Degenerative changes in the material properties of nucleus pulposus and anulus fibrosus promote changes in viscoelastic properties of the whole disc. Volume, pressure and hydration loss in the nucleus pulposus, disk height decreases and fissures in the anulus fibrosus, are some of the signs of the degenerative cascade that advances with age and affect, among others, spinal function and its stability. Much remains to be learned about how these changes affect the function of the motion segment and relate to symptoms such as low back pain and altered spinal biomechanics.
The intervertebral disk has a composite structure consisting of a gelatinous proteoglycan-rich nucleus pulposus surrounded by a collagen-rich anulus fibrosus. The proteoglycan in the nucleus pulposus provides high water content within the nucleus pulposus and, in turn, contributes to sustaining large loads applied to the vertebral body. The load is distributed evenly to the anulus fibrosus through hydrostatic pressure. The fiber orientation of the anulus fibrosus is suitable to resist hoop stresses generated by the hydrostatic pressure in healthy conditions (see article by Grunhagen and colleagues elsewhere in this issue).
Degenerative changes in the biomechanical properties can occur in the nucleus pulposus and anulus fibrosus tissues individually. These changes can be shown as changes in material properties of each tissue. Degenerative changes in structural properties may be represented as consequences of these changes in material properties of the substructure of the disk. However, degenerative structural changes in the disk, such as loss of the volume of the nucleus pulposus and fissures in anulus fibrosus, can only be evaluated by analysis of structural parameters. It is important to understand how these changes affect the function of the motion segment and relate to symptoms such as low back pain (LBP) (see article by Karppinen and colleagues elsewhere in this issue).
This article discusses the degenerative changes in the material properties of nucleus pulposus and anulus fibrosus followed by the changes in structural properties of the entire disk, with an emphasis on the degenerative changes in viscoelastic properties of the whole disk. Instability of the motion segment as a consequence of the structural failure associated with the degenerative changes on the disk are also discussed. Instability of the lumbar spine, which has been considered to be one of the significant causes for mechanical LBP, is reviewed.
Material properties of the degenerative intervertebral disk components
Nucleus Pulposus
The disk degeneration process affects several of the structures differently, and apparently at different times during its progression. The impaired synthesis of the disk matrix involves all of its components at different time points. The process is believed to start in the nucleus pulposus, exhibiting a decrease in its proteoglycan concentration and gradual change in collagen type that transitions into a more fibrotic tissue. These factors effectively dehydrate the nucleus pulposus down from a peak nucleus water content in the adult disk of approximately 70%–80%. Recently, Murakami and colleagues quantified the difference in water content between old (3 years) and young (6 months) anulus fibrosus and nucleus pulposus tissue of rabbits, showing significant differences among them. In addition, the nucleus pulposus glycosaminoglycan (GAG), DNA, aggrecan, and collagen types I and II contents were significantly larger in the younger tissue. Evidence like this shows that the nucleus tissue is the most affected. Its decay constitutes one of the largest enablers of disk degeneration. This transition into a more fibrotic type of tissue produces a stiffer nucleus pulposus and the shock-absorbing properties of the disk are severely limited.
The nucleus pulposus, usually referred to as fluid, loses its hydrostatic pressure feature. A more fibrotic (increased collagen in nucleus pulposus) tissue does not behave in the same manner as a fluid/gel nucleus pulposus. The nucleus pulposus tissue undergoes a process of stiffening by means of gradual loss of proteoglycans and change of collagens from type II to type I, becoming a more fibrous and solid tissue, which was found to amalgamate into one solid phase with the anulus fibrosus in 75% of the cases from the sixth to eighth decade in a cadaveric study by Haefeli and colleagues. The loss of proteoglycans causes the decrease of swelling pressure in the nucleus pulposus, identified as the main load-bearing mechanism in the nondegenerate nucleus pulposus. As a consequence, load mechanics are altered and, for a period during the initial phase of degeneration, the disk is unstable.
Anulus Fibrosus
The mechanical behavior of the anulus fibrosus has been well documented in terms of tensile and compressive tests, but less so in shear. Tensile behavior corresponds with the circumferential direction on the annular wall and was characterized in static and dynamic tests to explain its mechanism to resist hoop stresses produced by the nucleus pulposus hydrostatic pressure. These two loading conditions are commonly accepted to simulate the body weight borne by the spinal column (compression) and the additional stresses seen in outward lateral bending and flexion/extension (tension). The greatest strengths are usually seen when loading the lamellae in the direction of the reinforcing fibers. The arrangement of the elastic fibers plays an important role in the overall mechanical properties of the anulus fibrosus. Elastic anisotropy in the anulus is maintained with degeneration, with posterolateral and outer lamellae regions having decreases of about 30% to 50% with advancing degeneration.
However, in cases like spondylolisthesis, anterior-posterior shear seems to be the dominant failure mode and this has not been studied, as well as shear within the anulus lamellae in cases of annular failure leading to herniation. In the degenerate anulus fibrosus, the fiber patterns become disorganized, and the elastic response also varies as a consequence. The elastic properties in an intact model are anisotropic and highly nonlinear. This nonlinearity, exhibited by a toe region on the stress-strain curves, is common to cartilaginous tissues. Moreover, the response of degenerate anulus fibrosus tissue has been shown to be of a twofold increase in the toe region modulus in tensile testing, which was correlated with age, as well as fiber realignment toward the loading direction. Dynamic viscoelastic testing has shown that the dynamic modulus of anulus fibrosus increases with degeneration at tensile strains greater than 6%. Earlier quasistatic test results from Acaroglu and colleagues described a strong influence of degeneration on elastic properties such as the Poisson ratio, failure stress, and strain energy density of the anulus fibrosus. The work by Guerin and colleagues reports no other significant changes in the elastic properties of the anulus fibrosus tissue.
In addition to elastic anisotropy, permeability also has been shown to vary spatially and is influenced by age, degeneration, and water content in the disk. These values have been incorporated in a finite element model simulation by Natarajan and colleagues, and they showed their effect on disk height and annular failure.
Degenerative changes in structural properties of the motion segment
The function of the motion segment is to provide the spine with axial stability while allowing mobility. The intervertebral disk is responsible for carrying enormous amounts of compressive loading while maintaining flexibility. The load on the disk is mainly compressive, but it is also subjected to other types of loads such as tensile and shear stresses. As the compressive load is subjected to the disk, hydrostatic pressure develops within the inner core of gelatinous nucleus pulposus, which pushes outward causing the outer ring of fibrous anulus fibrosus to bulge and experience tensile stress in the fibers. Loads on the lumbar disk (L3/4) of volunteers performing different body postures as well as disk pressures have been measured in vivo. These studies revealed that the load on the L3/4 level disk in a sitting position and in a standing position with 20° of flexion was 250% of the total body weight, although the portion of the body above the L3/4 level represented only 60%. Such large loads have been validated with mathematical models. This finding suggests that the load on the lumbar disk is composed of external and internal inputs. The external load is the weight of the body above the lumbar disk, and the internal load is the muscle force required to stabilize the spine in different postures. Increases in disk pressure should also be expected when a fluid is injected, as Andersson and Schultz showed when they inquired about the effects of injecting saline in a disk, and found varied responses in cases in which the injected fluid was retained, notably the large increases in pressure (up to 83%). In contrast, a decrease in pressure was observed in the degenerated disk.
Several animal models have been established to investigate degenerative changes in structural properties of the lumbar motion segment. A commonly used mechanical damage method to cause disk degeneration is the needle puncture or stab wound. Several researchers have recently arrived at the same conclusions when reporting that the diameter of the wound has to be large enough to create degeneration. Korecki and colleagues showed that, in an in vitro cyclic testing setting, bovine disks showed immediate and progressive differences in the dynamic modulus and stiffness of the anulus fibrosus tissue after puncture. Aside from the lamellar disturbances, cell viability and matrix remodeling were observed. Another animal model (ovine) of disk degeneration from induced lesions has also shown regeneration in the midwall of the anulus fibrosus. In a different loading condition, a murine tail model has also shown differences in the anulus fibrosus tissue as a consequence of dynamic compression, but did not achieve degenerate disk quality after long cycles of compression. These reports suggest that puncture injuries lead to degenerative remodeling including granulation tissue, which current image-based diagnostics methods might not be able to distinguish.
MacLean and colleagues investigated static viscoelastic behaviors of rat caudal motion segments, vertebrae, and isolated disk explants in different permeability conditions and showed that differences in endplate permeability conditions had a significant effect on the viscoelastic behaviors. Johannessen and colleagues showed a decrease in stress relaxation after 10,000 cycles of compressive loading in adult sheep lumbar motion segments and recovery of the stress relaxation after 18 hours of unloading in phosphate-buffered saline solution, suggesting intervertebral disk fluid transport during loading and unloading.
Boxberger and colleagues used a degenerative disk model in rat by injection of chondroitinase-ABC to the disks. In this model, nucleus pulposus degeneration has been successfully induced with GAG loss as a consequence of chondroitinase-ABC injection to the disks, which were tested in a linear viscoelastic tension/compression regime afterward. Results showed that the dynamic stiffness was decreased at low loads. Nucleus pulposus GAG content was shown to be related to the neutral zone properties in the tension-compression cyclic tests. However, the tension and compression extremes of the load displacement curve were not shown to be related. This finding shows that a degenerate nucleus produces hypermobility in addition to low pressures. Such distortion in load sharing leads to the development of hoop stresses in the anulus that resist compressive loads.
Kim and colleagues used a rabbit degenerative disk model with 18-gauge needle puncture of the disk to investigate changes in dynamic viscoelastic properties of the whole disk associated with the disk degeneration. In this model, the proteoglycan content decreased and collagen content increased 4 weeks after puncture. The dynamic viscoelastic test showed a decrease in elastic and viscous properties in the punctured disk ( Fig. 1 ). The correlation study showed that the proteoglycan content positively correlated with the elastic and viscous mechanical properties and height of the disk; however, there was no correlation with the collagen content. These results suggest that the proteoglycan is a governing factor for viscoelastic properties and structural properties of the disk.
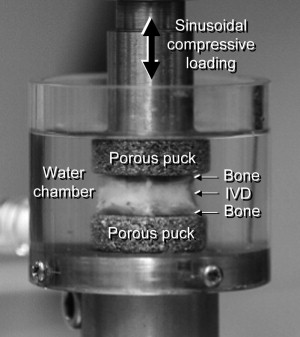
Using the same rabbit degenerative disk model and the dynamic viscoelastic testing method, Miyamoto and colleagues investigated the effects of OP-1 injection in the lumbar disk on the biomechanical and biochemical restoration of the disk. In this study, a significant increase in wet weight and proteoglycan content was observed in both nucleus pulposus and anulus fibrosus tissues of the OP-1-injected disks, compared with the lactose-injected control disks, whereas an increase in collagen content was observed only in the nucleus pulposus. These results suggested that increased proteoglycan content, induced by the injection of OP-1, resulted in tissue hydration in both the nucleus pulposus and anulus fibrosus. The results of the dynamic viscoelastic test showed that the elastic modulus has a significant positive correlation with the proteoglycan content in the nucleus pulposus and the proteoglycan and collagen content in the anulus fibrosus. Similarly, the viscous modulus was shown to have a significant positive correlation with the proteoglycan content in the nucleus pulposus and the proteoglycan and collagen content in the anulus fibrosus.
Degenerative changes in structural properties of the motion segment
The function of the motion segment is to provide the spine with axial stability while allowing mobility. The intervertebral disk is responsible for carrying enormous amounts of compressive loading while maintaining flexibility. The load on the disk is mainly compressive, but it is also subjected to other types of loads such as tensile and shear stresses. As the compressive load is subjected to the disk, hydrostatic pressure develops within the inner core of gelatinous nucleus pulposus, which pushes outward causing the outer ring of fibrous anulus fibrosus to bulge and experience tensile stress in the fibers. Loads on the lumbar disk (L3/4) of volunteers performing different body postures as well as disk pressures have been measured in vivo. These studies revealed that the load on the L3/4 level disk in a sitting position and in a standing position with 20° of flexion was 250% of the total body weight, although the portion of the body above the L3/4 level represented only 60%. Such large loads have been validated with mathematical models. This finding suggests that the load on the lumbar disk is composed of external and internal inputs. The external load is the weight of the body above the lumbar disk, and the internal load is the muscle force required to stabilize the spine in different postures. Increases in disk pressure should also be expected when a fluid is injected, as Andersson and Schultz showed when they inquired about the effects of injecting saline in a disk, and found varied responses in cases in which the injected fluid was retained, notably the large increases in pressure (up to 83%). In contrast, a decrease in pressure was observed in the degenerated disk.
Several animal models have been established to investigate degenerative changes in structural properties of the lumbar motion segment. A commonly used mechanical damage method to cause disk degeneration is the needle puncture or stab wound. Several researchers have recently arrived at the same conclusions when reporting that the diameter of the wound has to be large enough to create degeneration. Korecki and colleagues showed that, in an in vitro cyclic testing setting, bovine disks showed immediate and progressive differences in the dynamic modulus and stiffness of the anulus fibrosus tissue after puncture. Aside from the lamellar disturbances, cell viability and matrix remodeling were observed. Another animal model (ovine) of disk degeneration from induced lesions has also shown regeneration in the midwall of the anulus fibrosus. In a different loading condition, a murine tail model has also shown differences in the anulus fibrosus tissue as a consequence of dynamic compression, but did not achieve degenerate disk quality after long cycles of compression. These reports suggest that puncture injuries lead to degenerative remodeling including granulation tissue, which current image-based diagnostics methods might not be able to distinguish.
MacLean and colleagues investigated static viscoelastic behaviors of rat caudal motion segments, vertebrae, and isolated disk explants in different permeability conditions and showed that differences in endplate permeability conditions had a significant effect on the viscoelastic behaviors. Johannessen and colleagues showed a decrease in stress relaxation after 10,000 cycles of compressive loading in adult sheep lumbar motion segments and recovery of the stress relaxation after 18 hours of unloading in phosphate-buffered saline solution, suggesting intervertebral disk fluid transport during loading and unloading.
Boxberger and colleagues used a degenerative disk model in rat by injection of chondroitinase-ABC to the disks. In this model, nucleus pulposus degeneration has been successfully induced with GAG loss as a consequence of chondroitinase-ABC injection to the disks, which were tested in a linear viscoelastic tension/compression regime afterward. Results showed that the dynamic stiffness was decreased at low loads. Nucleus pulposus GAG content was shown to be related to the neutral zone properties in the tension-compression cyclic tests. However, the tension and compression extremes of the load displacement curve were not shown to be related. This finding shows that a degenerate nucleus produces hypermobility in addition to low pressures. Such distortion in load sharing leads to the development of hoop stresses in the anulus that resist compressive loads.
Kim and colleagues used a rabbit degenerative disk model with 18-gauge needle puncture of the disk to investigate changes in dynamic viscoelastic properties of the whole disk associated with the disk degeneration. In this model, the proteoglycan content decreased and collagen content increased 4 weeks after puncture. The dynamic viscoelastic test showed a decrease in elastic and viscous properties in the punctured disk ( Fig. 1 ). The correlation study showed that the proteoglycan content positively correlated with the elastic and viscous mechanical properties and height of the disk; however, there was no correlation with the collagen content. These results suggest that the proteoglycan is a governing factor for viscoelastic properties and structural properties of the disk.
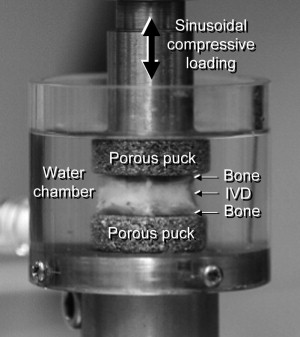
Using the same rabbit degenerative disk model and the dynamic viscoelastic testing method, Miyamoto and colleagues investigated the effects of OP-1 injection in the lumbar disk on the biomechanical and biochemical restoration of the disk. In this study, a significant increase in wet weight and proteoglycan content was observed in both nucleus pulposus and anulus fibrosus tissues of the OP-1-injected disks, compared with the lactose-injected control disks, whereas an increase in collagen content was observed only in the nucleus pulposus. These results suggested that increased proteoglycan content, induced by the injection of OP-1, resulted in tissue hydration in both the nucleus pulposus and anulus fibrosus. The results of the dynamic viscoelastic test showed that the elastic modulus has a significant positive correlation with the proteoglycan content in the nucleus pulposus and the proteoglycan and collagen content in the anulus fibrosus. Similarly, the viscous modulus was shown to have a significant positive correlation with the proteoglycan content in the nucleus pulposus and the proteoglycan and collagen content in the anulus fibrosus.
Instability of the motion segment associated with intervertebral disk degeneration
As disk degeneration progresses, structural failure of the disk is manifested by tears and clefts in the anulus fibrosus. These material disruptions occur in different directions and are the result of a variety of influencing factors, including altered loading of the disk. Potential relationships between osteophytes and peripheral tears were first reported by Schmorl and Junghanns, and also highlighted that, because of the tears, segmental instability would be affected. Farfan and Kirkaldy-Willis concluded that tears were by-products of torsional stresses, implicating them as initiators of the failure of other disk components in the disk degeneration cascade.
Disk fissures have been classified in 3 categories, depending on their morphology and anatomic position in the disk: (1) peripheral tears or rim lesions, parallel to the endplates and exhibiting normal separation of the disk from the subchondral bone of the vertebral body, which, with time, developed (2) circumferential tears that present evidence of delamination as a failure mode; and (3) radial tears that, as the name implies, propagate in a direction perpendicular to an imaginary axis of the disk (if it is considered as a flat cylinder), which usually lead to disk herniations and expulsion of nucleus material. The literature shows few reports that address the crack propagation phenomena involved in disk tears, because it is common to report only the resulting condition (ruptured anulus, herniated disk). Many of the models consider the anulus as a bulk material but, recently, more advanced models have incorporated annular layers and implemented permeability and porosity, as well as the disk’s osmoviscoelastic properties. The interlamellar structures have been deemed especially sensitive to shear stresses, and the literature is lacking reports of their allegedly weaker mechanical properties. They are believed to play a predominant role when destructive processes such as delamination occur as part of herniation, as has been attempted in analytical models of the disk, of anulus fibrosus tissue, and of individual lamellae. Schollum and colleagues recently analyzed in detail the interface between annular lamellae in an ovine model by subjecting thin slices of immature and mature anulus fibrosus tissue to microtensile tests. Although their studies mostly described the architecture of the interlamellar interface, important differences in the response to tensile forces were shown between young and old tissue, with the older tissue exhibiting a more ordered and uniform lamellar separation than the young tissue; however, the investigators did not report elastic properties.
Fujiwara and colleagues studied the effect of disk degeneration graded by magnetic resonance imaging on the segmental motion of the lumbar spine using a total of 106 motion segments obtained from 44 cadaveric lumbar spines taken from 18 women and 25 men with a mean age of 69 years. The investigators found that segmental motion increased with increasing severity of disk degeneration to grade 4, but decreased when the disk degeneration advanced to grade 5. Such segmental motion changes were greater in axial rotation compared with those in lateral bending, flexion, and extension, showing the importance of torsional instability in diagnosing spinal instability. The results of these studies are important for understanding the kinematic property changes in relation to the types or grades of disk degeneration. The results were consistent with the previous reports and the concept of 3 stages of spinal degeneration: dysfunction, instability, and restabilization proposed by Kirkaldy-Willis and Farfan.
Instability of the lumbar spine associated with intervertebral disk degeneration
Segmental instability of the lumbar spine is frequently considered a cause of LBP, but instability of the spine is poorly defined and understood. The basic concept of spinal instability is that excessive motion beyond normal constraints causes either compression or stretching of the neural elements or causes abnormal deformations of ligaments, joint capsules, annular fibers, or endplates, which are known to have a significant number of nociceptors. Even though several studies have indicated that excessive motion on flexion/extension radiographs is associated with LBP or degenerative disk disease, other studies cite decreased motion in patients with degenerative changes and such pain.
Lumbar segmental instability may be associated with a spectrum of clinical manifestations of degenerative changes in the intervertebral disk. Intervertebral disk degeneration has been studied using magnetic resonance (MR) imaging, and grades of degeneration have been reported. The relationship between the types (or grades) of disk degeneration and kinematic characteristics of the motion segment has been studied using cadaveric spinal motion segments. Despite some variation in results, likely because different loading conditions and methods of grading degenerative disk changes were used, the overall results of these studies indicate that the biomechanical characteristics of the motion segment can become altered significantly when degenerative changes develop in the intervertebral disk.
In vivo Measurement of Lumbar Segmental Movement
There have been numerous in vivo studies on segmental instability of the lumbar spine in which dynamic flexion/extension radiographs were used. However, these dynamic radiographic techniques have been found to be inaccurate. The errors associated with sagittal plane translational motion measurement reported in the literature range from 1 to 4 mm, or 3% to 15% of the vertebral depth. Schaffer and colleagues reported high false-positive and false-negative rates (ie, normal translations are categorized outside of the normal range and vice versa) with significant differences between measurement methods despite high reliability across radiographic quality, raters, and measurement. More sophisticated techniques, such as biplanar stereoradiography, centrode pattern analysis, and traction-compression radiography, have been introduced but have not been widely accepted. More accurate methods involve invasive techniques by inserting metal beads or spinous process wire to determine three-dimension (3-D) motion. However, because these methods are invasive, they are not appropriate for routine use in clinical practice or for in vivo human studies. Studies on segmental instability have also been limited by other factors in addition to these problems associated with accurate measurement of segmental motion in vivo. For example, the range of motion measured in most of these studies is affected by the variability in voluntary efforts that the subject applies at the time of examination and can also be limited because of pain.
Other two-dimensional (2-D) imaging methods for measuring axial rotation, as opposed to flexion/extension, have involved MR imaging of subjects in various rotated positions. Although these studies were noninvasive and controlled for voluntary motions, they could only determine changes in segmental motion around 1 axis. It has been suggested that coupled motions could play an important role in determining spinal instability. To measure these coupled motions, studies have been conducted to measure 3-D motions in vivo. More invasive techniques involve inserting wires into the spinous process of subjects to determine 3-D motion. Although this method has proved more accurate than radiographs, its invasive nature limits its widespread clinical use. Other studies have used biplanar radiography, in which the radiograms of the spine were taken from 2 directions simultaneously and 3-D motions are calculated by the positions of anatomic landmarks in corresponding images. There has been some concern about the accuracy in determining anatomic landmarks for biplanar radiography, as well as a lack of equipment for this method in typical clinical settings.
To overcome some of these limitations to 3-D motion measurement, Lim and colleagues developed a 3-D imaging technique using dynamic computed tomography (CT) to determine 6-degree-of-freedom (3 rotations and 3 translations) transformation of individual cadaveric cervical vertebrae during motion by tracking eigenvectors of the individual vertebrae. The investigators showed that accurate measurements (±1 mm and ±1°) can be made using CT in vitro. The research group expanded on this technique to measure vertebral segmental movements in human lumbar spines in vivo ( Fig. 2 ). Although this method was able to determine the rotations and translations of the lumbar vertebrae during motion in vivo, it was limited in determining transformation of the sacrum because an entire 3-D CT model of a sacrum is difficult to obtain clinically and the eigenvector analysis using a partial-sacrum 3-D model caused an error of the measurement of the segmental motion at L5 to S1. The same research group developed another method to determine transformation of individual vertebrae including the sacrum during motion using the 3-D CT model and a volume merge method ( Fig. 3 ). This method can determine the rotations and translations during motion even if the 3-D geometry of the bone is incomplete, as in the case of the sacrum (an assumption of the bone as a rigid body still holds) at each position. Thus, it is able to determine the transformation of the incomplete sacrum 3-D CT model with an accuracy of less than 0.1 mm in translation and 0.2° in rotation.
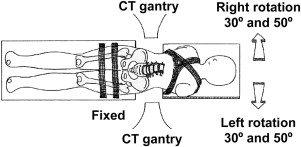
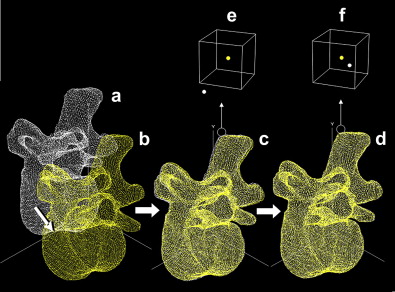
Relationship Between Instability and Disk Degeneration
Most patients with segmental instability have disk degeneration, but the relationship between instability and degeneration is not clear. Takeuchi and colleagues presented a study using MR images in which T1 relaxation time was decreased in degenerative disks and the energy dissipated to axial loading was linearly correlated with T1 relaxation time. The investigators attempted to correlate the intrinsic biomechanical properties of the disk with MR imaging findings, but no information could be derived about the segmental motion characteristics from this study. Toyone and colleagues reported that bone marrow adjacent to the disk in patients with symptomatic lumbar segmental instability, defined by flexion more than 5° and dynamic anterior-posterior translation more than 3 mm, had decreased signals on T1-weighted spin-echo MR images or Modic type I changes. Inaccurate flexion/extension radiographs of patients were used in this study by Toyone and colleagues and pathogenesis of the osseous changes with disk degeneration is not known.
Results of the in vitro studies of segmental motion characteristics and disk degeneration done by Fujiwara and colleagues showed that torsional motion was most significantly affected by the degenerative changes in disk and facet joints. In addition, some investigators advocate the importance of torsional loads and stability on the injuries and degeneration of the motion segments. Torsional instability in relation to the degenerative changes in the disk had been investigated in vivo using the aforementioned in vivo 3-D measurement technique. The investigators found that a relationship exists between the severity of intervertebral disk degeneration and increases in the torsional movement in vivo, which was previously shown only in cadaveric studies.
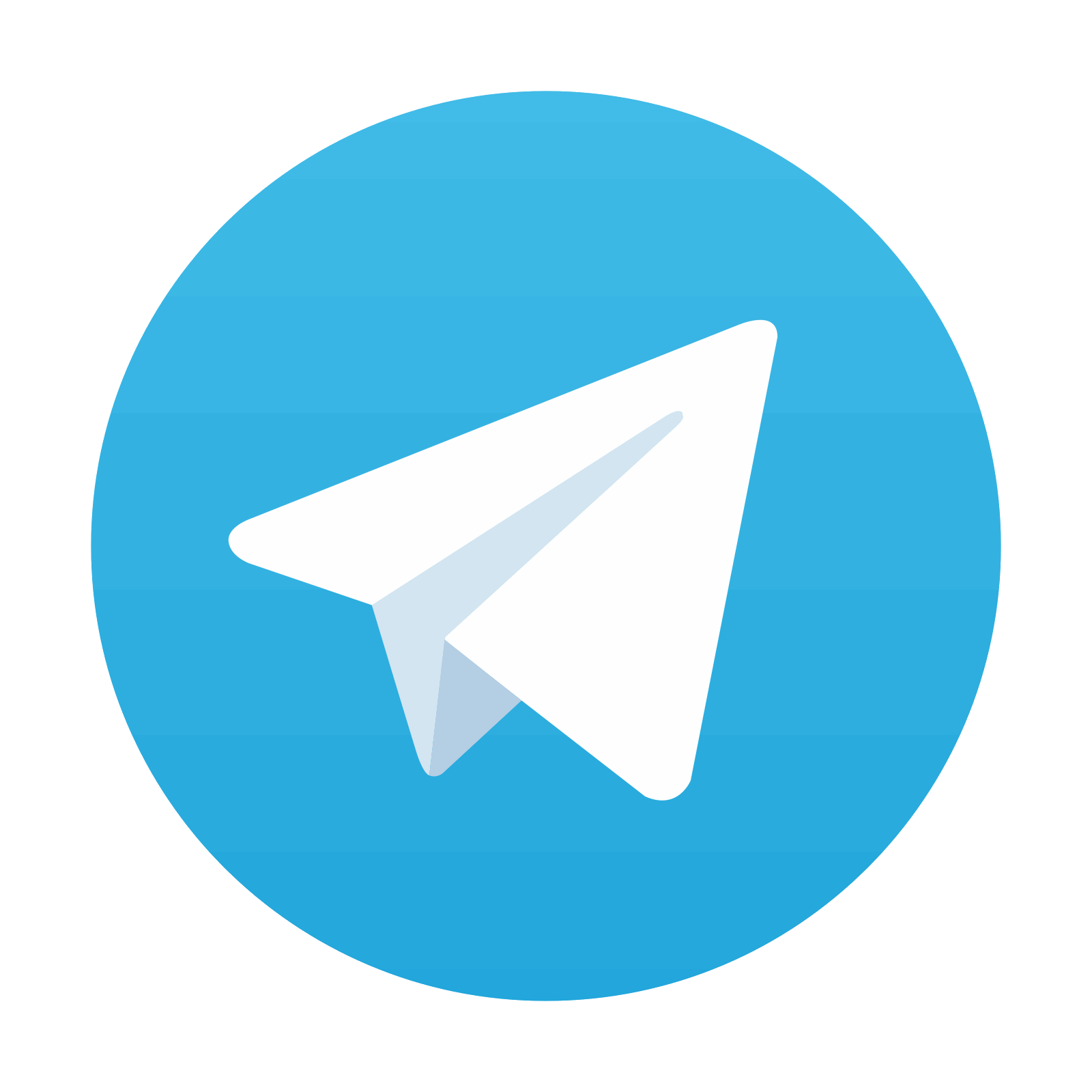
Stay updated, free articles. Join our Telegram channel

Full access? Get Clinical Tree
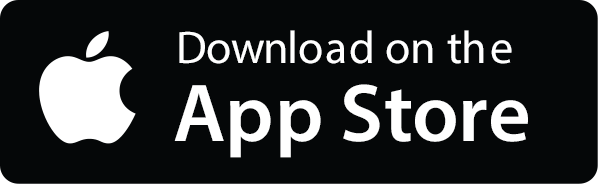
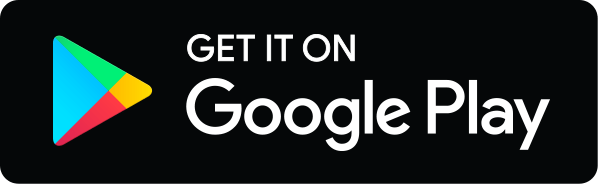