Fig. 22.1
Annular fibers are oriented in a 30° orientation with the endplate (a). This permits a significant torsion prevention potential (arrows) (b). In fact, they are more optimally oriented for torsion prevention than for distraction (or compression) prevention. If the annular fibers are lax (c), torsion resistance diminishes (arrows) (d). Chronic instability and mechanical pain may result. Lax annular ligaments also predispose to the more commonly observed imaging correlate of chronic instability and subluxation (e)
The rostral and caudal endplates are composed largely of hyaline cartilage and are firmly attached to the adjacent vertebral bodies by calcified cartilage. The endplates act as a semipermeable barrier between the vascular medullary portion of the vertebral bodies and the disk. Disks in adults and children are avascular and nutrients must enter through the adjacent endplates or capillaries into the annulus fibrosis [5]. In contrast, infant’s disk spaces are vascular and therefore nutrients enter the annulus via arteries or arterioles.
22.3 Function of the Intervertebral Disk Space
The intervertebral disk space composes 20–25 % of the vertebral column height. In axial loading the disk acts as shock absorber for the relatively incompressible vertebral body. The disk is exposed to variable compression loads throughout the day. The effects of position on the hydrostatic disk have been measured and confirmed by various authors [6–8]. As body weight increases with aging or when one rises from a supine position, the load on the disk space increases, predominantly in lower lumbar levels [5]. In the supine position with knees bent and supported, the lumbar spine is relaxed in a slightly less lordotic posture. The disk pressure in this position is the least and is primarily produced by surrounding ligaments and musculature. As a standing position is taken, the pressure in the disk increases as it takes on more of the axial load of the body. In a resting standing position, the majority of the pressure is borne in the center of the disk and is fourfold greater than that of being supine. As the nucleus pulposus compresses under axial loading, it bulges out laterally and is contained by the annulus fibrosus. The collagen fibers of the annulus are placed under tension and thus act as a capsule to contain nucleus pulposus. With bending, the nucleus migrates away from the increased force and the annulus bulges in the direction of the concavity of the curve. For instance, bending forward produces more kyphosis in the lumbar spine. In this position, the pressure in the ventral annulus is increased and the nucleus pulposus moves dorsally, where the pressure is less. This dorsal displacement places the dorsal annulus under tension as the nucleus pushes against it (Fig. 22.2).


Fig. 22.2
An axial load causes an equally distributed force application to the disk (a). An eccentric force application results in annulus fibrosus bulging on the side of the greatest force application (i.e., the concave side of the bend) (b). The nucleus pulposus moves in the opposite direction. Dashed lines indicate the positions of structures during force application (c)
With asymmetric loading of the spine, the intervertebral disk provides a measure of stiffness that is augmented by extra-discal structures such as spinal ligaments, muscles, and facet joints [7, 9]. To achieve both stability and motion, each of the components of the intervertebral disk plays different yet critical roles.
Under compression, the nucleus pulposus plays an important role in maintaining equal pressure along the endplates and maintaining intervertebral disk height. The osmotic properties of the intervertebral disk space are crucial regarding these roles. This osmotic system is centered about the hydration of the nucleus pulposus and abilities of the annulus fibrosus and the cartilaginous endplates to act as semipermeable membranes through which water moves.
The movement of water is governed by several factors. The forces that keep water within the disk are the extra-discal hydrostatic pressure and the oncotic pressure of the nucleus ground substance. The forces that drive water out of the disk are the extra-discal oncotic pressure and the intra-discal hydrostatic pressure. The semipermeable barriers through which water moves are the endplates and the annulus fibrosus. As axial loading increases, the intra-discal hydrostatic pressure increases, driving fluid out of the disk. As fluid leaves, the anionic charge of the mucoid ground substance becomes more concentrated and thus pulls fluid back into the disk so that the following equilibrium is maintained: intra-discal hydrostatic pressure + extra-discal oncotic pressure = extra-discal hydrostatic pressure + intra-discal oncotic pressure. With changes in compressive forces (i.e., position changes during the day), fluid shifts between the intra-discal substance and medullary bone to maintain this equilibrium [6, 7, 10]. These fluid shifts facilitate the transport of nutrients and wastes and may be important for the overall health of the disk.
As previously noted, the vertebral endplates act as firm surfaces that transfer axial loads to the intervertebral disk. They also act as semipermeable membranes that allow not only water but also nutrients to diffuse through from the vascular medullary bone of the vertebral bodies into the relatively avascular disk space. Disruption of the vertebral endplates is linked with disk degeneration and is discussed in further detail later.
22.4 Degenerative Changes in the Intervertebral Disk Space
Many events can occur to weaken the main components of the intervertebral disk leading to degenerative changes. The most common level of disk degeneration is at L4/5 and degeneration is more common at multiple levels as compared to a single level [1]. Degeneration results in decreased nuclear energy dissipation, swelling pressure, and compressive modulus relative to a normal nucleus; additionally, the annulus has a higher compressive stiffness and matrix function of the disk undergoes breakdown weakening biomechanical behavior [3]. Imaging findings that are associated with degeneration include: diminished disk height, facet narrowing, endplate spondylophytes and sclerosis, canal stenosis, lateral recess narrowing, desiccation, fibrosis or diffuse bulging of the annulus beyond disk space, fissuring or mucinous degeneration of annulus, defects or sclerosis of endplates, and osteophytes at vertebral apophyses [1, 11]. Mechanical behavior of the disk is related to biphasic-swelling properties as well as individual components: annulus, nucleus pulposus, and cartilaginous endplates [12].
The degenerated disk displays several interrelated changes. Annular tears develop as chondrocytes and fibroblasts are no longer able to produce quality fibers that can resist the expansile pressure of mobile central disk [5]. These tears are seen as early as the second generation of life and are present primarily in three different patterns: circumferential, transverse, and radial tears [13]. Transverse and radial tears are of greater clinical importance in degeneration as they have been shown to increase segmental motion markedly in rotation as well as moderately in flexion and extension but not significantly in lateral bending [4]. It has also been proposed that transverse tears in the posterior annulus act as a conduit for nucleus pulposus to herniate and cause nerve root compression. Though if the external layer of annulus fibrosus remains intact, there exists a possibility the protruded disk matter can return to its original position [5].
Decreased water content has also been noted in disk degeneration and has been linked to proteoglycan degradation. It is greatest in the nucleus pulposus [1]. Up to 70 % reduction in hydration is observed, resulting in desiccation of both the nucleus pulposus and other components of the disk [1]. The decrease of hydrostatic pressure of nucleus in disk degeneration has been noted both in vivo and in vitro studies [10, 14, 15]. This loss of water decreases the ability of the nucleus to weight bear. This progressively leads to increased weight bearing by the annulus and thus degeneration over time.
Weakening of the endplates may result from trabecular micro-damage and are identified on MRI as disk bulging into the vertebral bodies [16, 17]. With complete endplate disruption, herniation of nucleus fragments into vertebral bodies may occur. These are termed Schmörl’s nodes when they become calcified. The loss of the endplate’s ability to provide a firm surface for translation of compressive forces leads to the loss of the hydrostatic nucleus to weight bear in compression. The resultant compressive forces are then passed to the annulus and the developing fibrocartilaginous scar that forms peripherally. Further calcification of ligaments that span the intervertebral space can result in added fixation of the spinal segment [5]. With increased compressive stress, buckling of the annulus inward into the decompressed nucleus and outward beyond the margins of the vertebral endplates may occur and lead to a further decrease in disk height.
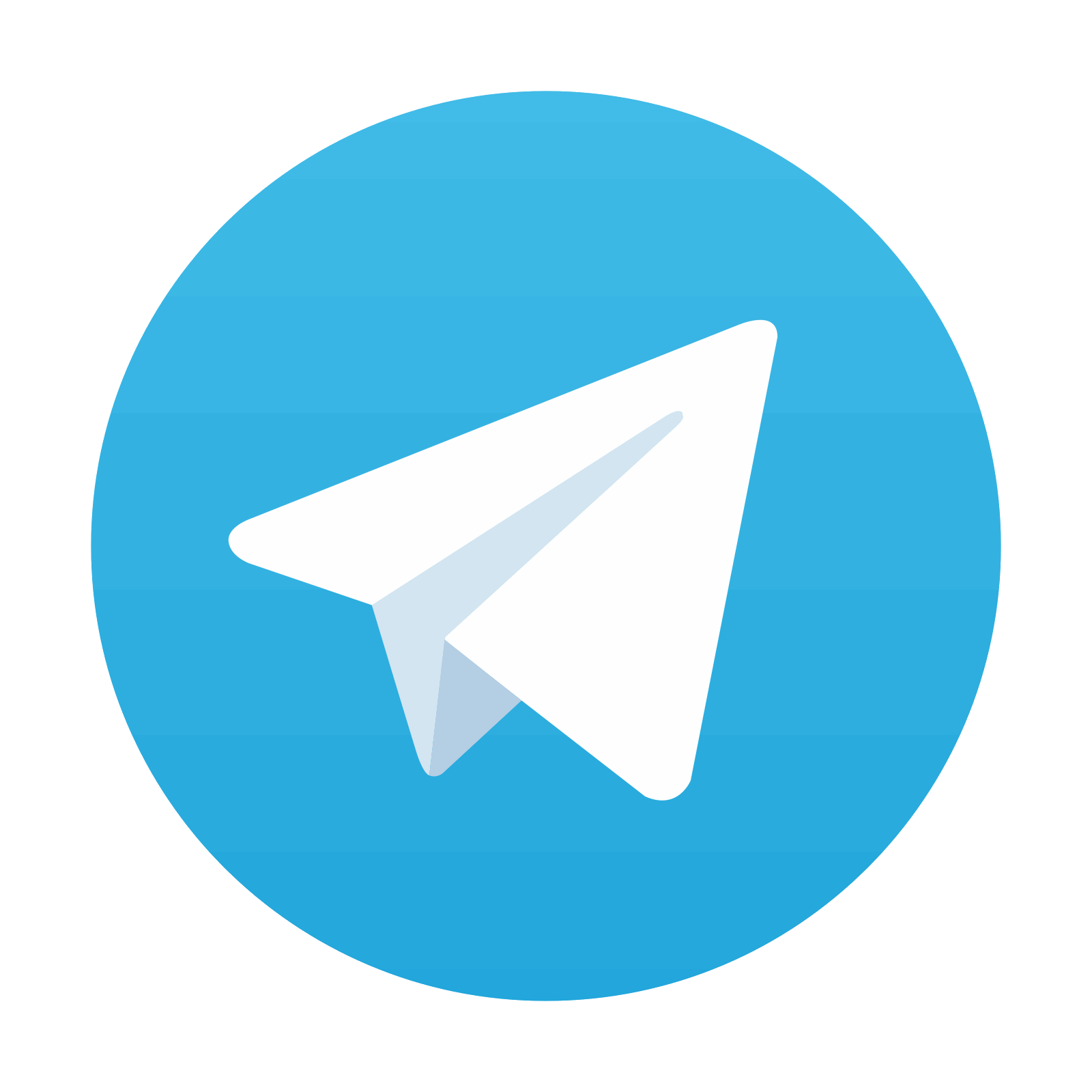
Stay updated, free articles. Join our Telegram channel

Full access? Get Clinical Tree
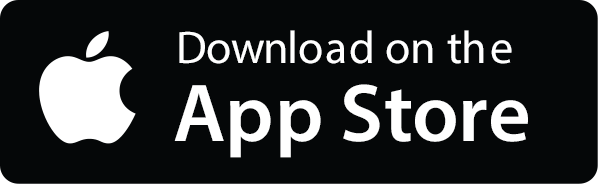
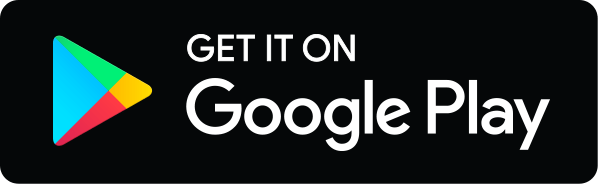