© Springer-Verlag London 2016
Dominique G. Poitout (ed.)Biomechanics and Biomaterials in Orthopedics10.1007/978-1-84882-664-9_2525. Biomechanics of Fracture in Growth Period
(1)
Department of Rheumatology, Zama General Hospital, Zama, Kanagawa, Japan
(2)
Department of Orthopaedic Surgery, Showa University Fujigaoka Hospital, Yokohama, Kanagawa, Yokohama, Japan
Keywords
Fractures in childrenChildren fracturesPediatric fracturesBone growth in childrenGrowth plate and epiphysis fracture in childrenCharacteristics of Fractures in Children
Management of fractures in children is completely different from that of adults. Biological reaction for fracture is characteristic in children because of the anatomic, physiological, and biomechanical property of skeletal structure [1]. Comprehension of the properties of children’s bone is essential to treat fractures in children. Some properties act favorably for the bone union and some make it difficult to treat fractures.
The Role of Periosteum
The detailed biomechanics of periosteum are described in Chap. 31. Periosteum in children’s bone is far thicker than that of adults and thus much callus would appear early after the injury. This is one of the reasons why union of fracture is obtained easily in children. High activity and rich blood supply in the thick periosteum affect new bone formation. The new bone formation in children occurs so immediately after the injury that reduction of the displacement, if necessary, should be achieved in early stages. The periosteum in children’s bone is stronger than that of adults [1]. It is well known that the connection between metaphysis and epiphysis is very strong. This strong connection is brought about by the strength of the periosteum. When bending stress is applied to the pediatric bone, the periosteum would be ruptured only in the convex side but not in the concave side. The periosteum in the concave side could act as “intact hinge”. This intact hinge is helpful to reduce the position and to maintain the reduced position. On the other hand, if the displacement is not reduced in the early stage, the gap between the exfoliated periosteum and the bone would be filled by new bone and severe deformity would remain [2]. This phenomenon is often observed in unreduced supracondylar fracture of the humerus or the radius distal fracture.
Characteristics of Children’s Bone
Bone density of children is lower than that of adults [3]. Bone porosity of children is higher than that of adults. Most of the bone cortex in children is occupied by Haversian canals [4]. Because of both the structural properties of bone and the thick periosteum, the whole bone unit in children has more endurance against stress (e.g., compressive stress, tensile stress, bending stress) than that of adults. Currey made an experimental study concerning the strength of the femur in children. Compared with adults, children had a lower modulus of elasticity, a lower bending strength, and a lower ash content. However, the children’s bone deflected more and absorbed more energy before breaking. It absorbed more energy also after fracture had started. The typical greenstick fracture surface of many specimens of children’s bone requires more energy for its production than the smooth surface of adult specimens [5]. Even when a fracture occurs in child bone, the fracture line is simple and is rarely accompanied by severe displacement. Most fractures in children are incomplete fractures in which the partial continuity of bone remains (i.e., subperiosteal fracture). When bending stress beyond the physiological tolerance is given to a bone, angular deformity of fracture occurs less often in children than in adults. In such cases, greenstick fracture more likely occurs in children. This fracture is often observed especially in distal third of the radius. When axial compressive stress is given to the bone, bamboo or buckle fracture often occurs at metaphysis, where the maximum porosity exists. Otherwise, a plastic deformation may occur [6]. The plastic deformation often observed in pediatric long bone fracture which is due largely to the complex nature of the molecular and histologic aspects of pediatric bone. Pediatric cortical bone has a lower mineral content than adult bone, accounting in part for its different material properties. Although plasticity allows children’s long bones to absorb more energy before fracture, a significant deformity may persist after injury [7, 8].
Epiphyseal Injury
Fracture of the growth plate is an injury unique to childhood. The prevalence of this injury in the whole fractures in children is 10–30 % [9]. Salter classified this injury into five groups by the verosity of stress on the epiphyseal plate and direction stress distribution at the plate, and the prognosis: type I, fracture through the growth plate; type II, fracture through the growth plate and metaphysis; type III, fracture through the growth plate and epiphysis; type IV, fracture through the growth plate, epiphysis and metaphysis, and type V, crush or compression injury of the growth plate [2]. Ogden proposed a new classification scheme of physeal and epiphyseal injuries to allow better estimation of prognosis for normal or abnormal growth. This classification based partially on the Salter-Harris system, but additionally detail subclassifications that relate to specific injury patterns [10]. Epiphyseal fracture often occurs in the metaphyseal side of the epiphysis and most of the epiphyseal cartilage cells remain in the epiphyseal side. Most such fractures heal without permanent deformity. A small percentage, however, has subsequent complications such as aseptic necrosis of epiphysis, non-union, premature epiphyseal closure (i.e., growth arrest), joint deformity (angulation, rotation) due to partial epiphyseal closure, and unequal length of leg or arm. The growth-plate has three-dimensional unevenness, which has been confirmed by scanning electron microscopic studies. This unevenness gives the growth-plate a multi directional endurance against compression, strain, and shear stress. The yield strength of a growth-plate depends on the degree of this three-dimensional unevenness [11, 12]. Reported yield strength of the growth-plate has varied as it depends on the material of the experiment [13]. According to Bright, the strength of the force which made a crack in the growth plate was one-half of the yield strength of the growth-plate. On the other hand, Bright and Nakada reported that no relation existed between these two strengths [12, 14]. Kim pointed out that the strength depends on the existence of the membrane of cartilage [11]. The membrane seems to reinforce the growth-plate in cooperation with the three-dimensional structure of the growth-plate. In his report, the ratio of fracture strain between the growth-plate without / with cartilage membrane was 61 % (tensile strength) and 37 % (strain strength). Amamilo reported that the periosteum contributed significantly to the stiffness of the epiphyseal plate [15]. The hypertrophying and calcifying layer is more fragile than the resting and proliferative layer. However, the section where the crack in the epiphyseal injury existed varies between authors. Bright reported that the crack was not observed only in the hypertrophying layer but also in the resting and the proliferative layer [14]. Nakada reported that crack existed mainly between the proliferative and hypertrophying layer [12]. Amamilo reported that the crack existed in the calcifying layer [15].
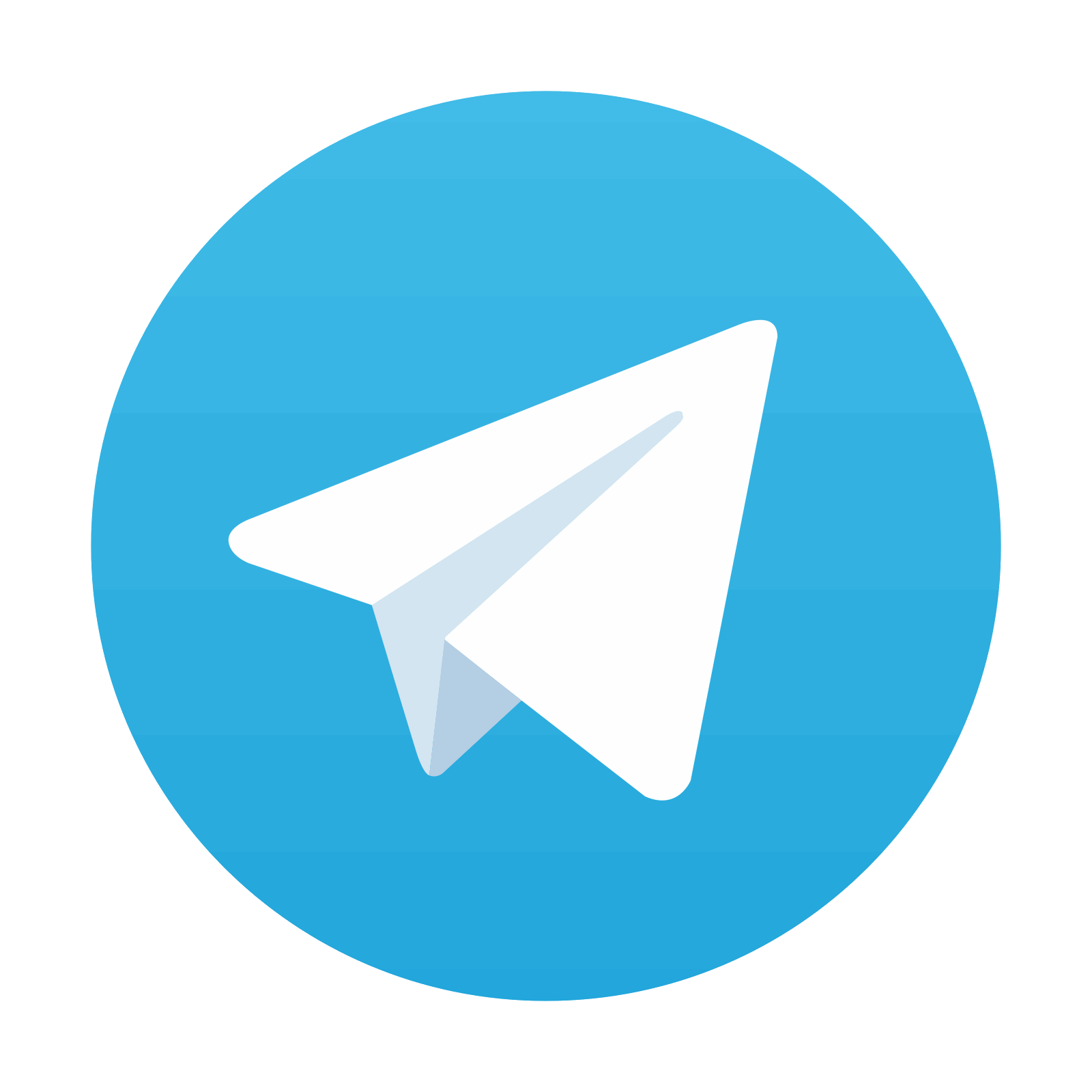
Stay updated, free articles. Join our Telegram channel

Full access? Get Clinical Tree
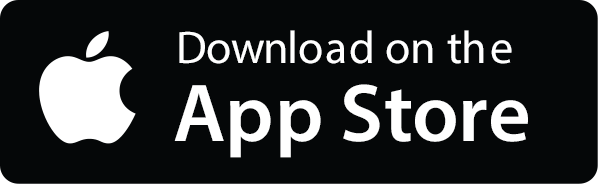
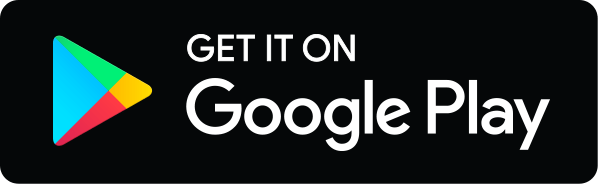