Fig. 2.1
This clinical photograph with all soft tissues removed from the elbow demonstrates the osseous congruity of the articulation. (A) Trochea. (B) Capitellum. (C) Coronoid. (D) Radial head. (E) Medial Epicondyle
Muscular forces interact with articular conformity to maintain elbow stability. Coactivation of the agonist–antagonist group (biceps, brachialis, and triceps) acts to center joint forces within the available articular arc of the ulnotrochlear joint [24, 25], while activation of the wrist extensors acts to center the radial head on the capitellum [15, 26]. Both the ulnohumeral and radiocapitellar joints are stabilized via a concavity-compression mechanism. After instability secondary to trauma, elbow rehabilitation regimens have thus focused upon supine active range of motion focusing on coactivation [27]. Electromyographic studies have shown that the anconeus is active during almost all elbow motions, which has led some authors to suggest that this muscle may also serve a role as a dynamic stabilizer [2]. The triceps has more than twice the cross-sectional area of any other muscle crossing the elbow joint and is larger than the biceps and brachialis combined. Of the flexors, the brachioradialis has a larger moment arm than the biceps, which is also larger than the brachialis . Generally, as muscle moment arms increase both muscle force and joint reaction force increase. Thus, those muscles with insertions closest to the articulation have larger moment arms and can produce the largest joint compression forces and thus make the largest dynamic contributions to stability. Moment is affected by joint position. In flexion, the overall potential flexor moment is equal to the potential extensor moment, while in extension the potential extensor moment exceeds the potential flexor moment. This may contribute to a greater propensity for elbow instability in extension as compared to flexion [2]. Muscle moment arms are also affected by humeral length, with potential triceps force production reduced by 20 % with one cm of humeral shortening, 40 % with two cm of humeral shortening, and 60 % with three cm of humeral shortening [28].
Classically, 40 % of axial loads across the elbow are transferred across the ulnohumeral joint and 60 % across the radiohumeral joint . However, load transfer is sensitive to a variety of factors. Changes in varus and valgus positioning of the elbow can lead to large changes in joint loading force. In valgus, 93 % of axial loads are transferred across the radius [29]. In addition, flexion and extension alter loading, with the radiocapitellar joint being more loaded in full extension [30]. This is due to the fact that in this position the muscles passing across the ulnotrochlear joint have the shortest moment arms [24]. The integrity of the interosseous membrane, in particular the central band [31], also alters load transfer, particular with the elbow in varus [29]. Finally, forearm rotational position alters load transfer, with pronation loading the ulnotrochlear joint and supination loading the radiocapitellar joint [29]. Loss of elbow stability can lead to malalignment and overload of one side of the joint, which can lead to accelerated radiocapitellar or ulnotrochlear degenerative changes.
While abundant cadaveric biomechanical studies have been conducted in attempt to understand the contributors to elbow stability [5, 13–20], these experiments are difficult to perform and their findings can be difficult to generalize to biokinematics in the live patient. First, in addition to displacement, instability can occur with rotation in three planes of each of the three bones. Second, cadaveric studies provide incomplete simulation of the contributions from the dynamic stabilizers. Third, while early experiments were performed using mechanical testing equipment, later experiments with electromagnetic tracking equipment have in several cases arrived at very different conclusions [32]. Fourth, forearm rotational position also alters laxity and joint reaction forces, with varus /valgus laxity in general increased in forearm pronation [33, 34], although the medial soft tissues are stressed more in pronation and the lateral soft tissues are stressed more in supination [5, 20, 25, 27, 35]. Finally, the relative contributions of each structure depend upon the deforming force applied, with many early studies applying nonphysiologic forces. As a result, even relatively elementary aspects of elbow biomechanics remain controversial.
Lateral Elbow Stability
As described by O’Driscoll and colleagues, the most common mechanism for dislocation of the elbow is rotation of the forearm on the humerus into valgus, extension, and external rotation as the forearm supinates off the humerus [36]. As this motion progresses tissue damage progresses from lateral to medial. The lateral collateral ligament complex first tears [37], then the anterior and posterior capsules tear, and finally the ulnar collateral ligament tears [36]. Depending upon the position of the arm and the energy of the trauma during the injury as well as the patient’s anatomy, the radial head and coronoid may also be fractured [38–40]. In 66 % of cases there are concomitant tears of the common extensors and in 50 % of cases there are concomitant tears of the anterior band of the ulnar collateral ligament [37]. Dislocation of the elbow without tearing the ulnar collateral ligament is theoretically possible with rotation around an intact ulnar collateral ligament although clinical dislocation without tearing of the ulnar collateral ligament is uncommon [36]. Finally, recent video evidence has suggested valgus may be more common than varus as a mechanism of injury [41].
The articular surfaces provide the majority of the stability to varus stress, supplying 55 % of stability in extension and 75 % in flexion [2]. Among the soft tissues, the lateral collateral ligament complex is the primary stabilizer of the ulnohumeral joint to varus stress [5, 8, 16, 35], with fascial bands within the extensor musculature (in particular the extensor carpi ulnaris, which has the best mechanical advantage) also playing a role resisting varus stress [5]. Clinically, residual lateral instability of the elbow is poorly tolerated because shoulder abduction places a varus stress across the elbow and thus many activities of daily living subject the elbow to varus stress (Fig. 2.2). By the same logic , an external fixation device applied to the lateral elbow for residual instability after instability repair protects the lateral collateral ligament complex by acting as a tension band, while offering relatively less protection to a medial repair or reconstruction [6].


Fig. 2.2
This clinical photograph demonstrates how activities of daily living subject the elbow to varus stress. In this image, a subject is picking a coffee cup up off of a table. The deltoid (straight yellow arrow) pulls through the arm as a lever against gravity on the cup (straight white arrow) to create a varus stress across the elbow (curved red arrow)
Lateral Collateral Ligament Complex
The lateral collateral ligament complex is classically thought to be composed of three portions: the lateral ulnar collateral ligament , the radial collateral ligament , and the annular ligament (Fig. 2.3) [5, 16, 42]. These structures are anatomically discrete from one another and from the overlying extensor musculature to a variable degree [5, 43]. Both the lateral ulnar collateral ligament and the radial collateral ligament arise anterior to the lateral epicondyle, with the radial collateral ligament becoming confluent with the fibers of the annular ligament while the lateral ulnar collateral ligament continues to the supinator ridge of the ulna (Fig. 2.4). The lateral collateral ligament complex was classically described to arise from the axis of rotation of the elbow, with the lateral ulnar collateral ligament being isometric (Fig. 2.5) [2, 42, 44]. However, this remains controversial as both a computer modeling study [45] and a cadaveric study [46] have suggested that the radial collateral ligament is isometric while the lateral ulnar collateral ligament is taut in flexion and loose in extension. The isometric point laterally has been identified 2 mm proximal to the center of the capitellum [45].




Fig. 2.3
This clinical photograph of a cadaveric dissection in which all structures aside from the humerus, radius, ulna, joint capsule , and ligaments have been removed demonstrates the lateral collateral ligament complex, including the lateral ulnar collateral ligament (solid box), annular ligament (dashed box), and radial collateral ligament (dotted box)

Fig. 2.4
This clinical photograph with the lateral collateral ligament complex removed demonstrates the attachment sites for the lateral ulnar collateral ligament (dashed boxes)

Fig. 2.5
This clinical photograph demonstrates that, in extension, the lateral ulnar collateral ligament (solid box) can be seen to be nearly isometric
Elbow instability is thought to occur in stages such that isolated tears of the lateral collateral ligament complex are possible, leading to a subluxation phenomenon called posterolateral rotatory instability (Fig. 2.6) [47, 48]. Posterolateral rotatory instability is a combination of external rotation/supination of the forearm on the humerus, axial loading, valgus angulation, and posterior displacement of the forearm on the humerus. Clinically, a tear in the lateral ulnar collateral ligament is thought to be the defining pathology to allow this subluxation [44, 48]. However, the Y-shaped configuration of the lateral ulnar collateral ligament and the radial collateral ligament may be structurally self-reinforcing therefore injury of only the lateral ulnar collateral ligament may not lead to gross instability. Cadaver studies have shown that isolated sectioning of either the lateral ulnar collateral ligament or radial collateral ligament is not sufficient to produce posterolateral rotatory instability and requires sectioning of both structures to create instability [18, 49]. Nevertheless, the relative importance of each portion of the lateral collateral ligament complex remains controversial. Olsen and colleagues found that sectioning of the annular ligament while leaving the lateral ulnar collateral ligament intact increased varus opening from 2–3° to 6–11°, suggesting that the lateral ulnar collateral ligament may not be the primary stabilizer and that the annular ligament, which was traditionally thought to be of relatively little importance for stability, may be functionally important [50]. In a sequential sectioning study, Olsen and colleagues found that the radial collateral ligament was the primary stabilizer and that the lateral ulnar collateral ligament was an accessory, while the annular ligament played essentially no role in stabilizing the lateral elbow [8]. Consequently, all components of the lateral complex probably play a role in lateral sided stability and repair of each component will likely maximize stability.


Fig. 2.6
This clinical photograph demonstrates that after release of the lateral collateral ligament complex (outlined in dots) from the humeral attachment (outlined with a dashed box) the ulnotrochlear joint gaps (black double–sided arrow) with a rotatory stress
Anatomically, the lateral soft tissues most commonly tear from the humeral origin resulting in tears of both the radial collateral ligament and lateral ulnar collateral ligament . Thus, the preceding debate regarding which portions of the ligament are most important for stability may not be clinically important as both are commonly injured as a unit (Fig. 2.7) [5, 16, 23, 35, 37]. In acute repairs, most surgeons attempt to gather tissue from both from the radial collateral ligament and lateral ulnar collateral ligament [39, 40]. In chronic instability , reconstruction of just the lateral ulnar collateral ligament leads to excellent functional outcomes and reliable restoration of stability [16, 39, 40, 48]. One cadaveric study demonstrated that reconstruction of the lateral ulnar collateral ligament provides equivalent stability to reconstruction of both the lateral ulnar collateral ligament and radial collateral ligament [17]. Thus, regardless of the controversy in cadaveric studies, the lateral ulnar collateral ligament appears to be the most critical part of the lateral collateral ligament complex to reconstruct.


Fig. 2.7
This clinical photograph demonstrates release of the humeral attachment (dashed box) of lateral collateral ligament complex (outlined in dots and gripped by the forceps), which is the most common anatomic location for ligament tears
Coronoid
The coronoid is a critical elbow stabilizer, acting as a buttress against axial loading, rotation, and posterior displacement of the ulna. The coronoid also serves to lengthen the articular surface of the sigmoid notch, improving flexion/extension range of motion. The coronoid is also relatively exposed to shear stress and is thus at risk for fracture. Up to 60 % of the anteromedial facet portion of the coronoid is unsupported by metaphyseal bone placing it at risk for fracture especially during varus posteromedial rotatory dislocations [38]. In one series of operatively treated elbow dislocations, 63 % patients had a coronoid fracture [37].
While all authors agree that the coronoid is an important stabilizer for the elbow, coronoid fracture height varies and the exact height necessitating operative fixation is controversial. One study suggested that loss of as little as 25 % of the coronoid can lead to subluxation of the ulnotrochear joint in midflexion in the absence of the radial head [2]. Another biomechanical study demonstrated that fracture of 40 % of the coronoid increased both varus laxity and internal rotation stress [51]. Still another study found that radial head excision and removal of 30 % of the coronoid could lead to a dislocation even with the medial and lateral ligamentous complexes intact [52]. With an intact radial head, the same study found that 50–70 % coronoid excision was necessary to create a dislocation [52]. Based upon these studies, the threshold that a coronoid surgical repair is indicated remains controversial. The threshold is likely 40–50 % based upon the biomechanical data for fractures in isolation, although it may be as small as 10–15 % depending upon the concomitant injuries and how effectively they can be addressed [38, 52]. As a result, coronoid fracture fixation is a critical portion of the surgical treatment of elbow instability and should be considered as a necessary component of most repairs to maximize stability [38–40]. In cases of instability with coronoid bone loss, several coronoid reconstruction techniques have been described including radial head autograft, olecranon autograft, iliac crest autograft, coronoid allograft, and prosthetic reconstruction emphasizing the importance of restoring the coronoid to achieve stability [51].
Radial Head and Capitellum
The radial head provides lateral stability through three mechanisms: (1) by acting as a buttress, (2) through the concavity compression mechanism, and (3) by tensioning the lateral ulnar collateral ligament . The radial head is commonly fractured in the setting of elbow instability and in general should be repaired or reconstruction in most cases to maximize stability. Radial head resection increases laxity in multiple directions [52]. In one series of operatively treated elbow dislocations, 58 % of patients had a concomitant radial head fracture [37].
Multiple biomechanical analyses have demonstrated the importance of the radial head in elbow stability . In a cadaveric study, Hotchkiss and colleagues demonstrated that the radial head contributes up to 30 % of stability to valgus torque/displacement in the setting of an intact ulnar collateral ligament [53]. Subsequent cadaveric studies have demonstrated that isolated radial head excision doubles the valgus laxity of the elbow [52] and increases rotatory laxity by up to 145 % [54]. The radial head is particularly important in association with a coronoid fracture—in one cadaveric study when both were absent subluxation occurred even with completely intact ligaments and radial head replacement alone could stabilize the elbow [54]. The mechanism of posterolateral rotatory instability has been suggested to require pathologic external forearm rotation. The radial head serves as a block to excess external rotation, as the anterior radial head must sufficiently externally rotate to clear the distal capitellum to result in posterolateral rotatory instability [55]. Thus even small radial head defects can play an important role in stability if they are inopportunely placed [55]. The capitellum likely plays a similarly important role—in one study after excision of the capitellum -valgus laxity increased 3.1° with active elbow flexion in pronation [56]. Nevertheless, other data supports no change in varus /valgus displacement after capitellar excision in the setting of intact ligaments [57].
In addition to stabilizing the elbow to varus and external rotation, the radial head may also act to tension the lateral collateral ligament complex, as, after excision of the radial head and sectioning of the lateral collateral ligament complex, restoration of both structures is necessary to completely restore elbow laxity [58].
Because the radial head acts as a physical block to dislocation, multiple studies have shown that monoblock radial head replacements provide significantly more stability than bipolar components [15, 59]. In a cadaver study of surgically fixated terrible triad injuries comparing monoblock and bipolar components, 16-fold more force was required to dislocate a monoblock component than a bipolar component [59]. A second, similar study showed that this effect was amplified by the status of the lateral collateral ligament complex and common extensor because the bipolar radial head not only allows posterior translation but it then tilts so that continued force on the radial head now resolves to contain a dislocating shear force vector [60]. Anatomic restoration of radial head height is critical both to restore stability and to avoid altered ulnotrochlear kinematics and accelerated capitellar chondrosis [61]. With excessive radial length the ulna tracks in varus and external rotation, while with inadequate length the ulna tracks in valgus and internal rotation [61]. Even fractures that only involve a portion of the radial head may be plagued by similar issues—in a cadaver study Shukla and colleagues demonstrated that fractures that involved only 30 % of the surface area of the radial head reduced subluxation force by 80 %, even if the fragment was retained but depressed 2 mm or retained but angulated 30°, presumably due to loss of the concavity-compression mechanism [26].
Medial Elbow Stability
The ulnar collateral ligament has three distinct sections: the anterior band, the posterior band, and the transverse band (Fig. 2.8) [19, 42, 62]. The anterior band of the ulnar collateral ligament has been described as isometric in some studies [19, 42, 46] and anisometric in others (Fig. 2.9) [63]. The posterior band is taut from 60 to 120° of flexion and can limit flexion in the stiff elbow and require release [19, 64]. In flexion, the anterior band of the ulnar collateral ligament serves as the primary stabilizer of the elbow to valgus stress (Fig. 2.10) [19, 23, 50, 53, 62, 64], with the radial head serving as a secondary stabilizer [32]. In full extension the anterior capsule and osseous congruity provide valgus stability to the elbow [53], and thus clinically this ligament must be tested in flexion [20]. The anterior band of the ulnar collateral ligament takes origin from the anterior inferior aspect of the epicondyle proximally and inserts on the sublime tubercle of the ulna distally (Fig. 2.11). The anterior band of the ulnar collateral ligament has a long, thin insertion on the sublime tubercle and the portion immediately adjacent to the articular surface is most critical biomechanically [65].





Fig. 2.8
This clinical photograph of demonstrates the ulnar collateral ligament, including the anterior band (solid box), posterior band (dotted box), and transverse band (dashed box)

Fig. 2.9
This clinical photograph demonstrates that, in extension, the anterior band of the ulnar collateral ligament (solid box) is nearly isometric

Fig. 2.10
This clinical photograph demonstrates that, after release of the ulnar collateral ligament (outlined in dots and grasped by the forceps) from the humeral attachment (dashed box), the ulnotrochlear joint gaps (doubled–sided arrow) to valgus stress

Fig. 2.11
This clinical photograph with the ulnar collateral ligament removed demonstrates the humeral (dashed box) and ulnar (dotted box) attachment sites for the ulnar collateral ligament
While the anterior band of the ulnar collateral ligament is the primary valgus stabilizer, the radial head is a secondary valgus stabilizer and can even contribute up to 30 % of the stability with an intact anterior band of the medial collateral ligament [19, 23, 32, 50, 53, 62, 64]. Thus in injuries that affect both structures, the radial head becomes an important stabilizer that must be surgically addressed [32, 61]. If radiocapitellar column length is not restored, the ulnar collateral ligament may not heal at an anatomic length, potentially resulting in chronic valgus instability [32, 61]. However, unlike lateral instability, valgus instability of the elbow can be achieved equally with either monoblock or bipolar radial head arthroplasties [32, 34].
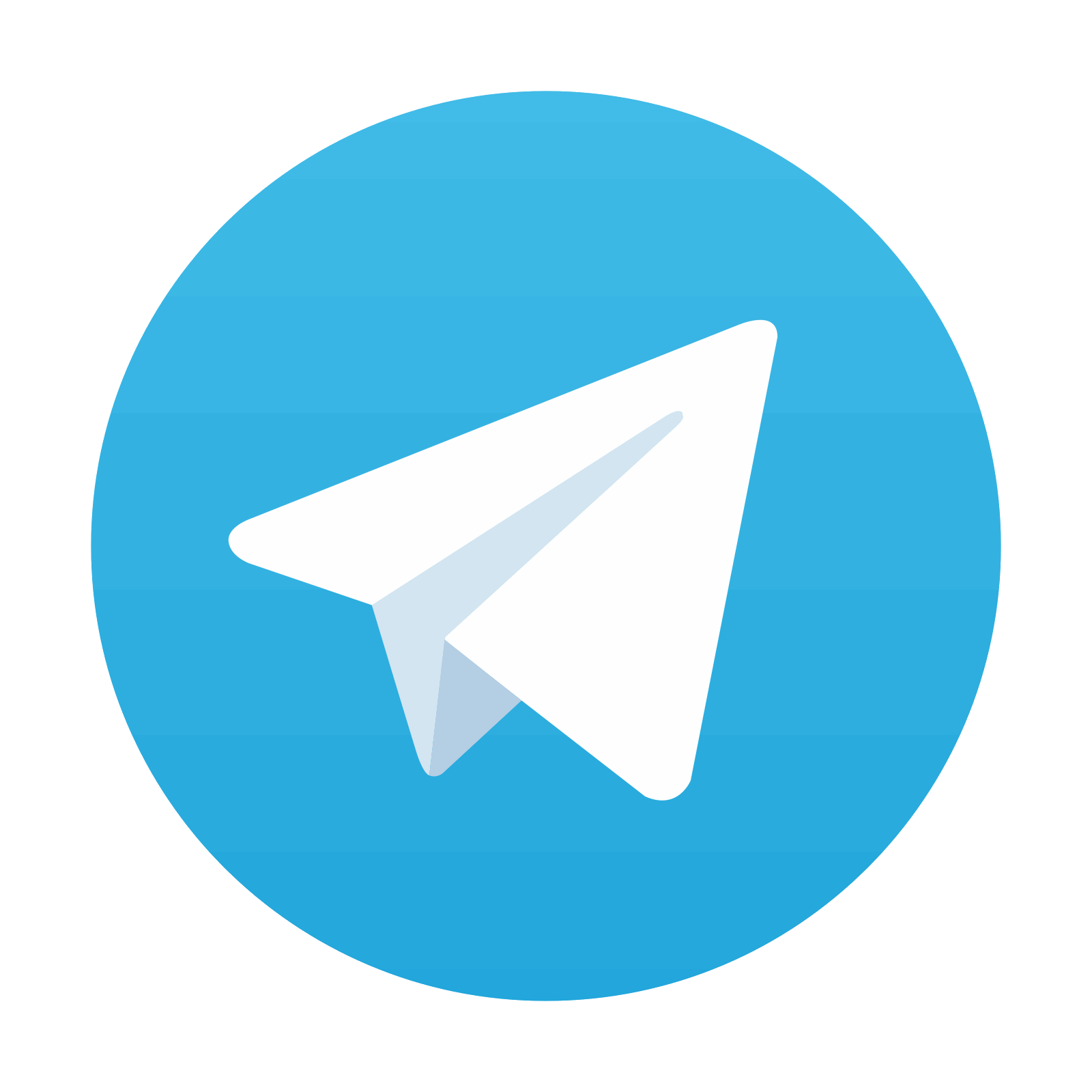
Stay updated, free articles. Join our Telegram channel

Full access? Get Clinical Tree
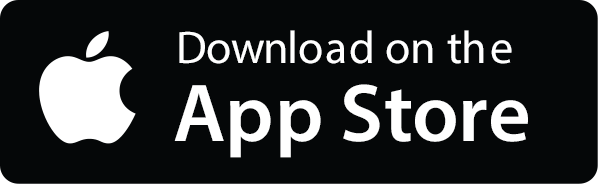
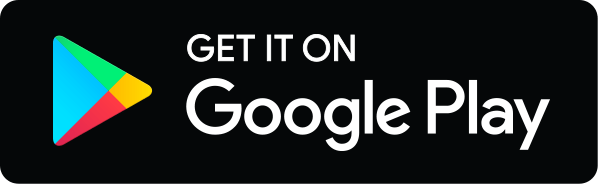