Fig. 2.1
Arm of the forces generated on the elbow joint, when the force is applied: (a) at the level of the wrist, (b) at the level of MCP joint, and (c) at the level of fingertips. Arc of rotation – center of capitellum, attachment of the distal biceps marked by green line on the radial tuberosity, distances in millimeters, my own forearm
In normal circumstances, elbow flexion in man is from 0° to 150°, in woman from hyperextension 12–15° to 150°. The checking agent is an impact of the tip of olecranon to trochlear fossa – in full extension collateral ligaments are taut and stop the movement [26].
So, in example taken from book of Nordin and Frankel [26], the extension moment exerted by the load in the hand is 20 N times 0.35 m, or 7 Nm. This must be opposed by an equal and opposite flexion moment from the muscles. So, if the muscle exerts a moment of 7 N m at a moment arm of 35 mm (0.035 m), the muscle tension T must equal 7 Nm divided by 0.035 m, or 200 N. These calculations are of course simplification, treating elbow like pure hinge joint with all muscles acting parallel to long axis of humerus, like biceps and brachialis. Of course, in real situations, all other muscles (wrist and finger flexors) are clenched to stabilize the lifted object. The tensions in the finger flexor muscles compress the humeroulnar joint, due to flexor digitorum superficialis originating from the medial epicondyle and flex due to action of flexor digitorum profundus. We even sometimes use these muscles to restore elbow flexion in brachial plexus palsy (Steiner procedure). Clenched fist is stabilized as well by extensors – this pressure of the radial head against the humeral joint phenomenon might be illustrated by radial head migration after Monteggia fracture.
Generally, all actions that require a large force to be exerted cause all of the available muscles to contract, not just to produce a movement but also to stabilize the joints [23].
Basic works of Amis, Basmajian, and Long described the function of separate muscles using electromyography investigation. Lower limb carries the body weight during walking, so basic studies evaluate muscle function during gait phases, changing the position of the body. Studies of the upper limb are more difficult; we need to precise exactly, which action is of the interest: lifting heavy objects, stabilizing joints, operating peculiar machines, and ergonomics for industrial purposes.
2.2.1 Capacity and Contact Areas of the Elbow Joint
The capacity of the adult elbow joint is about 25 ml, reaching maximum at 80° of flexion – that’s why stiff elbow most commonly is flexed [28]. The central depression of the radial head articulates with the dome of capitellum; medial triangular facet of TFCC is always in contact with the ulna. With the load of 10 N, about 9 % contact of joint surfaces occurs, with the load of 120 N, it increases to almost 73 % [15].
2.2.2 Stabilization of the Elbow Joint
The elbow is one of the most congruent and stable joints of the human body.
Main reasons for that are almost parallel bony components of joint surfaces and very solid soft tissue stabilizers – lateral and medial collateral ligaments and anterior capsule. Lateral collateral ligament and anterior bundle of medial collateral ligament start from the endpoints of axis of rotation of the elbow joint [34, 35].
Medial collateral ligament has two components: the anterior bundle taut in extension but its posterior bundle is taut in flexion. Lateral collateral ligament assumes rather constant tension during all activities and functions with or without the radial head, central part of it called lateral collateral ulnar ligament that attaches to ulna, thus stabilizes the ulnar-humeral joint and controls the pivot-shift maneuver [27, 34, 35].
In extension, anterior capsule provides about 70 % of soft tissue restraint; in flexion main agent is a medial collateral ligament.
In extension, varus resistance is controlled equally by joint congruency, mainly olecranon in olecranon fossa and lateral collateral ligament; in flexion, joint congruency is responsible for 75 % of stability.
Valgus stress in extension is spread equally among the joint congruency, anterior capsule, and medial collateral ligament; in flexion in 74 %, the medial collateral ligament is responsible for resistance.
Varus and valgus laxity of the elbow depends on the forearm rotation [30]. Increased valgus/varus laxity in medially unstable joints puts, for instance, baseball pitchers in a risk of medial collateral ligament chronic injury due to permanent overload. So, one should clinically test the stability in sportsman in different ranges of prono-supination.
Bony eminences – like tip of olecranon, of coronoid process, and even partial excision of radial head – seem to play a crucial role in the elbow stability, and their restitution in traumatic setting is crucial for the athletes [10].
The role of muscles surrounding the elbow joint in its stabilization was postulated because of their course parallel to collateral ligaments. But in recent electromyographic studies, e.g., of flexor carpi ulnaris and extensor digitorum superficialis, muscles did not reveal their significant activity, when valgus or varus forces were applied [22]. Also baseball pitchers with medial collateral ligament insufficiency did not present any increased EMG activity of these muscles – so they probably do not support collaterals in their function [19].
2.2.3 Elbow Joint Load During Normal Activities
The size of the joint forces obviously relates to the external load. The elbow muscles work at a large mechanical disadvantage when comparing their small moment arms about the joint axis to the large moment arms of loads exerted on the lower limb. The radius of the curvature of joint surface, e.g., of capitellum and the length of the forearm, elongated by the hand and fingers, makes the articular forces surprisingly high.
The same muscles act differently in extension, neutral, and flexed position of the elbow.
In sagittal plane of motion, elbow is a hinge joint. Moments of forces applied at the hand are balanced by the muscles, tendons, ligaments, and contact forces on articular surfaces. In a single muscle, two-dimensional analysis basic equilibrium equation is used:
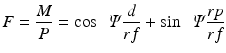
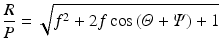
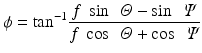
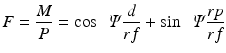
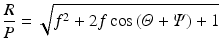
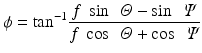
Where Ψ, Θ, and ɸ are the angles between the forearm axis and applied force, P, muscle pull, M, and resultant joint force, R, respectively [4, 7].
Classical works of Amis showed that when the elbow is near full extension, both the forearm muscles and the elbow flexors (principally biceps, brachialis, brachioradialis, and pronator teres) are pulling in similar directions. Their tensile forces then addict, resulting in very large forces acting onto the end of the humerus. This reaches a maximum of 5 kN at 30° flexion, for maximal isometric strength of a normal young male adult [4, 5]. Flexion starts from compressing the elbow joint and tearing radial head a little bit to the front, mainly by the biceps tendon.
In the bended elbow, flexors tend to distract the joint, in opposition to the forearm muscles that are always acting along the forearm. Thus, the elbow forces fall to their lowest values, 1 kN maximum.
Triceps is the primary elbow extensor; it is the strongest single muscle in the upper limb. The lateral head of the triceps does not insert into the tip of the olecranon and passes alongside it laterally and is attached to the fascia of the anconeus. Elbow extension causes large humeroulnar joint forces and that the triceps tension causes large tensile stresses in the olecranon. The joint force varies from 1.5 kN acting onto the distal aspect of the humerus at full extension, to 3.5 kN acting onto the anterodistal aspect at 120° flexion [7].
Pronator teres and pronator quadratus are the main pronators, while supination results primarily from actions in biceps and supinator. These muscles tend to cause forces acting transversely to the axis of the forearm, their action is reinforced by flexors and extensors of the wrist and fingers that pull the bones axially. The result is that the humeroradial and humeroulnar joints are loaded, while the proximal radioulnar joint is subjected to only small forces [4, 5, 11].
Pushing toward the centerline of the body with hand, when the elbow flexed, such as when pulling a mill or like they do in sumo, holding a large object causes torsion load on the humerus. This is in response to tensions in the anterior pectoral muscles causing internal rotation at the shoulder. The distal humerus has to be wide enough to resist the abduction action of the forearm. The load is resisted by a force couple: tension in the medial collateral ligament and compression in the humeroradial joint [4, 5].
When the force is directed along the centerline of the forearm (good technically boxer hit), then approximately 70 % of the load is transmitted by the radius directly to the capitellum [3]. When the force vector passes toward the lateral side of the elbow, in a slight varus position, then all of the load passes directly to the radius and the elbow is stable. If the force passes medially, in a slight valgus, the tenuous lateral collateral ligament structures do not maintain stability, but this situation is not normally encountered due to the carrying angle.
2.3 Interosseous Membrane
Pushing, or falling on extended hand, causes compressive forces to pass through the hand and wrist, then along the forearm to the elbow. One of the elements active in transmission of these forces is interosseous membrane. The exact role of an interosseous membrane is not fully known yet. The central part of the interosseous membrane includes strong interosseous ligament, and its resistance is estimated as similar to patellar tendon. This postulated structure links the radius and ulna, prevents proximal radius migration, and provides transverse stability in between forearm bones. In a fall, radius bears 80 % of the wrist load and 60 % of the elbow load. Interosseous ligament transfers load from hand to elbow and prevents radius and ulna from splaying.
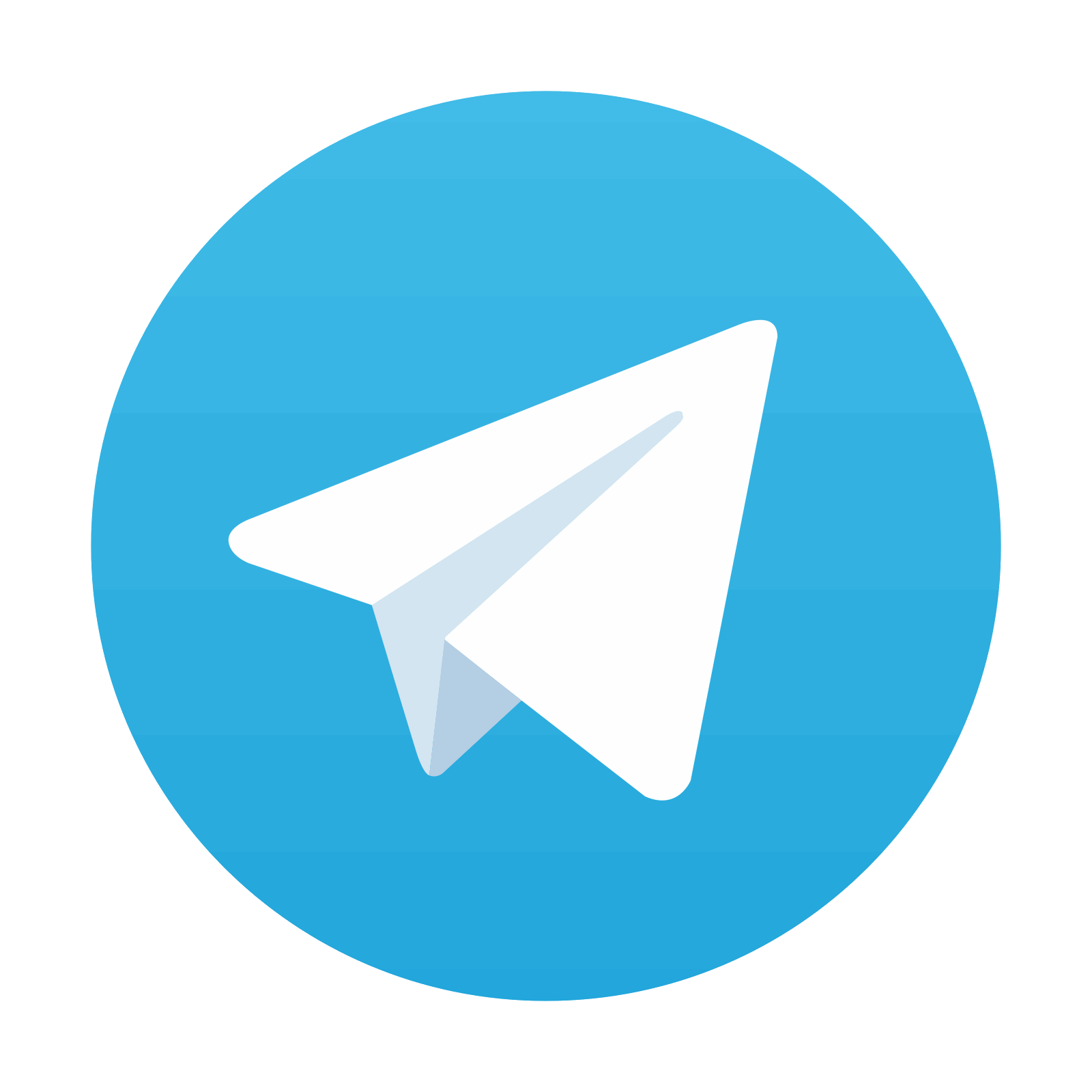
Stay updated, free articles. Join our Telegram channel

Full access? Get Clinical Tree
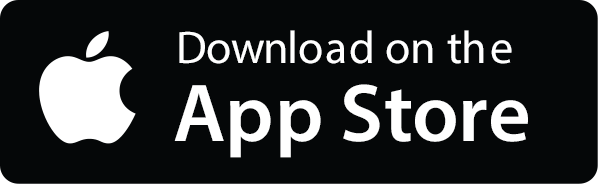
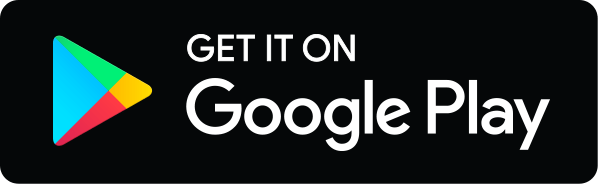