of cancers [18, 19].
A final promising new treatment strategy for the treatment of metastatic breast cancer to bone is recombinant Apo2 ligand/TNF-related apoptosis-inducing ligand (Apo2L/TRAIL) . This treatment strategy targets tumor cells themselves instead of targeting components of the bone microenvironment that make up a bone metastasis niche which is thought to regulate a cancer’s ability to metastasize. Apo2L/TRAIL is a member of the TNF family that induces apoptosis in a variety of cancer cell lines, and appears to preferentially target tumor cells as opposed to normal cells, making it a more tumor-specific treatment, as opposed to a treatment with many systemic side effects [20–23]. Recombinant human apoptosis ligand 2/tumor necrosis factor-related apoptosis-inducing ligand (rhApo2L/TRAIL) is an optimized soluble form of an endogenous apoptosis-inducing ligand, which has been found to have antitumor activity in both in vitro and in vivo models. In addition it has been shown to be effective when used alone and in combination with more conventional therapies [24]. Clinical trials have shown that rhApo2L/TRAIL is generally well tolerated and safe both alone and in combination with other agents [25–27]. A recent study examined the effect of rhApoL2/TRAIL treatment both alone and in combination with a RANKL inhibitor, a more conventional treatment for bone metastasis. They found that treatment with rhApoL2/TRAIL in an animal model of breast cancer rapidly reduced the skeletal tumor burden in their animal model. In addition, addition of a RANKL inhibitor further reduced the skeletal burden of disease [28]. This is an exciting new potential therapeutic option for patients with skeletal metastasis, and could potentially augment the more traditional treatment approaches we have now for these patients.
Biotargeting in Prostate Cancer
Bone morphogenetic proteins (BMPs) belong to the TGF-beta superfamily and play an important role in skeletal growth and development. BMPs are also detected in both primary tumors and metastatic bone tumors in prostate cancer [29, 30]. Thus, BMP-mediated pathways are assumed to be involved in the osteoblastic metastasis of prostate cancer. BMPs are thought to be promoters of prostate cancer metastases to bone by stimulating cellular migration and invasiveness of prostate cancer cells. In addition, the overexpression of the BMP antagonist, noggin, in prostate cancer cell lines has also been shown to inhibit bone metastasis in a mouse model [31, 32]. Therefore, targeting BMPs may play a crucial role in the prevention of prostate metastasis to bone.
The Wnt signaling pathway has been implicated in a variety of cancers including a number of GI and GU cancers, including prostate cancer [33]. The Wnt proteins bind to a number of receptor complexes and ultimately induce β-catenin activity. Wnt signaling is essential for skeletal growth [34, 35] and has been shown to be involved in prostate cancer bone metastasis [36]. Wnt proteins have been shown to increase expression of bone morphogenetic protein-4 (BMP-4) and bone morphogenetic protein-6 (BMP-6) in prostate cancer cell lines, and this BMP expression contributes to the pro-osteobastic activity of the Wnt proteins. However, Wnt proteins also mediate osteoblastic activity through BMP-independent pathways, indicating that the Wnt proteins may be a potential target in treating prostate cancer bone metastasis [37].
Biotargeting in Thyroid Cancer
Angiogenesis plays an important role in the ability of tumor cells to grow and metastasize, and is thought to be quite important in the development of more aggressive thyroid cancer s. Vascular endothelial growth factor (VEGF) is an endothelial-specific mitogen and plays a key role in tumor angiogenesis in thyroid carcinoma, as well as tumor cell proliferation through downstream activation of tyrosine kinase [38–40]. In general, tyrosine kinases function to stimulate tumor proliferation, angiogenesis, tumor invasiveness, and metastasis. There has been an interest in using tyrosine kinase inhibitors in the treatment of thyroid cancer since the discovery of mutations in tyrosine kinases RET and RAS that play a role in oncogenesis, in addition to their ability to inhibit growth factor receptors such as VEGF receptor [41, 42]. Tyrosine kinases affect regulation of both cancer cells and noncancerous cells, and therefore, targeting downstream targets may provide more tumor-specific effects, and help to limit systemic toxicity. VEGF mRNA and protein levels are associated with high mitogenic activity and increased growth in thyroid cancer cell lines. The overexpression of VEGF in differentiated thyroid cancer has been correlated with poor prognosis, increased risk of recurrence, and greater probability of metastasis [43, 44]. Inhibition of VEGF production or VEGFR phosphorylation has been shown to reduce the growth of thyroid cancer cell lines as well as growth of these cell lines in animal model [45–47]. Studies have also shown stabilization of disease progression, as well as prolonged progression-free survival in thyroid cancer patients treated with anti-VEGF therapy [48, 49]. VEGF inhibitors are currently being used in the management of renal cell carcinoma, as well as gastrointestinal stromal tumors (GIST), among others, and may offer a promising new target in the management of thyroid cancer metastases to bone.
Epidermal growth factor receptor (EGFR) is a growth factor that stimulates cell growth, proliferation, and differentiation. Mutations that lead to EGFR overexpression have been associated with a number of cancers [50]. Binding of EGFR to its cell surface receptor initiates several signal transduction cascades, principally the MAPK, Akt, and JNK pathways, leading to DNA synthesis and cell proliferation [51]. EGFR overexpression is implicated in the progression of aggressive thyroid carcinoma [52] and EGFR has been found to be significantly overexpressed in metastatic thyroid tumors compared with primary tumors [53]. In addition, co-expression of EGF and EGFR is also associated with bone metastasis thyroid cancer [54]. Disruption of EGFR signaling decreases the growth and invasion of thyroid cancer cells in vitro [55].
Given the importance of both EGFR and VEGF in the progression of metastatic thyroid cancer, blockade of both EGFR and VEGFR tyrosine kinase activity may offer an important approach for the treatment of bone metastasis in the setting of thyroid cancer. Suppression of both EGFR and VEGFR signaling significantly reduces thyroid cancer tumor volume in nude mice by both direct antitumor and antiangiogenic effects [56]. A phase II multicenter study in France was recently completed to examine the safety of sunitinib, a multiple receptor tyrosine kinase inhibitor, in the treatment of thyroid cancer. Results of the study are not yet available, but will hopefully lead to phase III clinical trials to examine the drug’s efficacy in the treatment of thyroid cancer. However, targeting of tyrosine kinase inhibitors effects both cancerous and noncancerous cells, so blockade of downstream targets may provide more tumor-specific advantages, and limit systemic toxicity.
Biotargeting in Lung Cancer
Lung cancer originates in a highly vascular and well-oxygenated environment with tremendous exposure to toxic elements. Cigarette smoking is a very-well-established major risk factor for lung cancer development. For many cancers, there is an extensive latency period between primary tumor diagnosis and development of metastatic disease. Lung cancer, however, frequently does not display this latency period, suggesting that primary tumor cells are sufficiently adapted to survive at distant sites more readily than other carcinomas. Several features of lung cancer may be responsible: (1) the toxic insults responsible for the initial tumor development may confer additional pro-metastatic mutations; (2) many lung cancers are diagnosed at a late stage.
With medial overall survival of less than 6 months, NSCLC management algorithms do not include a standard prospective screening strategy to detect BMD. The European Society for Medical Oncology (ESMO) recommends a bone scan when there is bone pain, hypercalcemia, or elevated alkaline phosphatase. Skeletal-related events, defined as pathologic fracture, spinal cord compression, palliative radiotherapy, or surgery to bone, consistently predict life expectancy for NSCLC patients [57].
Like many other solid organ tumors, lung cancer cells have distinct preferences for the tissues to which they metastasize, the so-called tissue tropism. The ability of primary tumor cells to direct adaptation at distant sites for future colonization has been termed developing a “pre-metastatic niche” [58]. Evidence suggests that tumor cells navigate through the aforementioned stages of metastasis via interactions with stromal environments both at the primary site and metastatic site. The role of mesenchymal stem cells (MSCs) in tumor-stromal interaction is an important area of study. Patients with untreated lung cancer without bony metastasis exhibit changes in MSC plasticity that predisposes the bone to osteolysis [59]. These tumor-stromal interactions are critical in the metastatic process and an important potential area of therapeutic intervention.
While there is some evidence to suggest pre-metastatic conditioning in the bone marrow, the bone marrow environment has several molecular features favorable for lung cancer micrometastases [60]. The interaction between osteoclasts and osteoblasts promotes bone degradation, which releases extracellular matrix-bound growth factors. These, in turn, promote the growth of tumor cells. Examples of tumor-secreted factors that stimulate osteoblast and osteoclast activity are interleukins (IL-1, IL-6), receptor-activator-of-nuclear-factor-Kappa-B-ligand (RANKL), parathyroid hormone-related protein (PTHrP) , and macrophage inflammatory protein-1-alpha (MIP-1a). Tumor-induced secretion of osteoclastic factors trips a vicious cycle of osteoclast-mediated bone resorption. In addition, proteolytic degradation of bone matrix occurs through tumor secretion of MMP and other proteases released at the tumor-stromal interphase [61]. In turn, osteoclasts then release TFG-beta and IGF-1 from bone matrix which stimulate PTHrP production and, therefore, promotes tumor growth. PTHrP stimulates osteoclast activity, prevents osteoclastic apoptosis, and enhances renal tubular reabsorption of calcium.
Tumor cells achieve local bone resorption by activating osteoclast precursors. The osteoclastogenesis process is regulated by the receptor-activator-of-nuclear-factor-Kappa-B-ligand (RANKL)/RANK/osteoprotegerin (OPG) pathway. RANKL, a member of the tumor necrosis factor (TNF) family, is expressed on the surface of osteoblasts and osteocytes and released by activated T cells. RANKL binds RANK, which is present on osteoclasts and osteoclast-precursors, and activates NF-kB, a transcription factor that is also activated within lung cancer cells by EGFR/oncogenic K-ras [62]. Increased expression of RANKL induces osteoclast formation and activation, which increases bone resorption and local bone destruction. OPG is a soluble glycoprotein that binds RANKL and competitively inhibits the RANK-RANKL interaction .
Targeting this pathway has been the focus of intense research interest in all MBD in solid tumors. Bisphosphonates, synthetic analogues of pyrophosphate, are anti-osteoclastic agents by inhibiting osteoclast-mediated bone resorption. Zoledronic acid has been shown to decrease the number of SREs [63]. More recently, investigations are targeting the RANKL/RANK/OPG pathway directly. Denosumab, a monoclonal antibody that binds and neutralizes RANKL and thereby inhibits osteoclast function, has been approved for use to help prevent SREs in patients with BMD from solid tumors [64]. A phase III trial compared zoledronic acid with denosumab in patients with multiple myeloma or solid tumors (40 % of which were NSCLC); when stratified by tumor type, there was no statistically significant difference between the two therapies for time to first SRE [65, 66]. Bisphosphonate use has been limited in NSCLC as compared with other solid organ cancers; in part, this may be attributable to intrinsic nephrotoxicity of this drug class. Furthermore, previous cisplatin nephrotoxicity and smoking-related arteriosclerosis may limit the therapeutic risk-benefit ratio.
Another potential important mediator in the tumor-stromal interaction is epidermal growth factor (EGF) , which directly stimulates tumor cell proliferation and indirectly increases bone stromal favorable for metastasis [67]. Gefitinib, an EGFR inhibitor, may block osteoclast activation by inhibiting EGF signaling in bone stromal cells. Tumor cells release three EGF-like factors: heparin-binding EGF, amphiregulin (AREG), and TGF-alpha; these activate the EGFR pathway in osteoblasts and downregulate OPG expression [68, 69]. Therefore, the disinhibition of this competitive inhibitor of RANK-RANKL osteoclastogenesis should theoretically diminish this vicious cycle. Moreover, there is evidence of osteoblastic reactions in patients treated with EGF tyrosine kinase inhibitors (TKI); however the mechanism and implications are not well understood.
Biotargeting in Renal Cancer
Because of RCC’s poor response to standard chemotherapies, alternative treatment options have been the standard of care for systemic disease. The primary treatment for non-metastatic disease is surgery alone. Until recently, very few systemic treatments have been approved for first-line therapy of isolated renal disease. Prior to 2005, immunotherapy with high-dose interleukin-2 (IL2) and interferon-α (INFα) were the standard therapies available for advanced or systemic RCC. These treatments are not well tolerated by patients due to side effects and have shown only a modest improvement in survival of around 3 months compared to placebo. Starting in 2005, targeted therapies for RCC began to become available. Most of these therapies were believed to be working through inhibition of the VEGF-mediated pathway, which is thought to be deregulated as a result of VHL gene dysfunction. Bevacizumab, an anti-VEGF antibody, was first approved for use in colon cancer patients in 2004. It was found to have a modest response in patients with RCC and was approved for combination use with INFα in 2009 for patients with RCC. It is one of the few treatments now approved for first-line treatment of non-metastatic RCC [79]. The major side effect of this antibody is increased risk of thromboembolic events.
The most recent round of molecular targeted therapies for RCC has focused on the mammalian target of rapamycin (mTOR) pathway. This pathway is involved in the regulation of cell growth and cell proliferation. It has been the focus of research recently in cancer therapy as this pathway appears to be integral to cell proliferation in several cancers [80]. It is thought to work in part by regulating flow of nutrients in and around the cell and may be bringing in new blood supply via angiogenesis by increasing translation of HIF1α and HIF2α. The first intravenous form of an mTOR inhibitor, temsirolimus, was approved in 2007 for metastatic RCC, followed by approval of the oral mTOR inhibitor, everolimus, in 2009. In a randomized clinical trial, temsirolimus was found to improve survival by approximately 3.5 months compared to INFα alone [81]. The side effect profile was also well tolerated by patients. This agent is now being used for patients with poor prognostic risk factors or metastatic RCC.
There are several upcoming targeted therapies that have some promise in the treatment of metastatic RCC. Several new RTK inhibitors that are more directed towards the tyrosine kinase receptors involved in RCC are under development and in early clinical trial. Similarly, there has been work done in the development of more selective VEGF inhibitors . These newer molecules are hoped to reduce the side effect profile compared to the currently available treatments, which may allow for combination therapies and higher dose treatment. This could ultimately help improve survival and decrease metastatic bone disease morbidity.
Unfortunately, all of the agents available to date and even some of the newer therapies on the horizon are cytostatic agents and not cytotoxic [70–75]. As a result, ultimately the RCC cancer cells develop resistance with inevitable disease progression. What is needed now for metastatic RCC are agents that induce cell death. There is some hope in this area. Naptumomab estafenatox is a fusion protein that binds cancer cells and leads to T-cell-activated cell destruction [75]. There is some hope that this could be an effective treatment for RCC and other cancers as well. There has also been work looking at the induction of apoptosis in RCC. Inhibition of the mTOR pathway is thought to partially work through this mechanism [76]. Similarly, targeted therapies looking at inhibition of EGF receptor may be working through induction of cell apoptosis [77, 78].
Recent evidence suggests that the calcium-sensing receptor present in normal renal tissue is up-regulated in metastatic RCC to bone compared to non-metastatic RCC or metastatic lung RCC. In these same cells that showed up-regulation of the calcium-sensing receptor there was increased RCC cell migration in the setting of a high-calcium substrate [79]. These findings strongly suggest that there are molecular differences in RCC tumors that spread to bone and that there could be potential targets that specifically prevent bone metastasis, such as blockage of the calcium-sensing receptor in high-risk patients. Other targeted therapies that specifically look at the bone environment have focused on proteins that are involved in the angiogenesis pathway. The rationale for studying this pathway goes back to our understanding that RCC is a very vascular tumor and appears to be dependent on that vascularity for growth. The angiopoietin family is a set of proteins that have been studied as potential targets in metastatic RCC. Like the VEGF family, they are involved in vessel development and have shown increased expression in RCC to varying degrees. There is evidence to suggest that decreasing angiopoietin-1 and increasing angiopoietin-2 are linked to a poorer prognosis [80–82]. Molecular studies of cell lines have confirmed that RCC with bone affinity seems to have increased expression of Ang-2 and decreased expression of Ang-1 [83].
Another protein that has been linked to prognosis and pathogenesis of metastatic RCC to bone is semaphorin-4D and its receptor Plexin-B1. Semaphorin-4D (Sema4D ) is a membrane-bound and -secreted protein initially identified as a cell signaling molecule present on activated T-cells. Activation of Plexin-B1 through binding of Sema4D is thought to be coupled with c-Met activation, which in turn leads to downstream endothelial cell migration and capillary formation. This pathway appears to be independent of the VEGF-mediated pathway [84, 85, 87]. Newer evidence has also shown that this diverse molecule is implemented in the signaling pathway between osteoclasts and osteoblasts. Most notably, it has been shown to be expressed in high levels on the surface of osteoclasts as well as present in the supernatant of cultured osteoclasts, while its receptor Plexin-B1 has been found on the surface of osteoblasts. Sema4D has been shown to reduce osteoblast differentiation and thereby bone formation. Sema4D knockout mouse models have a higher bone mass phenotype with a higher rate of bone formation [86]. Therefore, this molecule is a potential ideal target for therapy specific for preventing RCC-mediated bone destruction and tumor growth within bone.
Finally, there has also been some headway made in using biomarkers to help predict a patient’s response to therapy. This may greatly help treat patients with RCC because there are so many agents available and it is difficult to predict which will be most effective in slowing tumor growth. Work in this area is still ongoing, but the potential for helping treat patients with RCC is high.
Biotargeting in Multiple Myeloma
One of the hallmarks of multiple myeloma , and perhaps the primary concern for orthopedic surgeons, is the extensive destruction of bone. In skeletal lesions, increased production of multiple cytokines (IL-1β, IL-3, and IL-6) helps promote osteoclastic differentiation, while additional factors (TNFα, hepatocyte growth factor [HGF], and VEGF) further support osteoclast survival and bone resorption [89]. Myeloma cells produce macrophage inflammatory protein-1α (MIP-1α), a chemokine involved in cell adhesion and migration, which stimulates survival, proliferation, and migration of myeloma cells [90]. MIP-1α-induced expression of IL-6 and RANKL by local marrow cells may play a significant role in osteoclastogenesis and the development of skeletal lesions [88, 91].
One of the key features that appear to help propagate the survival of myeloma cells is the ability of the cells to create a symbiotic relationship between themselves and the surrounding bone marrow environment. Several novel therapies are now emerging that specifically target that interaction. There has been evidence to suggest that targeting the bone can not only reduce skeletal events in myeloma, but also improve survival. This has been seen in the use of bisphosphonates in myeloma with evidence of improved survival seen in patients placed on bisphosphonates [92, 93]. Bisphosphonates are currently the standard of care for myeloma-induced lytic bone disease. Bisphosphonates have been found to reduce skeletal events in myeloma patients, but have not been found to reverse the osteolytic process once established. One clinically available option that has strong potential therapeutic benefit in myeloma bone disease is the RANKL inhibitor denosumab. The RANKL, OPG, and Rank pathway is integral to the lytic bone disease seen in all metastatic bone disease and myeloma is no exception [94]. Phase II and III clinical trials are under way for use of denosumab in myeloma patients as an alternative to bisphosphonates.
Some other potential targets that are being looked at as options for directly inhibiting myeloma-induced bone disease include Dickkopf-1 (Dkk-1) and sclerostin [95]. Dkk- 1 inhibits the Wnt/beta-catenin pathway by binding to LRP5/6 receptors. Inhibition of Wnt leads to increased bone destruction via osteoclastogenesis and decreased bone formation via reduced osteoblastogenesis. Antibodies directed at Dkk-1 have been shown to reduce osteolysis and increase bone density in myeloma mouse models. Currently, clinical trials are under way looking at potential antibody inhibition of Dkk-1 in metastatic disease and myeloma. Sclerostin is another Wnt/beta-catenin pathway inhibitor that is under investigation as a target to prevent and reverse bone loss in patients with osteoporosis [96]. Although not utilized yet in cancer patients, it also has much potential as a targeted therapy to prevent neoplastic cell-induced bone destruction in multiple myeloma and other malignancies.
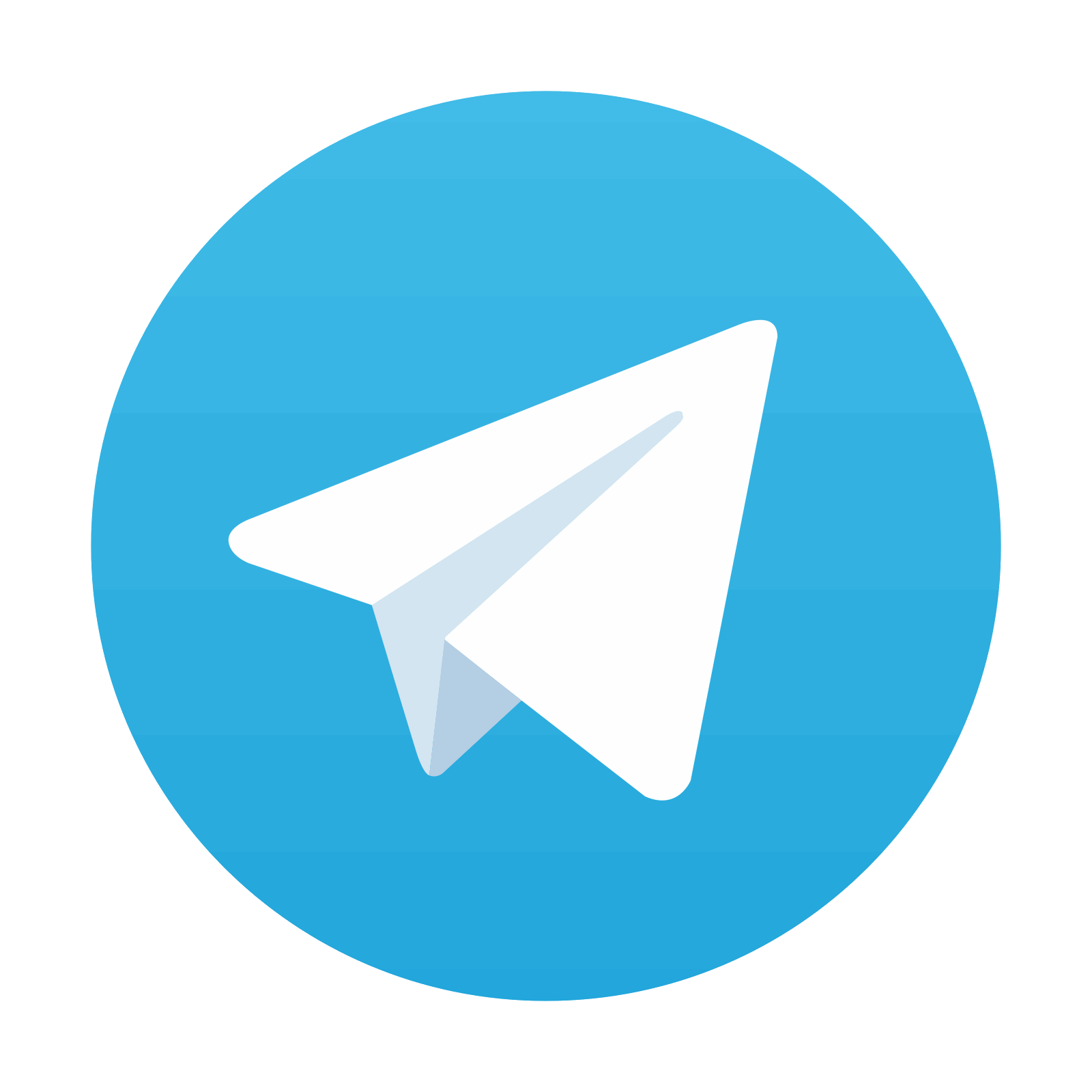
Stay updated, free articles. Join our Telegram channel

Full access? Get Clinical Tree
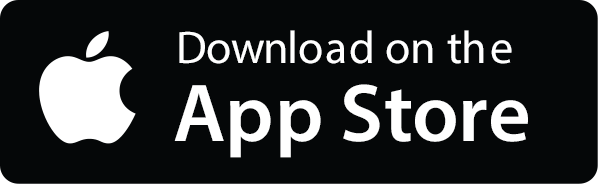
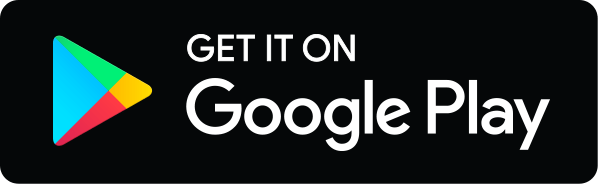