Walking
Running
Ground reaction forces
1.5 × body weight
2–3 × body weight
Velocity
Lesser
Greater
Ranges of motion
Lesser
Greater
Support phase
Single and double limb support
Single limb support only
Greater time spent in stance
Lesser time spent in stance
Swing phase
One limb at a time
Overlap between limbs (period of “float”)
Center of gravity
Higher
Lower
Base of support
Wider
Narrower
Muscular demands
Lesser
Greater
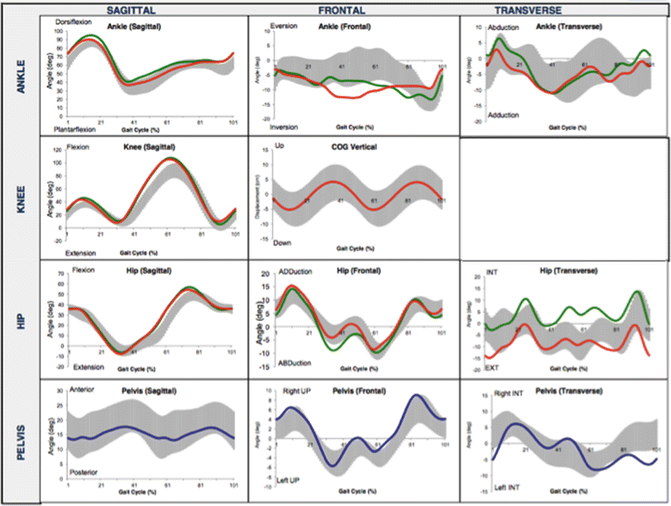
Fig. 3.1
Normal kinematic curves of the lower extremity during running. Courtesy of University of Florida Health Sports Performance Center, Running Analysis
There are three main phases of running: stance, swing, and float. As running speed increases, such as when a runner transitions from jogging to sprinting, the time spent in the stance phase decreases. The first half of the support phase is accountable for the proper force absorption and attenuation, with the second half more responsible for preparing the limb for forward horizontal propulsion. The support phase begins as the foot contacts the ground. A synchronous coordination of movement, starting from the interaction between the ground and the foot and continuation up the kinetic chain, is vital to healthy running biomechanics (Table 3.2). At this time, the process of force dissipation, or “pronation,” is required. Pronation throughout the lower leg is comprised of subtalar joint eversion, forefoot abduction, talocrural joint dorsiflexion, and tibia internal rotation. Continuing up the kinetic chain, the knee then progresses into a more flexed, internally rotated, and valgus position. The hip will subsequently flex, adduct, and internally rotate and precede pelvic elevation and anterior rotation. Ultimately, the lumbosacral spine will extend and laterally flex towards the stance limb. During the second part of the stance phase, the body will prepare for eventual limb unloading and swing phase, requiring the foot to re-supinate and become more rigid [16]. Due to the complex nature of the running motion, it is imperative that an individual working with the running athlete have a keen understanding of normal running biomechanics.
Table 3.2
Lower kinetic chain biomechanics
Pronation | Supination | |
---|---|---|
Lumbopelvic | Ipsilateral side bending | Contralateral side bending |
Contralateral pelvis drops | Contralateral pelvis raises | |
Anterior innominate rotation | Anterior innominate rotation | |
Hip | Flexion | Extension |
Adduction | Abduction | |
Internal rotation | External rotation | |
Knee | Flexion | Extension |
Abduction | Adduction | |
Internal rotation | External rotation | |
Ankle | Dorsiflexion | Plantarflexion |
Internal rotation | External rotation | |
STJ | Talus adduction | Talus abduction |
Talus plantarflexion | Talus dorsiflexion | |
Calcaneal eversion | Calcaneal inversion | |
MTJ | Dorsiflexion | Plantarflexion |
Abduction | Adduction | |
Inversion | Eversion |
Static Biomechanical Assessment
A thorough biomechanical assessment is an important aspect of the management and prevention of stress fractures. Intrinsic biomechanical properties have been implicated as possible contributing factors not only in stress fractures but also in other overuse injuries of the lower extremities. Though results are conflicting, it has been hypothesized that the structure and alignment of the lower extremity may influence the body’s ability to control the forces associated with high impact activities [17]. Though there are many possible structural irregularities within the kinetic chain, specific structural characteristics have been linked with stress fractures and should be addressed. It is recommended that a static biomechanical assessment be performed in conjunction with a kinematic assessment to allow for a complete picture of the mechanics contributing to injury.
Foot Type Classification
Various methods of classifying foot structure have been presented in the literature, including, but not limited to, the Foot Posture Index [18, 19] and longitudinal arch angle [20, 21]. Classification of foot type attempts to distinguish between the static postures of a hyper-pronated, supinated, or neutrally aligned foot (Fig. 3.2). Though static foot structure has been postulated to contribute to pathology, results in the literature are conflicting [17, 22–24]. A hyper-pronated, low-arched foot is thought to contribute to excessive torsional forces about the tibia and strain on lower extremity musculature, as increased eccentric demands are required to control excessive motion. Conversely, a supinated or high-arched foot is thought to contribute to excessive stress on the bony architecture due the decreased ability to adequately absorb and dampen ground reaction forces [25].
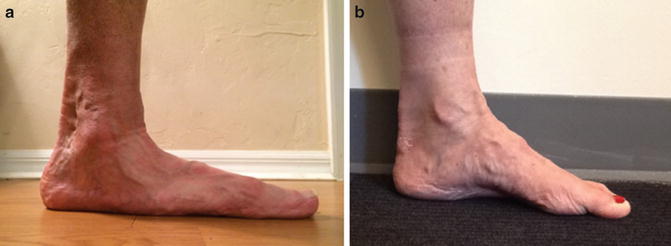
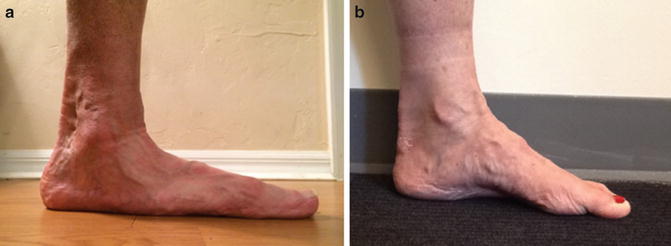
Fig. 3.2
(a) Low-arched, pronated foot type; (b) high-arched, supinated foot type
Other Structural Links to Stress Fracture
Aside from foot structure, other lower extremity biomechanical characteristics should also be assessed. Structural leg length inequality seems to have the most evidence regarding its relationship to lower extremity stress injuries. It is believed that differences in side to side leg length may lead to altered lower extremity loading patterns, however preference as to the risk of injury on either the longer or shorter limb has not been consistently established [17, 24, 26–28]. Excessive forefoot, rear foot or tibial varus, q-angle, tibial torsion, and genu varus, valgus, or recurvatum are all thought to be factors contributing to tibial stress fractures, though the evidence about the true extent is conflicting [5, 16, 29]. Until research is able to more clearly and consistently demonstrate the contribution of static biomechanical measures to overuse injury, attention should be placed at any identified abnormalities.
Running Gait Analysis
The role of formal gait analysis is to determine whether a relationship exists between an individual’s abilities and limitations and their individual gait pattern, to either enhance performance or assist with injury management [30]. Similarly to the evaluation of walking, running gait can be analyzed along a continuum ranging from real-time observational inspection, video motion analysis, or with the use of more extensive measurement devices and instrumentation. Analyses can be further performed by observing the runner while running on a treadmill or over level ground. A treadmill is often utilized as a means of gathering information from various angles while easily capturing successive foot strikes. However, there is conflicting evidence regarding whether running on a treadmill mimics the conditions of over ground running [31–37]. Provided the treadmill has limited intra-stride length variability [38], a sufficiently stiff surface [34, 38], and a belt speed that is adequately regulated [34], running on a treadmill can be generalized to over ground environments [37]. Conversely, it has also been concluded that increasing running speed on a treadmill is mechanically different from that while running over ground [35, 36]. Despite these claims, instrumentation may be setting dependent, though it is advisable to perform the analysis in the conditions in which the runner routinely trains.
Observational Gait Analysis
Observational gait analysis is the most widely utilized and least expensive form of running analysis. It is a gross analysis where the examiner will directly observe a runner with the naked eye in real-time. Attention is placed on the movements associated within each phase of the running cycle and is monitored in each of the three cardinal planes of motion. Anatomical markers may be placed on specific bony landmarks to assist with observation of body segments, and is especially beneficial when looking at more subtle movements, such as rotation in the transverse plane.
Due to the high limb velocities associated with running, it is difficult to examine specific amounts of motion or the simultaneous coordination of multiple joint segments of the lower kinetic chain [39]. Therefore, each joint or anatomical region is individually observed and examined in accordance with period of the running phase. Usually, observation of the loading phase of stance follows a “bottom up” approach, identifying the lower extremity’s ability to pronate first at the foot and ankle, followed by progression up the lower kinetic chain. Conversely, when evaluating the later phase of stance, which focuses on the ability of the lower extremity to supinate, an observer can utilize a “top down” methodology [15].
Motion Analysis
The use of a video capture device will help enhance the accuracy of observational analysis. It will further allow a more quantitative description of gait parameters in relation to joint kinematics, however lacks the ability to measure associated forces acting upon the body. Motion capture is helpful to observe the movements and positions of multiple joint segments simultaneously, and assist with identifying other characteristics of gait including cadence, step length, stride length, and running speed [15]. To maintain a level of consistency and accuracy with measuring joint angles and positions within and between assessments, the marking of certain bilateral anatomical landmarks is advised (Fig. 3.3). A typical marker setup may include the anterior superior iliac spine (ASIS), posterior superior iliac spine (PSIS), greater trochanter, lateral femoral condyle, tibial tubercle, knee joint line, bisection of the posterior calcaneus, and second ray. Tight fitting clothing with increased exposure of the skin is also helpful when assessing true human movement.
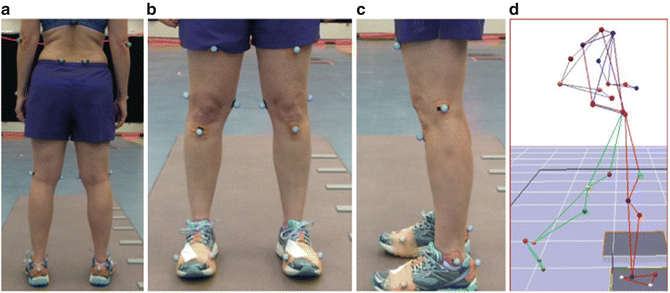
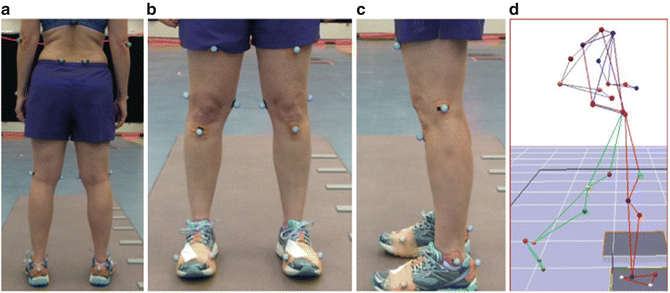
Fig. 3.3
Example of marker placement during running gait assessment; (a) posterior view, (b) anterior view, (c) lateral view, and (d) representation of an associated computer model
The simplest form of motion capture is using a standard video camera (30–60 frames/s) that can view the runner from anterior, posterior, or lateral views. Though there has been evidence for the effectiveness of two-dimension motion analysis [40], especially in regards to observing frontal plane joint projections [41], there are inherent limitations of its use with gait analysis. Transverse plane and combined tri-planer motions are harder to interpret and often less accurate. Due to the subtlety of transverse plane motion, which is out of plane in relation to the view of the motion capture device, true representations of rotational movements are more difficult to appreciate. However, the information gathered can still be useful and may assist a medical professional in directing future management of the healthy or injured runner.
More advanced forms of motion analysis systems have been developed that enable a clinician to readily capture three-dimensional measurements [42]. Though often more expensive, they are feasible for clinical use and provide more accurate representations of movement. With the use of reflective markers, computers assist in the capturing and processing of information regarding motion of limb segments, joint velocities, and joint angles [15].
Multiple high-speed motion capture systems and intricate computer software are also utilized for analyzing running in three-dimensions. Three-dimensional analysis is ideal, and has been found to be accurate in assessing running kinematics [43]. Kinematic data can further be utilized in conjunction with force plate data and electromyography (EMG). However, due to the costliness and longer setup times of such instrumentation, these measures are often utilized for laboratory, experimental gait analysis purposes. The use of force plates will provide information pertaining to how ground reaction forces in the vertical, horizontal, and transverse planes are imposed onto the body during the stance phase of running. This can be done with force plates mounted in the floor. Often, a laboratory setting makes the simulation of a normal running stride difficult and potentially unattainable due to limited space and the location of the force plate relative to the runner’s normal stride [44, 45]. Due to the high stride to stride variance in ground reaction force measurements of both over ground and treadmill running, sufficient steps are preferred and best gathered via an instrumented treadmill [46]. Whether with level ground observation or via instrumented treadmill, measures of vertical loads, horizontal shear, vector patterns, joint torques, and center of pressure are helpful in the prevention and management of bony stress injuries [15]. EMG data is captured either by surface or fine wire electrodes, and can help in the analysis of a runner’s neuromuscular control and patterns of muscle timing.
Running Biomechanical Assessment
For organization and clarity purposes, characteristics of running gait associated with stress fractures will be discussed separately in relation to the cardinal planes of motion (Table 3.3). This will assist a clinician who is performing a running analysis without the resources of extensive instrumentation to identify characteristics reported in the literature to be associated with stress fractures in running athletes.
Table 3.3
Biomechanical considerations for stress fractures during running
Force characteristics during limb loading | • Impact peak amplitude |
• Instantaneous vertical ground reaction force loading rate | |
• Average vertical ground reaction force loading rate | |
• Free moment | |
Sagittal plane | • Foot strike pattern |
• Peak tibial acceleration | |
• Stride and step lengths | |
• Cadence | |
• Peak knee flexion during stance | |
• Peak hip flexion during stance | |
Frontal plane | • Hip adduction |
• Knee abduction | |
• Tibial outward tilting (excessive crossover) | |
• Rear foot eversion | |
• In-toe or out-toe | |
Transverse plane | • Hip internal rotation |
• Knee internal rotation | |
• Tibial internal rotation | |
• Foot pronation/supination |
Sagittal Plane Mechanics
Sagittal plane joint position and motion at the trunk, hip, knee, and ankle must be closely examined to identify possible risk factors that may predispose a running athlete to injuries. This can best be accomplished through inspecting an athlete from the lateral view. Joint positions during loading are highly related to kinetic and kinematic data that may place a runner at risk for injuries such as stress fractures. Reports show that 600,000–1,920,000 runners per year will sustain a stress fracture [1]. Due to this high volume of injuries, attempts to understand the mechanisms of these injuries have been the goal of recent research, with repeated impact loading being deemed the primary root cause [6]. Ground reaction forces, the forces exerted by the ground on a body [47], have been commonly measured as a way to approximate the external load acting on body structures during running.
To fully understand the effects of forces acting on the body, certain terminology should be defined. Loading rate is defined as the speed at which forces are applied to the body and can be identified using accelerometers or by the initial slope of the ground reaction force curve as the foot makes contact with the support surface. The steeper this slope of early stance on the ground reaction force curve, the more rapid the force application onto the body (Fig. 3.4). Vertical average loading rate, vertical impact peak, and vertical instantaneous loading rate seems to be higher in those who sustained overuse running related injuries [48].
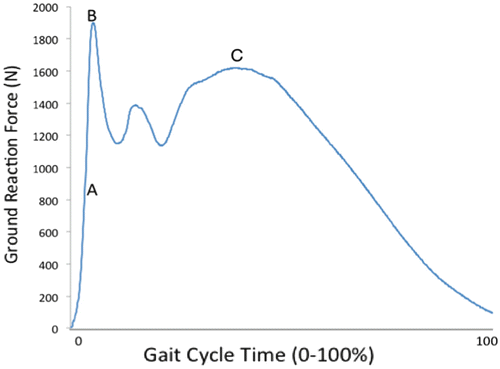
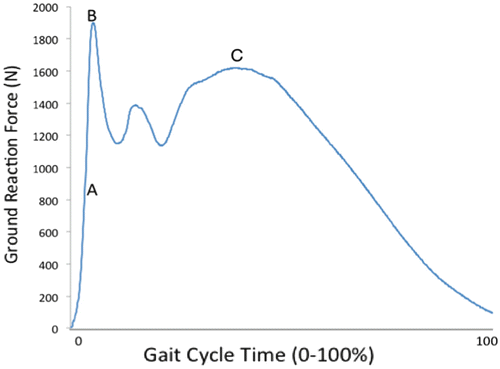
Fig. 3.4
Running ground reaction curve. “A” depicts the slope of the line used for determination of vertical loading rate; “B” depicts the impact peak; “C” depicts the active peak. Courtesy of University of Florida Health Sports Performance Center, Running Analysis
Foot Position and Strike Pattern
The foot is typically divided into three sections, the rear foot, forefoot, and mid foot. The rear foot is made up of the talus and calcaneus, while the forefoot contains the metatarsals and phalangeal bones. The section of the foot between the rear and forefoot is termed the mid foot and houses the remaining tarsal bones, including the navicular, cuboid, and three cuneiforms. The manner and location at which a runner contacts the ground and loads the foot can differ and will alter how the body accepts ground reaction forces (Table 3.4). A rear foot strike pattern is characterized by loading the posterior-lateral heel and the foot dorsiflexed. Conversely, with a forefoot strike pattern the foot in slight plantar flexion and inversion, first loads the anterior aspect of the foot just proximal to fourth and fifth metatarsal heads (Fig. 3.5).
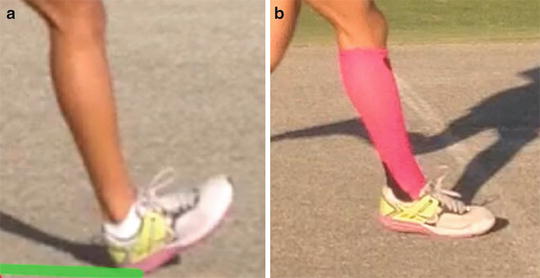
Table 3.4
Differences between strike patterns
Rear foot | Forefoot | |
---|---|---|
Location of foot contact | Postero-lateral heel | Lateral forefoot proximal to fourth and fifth metatarsal heads |
Ankle position at contact | Dorsiflexion | Slight plantar flexion and inversion |
Center of pressure | Posterior lateral to anterior medial | Anterior to posterior (as heel lowers) followed by anterior |
Loading of arch | Mid stance | Impact |
Direction of foot pronation | Rear foot to forefoot | Forefoot to rear foot |
Effect at knee | More work | Less work |
Effect at ankle | Less work | More work |
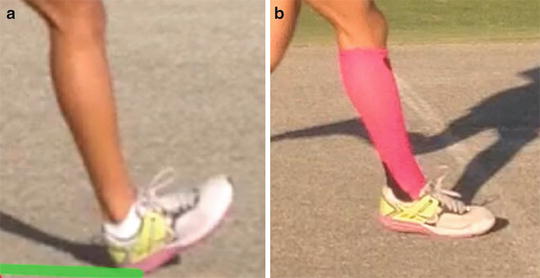
Fig. 3.5
Examples of strike patterns: (a) rear foot strike; (b) forefoot strike
Aside from location of contact, other biomechanical differences exist between strike patterns. As a foot is loaded at the rear foot, the center of pressure is transferred from a posterior lateral to anterior medial aspect of the foot, allowing pronation to occur first at the rear foot then by the forefoot. The medial longitudinal arch and plantar fascia, which assists in shock attenuation, is loaded at mid stance of the running cycle. When a foot contacts the ground more on the forefoot, the center of pressure goes in a posterior direction as the heel lowers to the ground and then changes direction to move anteriorly to prepare for unloading. Pronation occurs in the reverse direction, first working to assist with force dissipation at the forefoot, then at the rear foot. The medial longitudinal arch and associated plantar fascia also works to attenuate forces at impact [49]. Location of strike pattern can be best assessed from viewing the runner from a lateral perspective and identifying both ankle position and location of loading at initial contact.
Various strike patterns have been a topic of continuing debate and highly studied in the running literature [50–53]. Both rear foot and forefoot strike patterns present with inherent benefits and pitfalls in relation to biomechanical links to injury. One study revealed runners who habitually rear foot strike have higher rates of repetitive stress injuries when compared to forefoot strikers, due to an inability of the body to effectively attenuate ground reaction forces [54]. Others, on the contrary, have hypothesized that forefoot striking places the foot and ankle at greater risk of injury, in part due to an increase in eccentric work and strain at the foot and ankle [55, 56].
Stress injuries are often due the repetitive overload due to an inability to properly dissipate associated forces, resulting in micro trauma of bodily tissues. The manner at which the body accepts loading forces varies between the strike patterns and is illustrated in the different shape of their representative ground reaction force curves (Fig. 3.6). A significant correlation exists between tibial shock and vertical ground reaction force loading rates, especially at the early parts of stance phase, and lower extremity stress fractures. Within the first 10 % of the stance and lasting about 10–30 ms, the first peak in force, or “impact transient,” occurs when a heel initially contacts the support surface. The presence of an impact transient is a sudden rise in force following initial ground contact and exemplifies forces acting rapidly on the body [57]. A second peak, the “active peak,” ensues during mid stance and can last up to 200 ms [58]. A rear foot strike contains double impact peaks, while a forefoot striker has an overall flatter curve, lack of an impact transient, and decreased rate of loading [49, 51]. The disappearance of impact transient in forefoot strikers may be attributed to the pre-activation of the triceps surae complex that will assist in the dampening of impact forces [3].
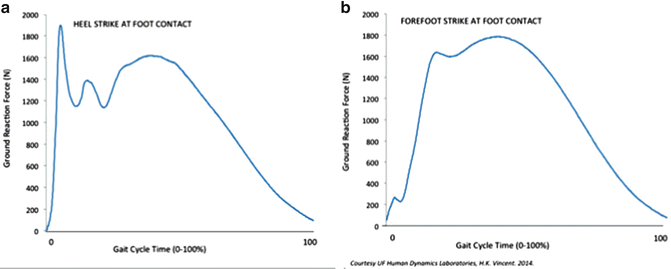
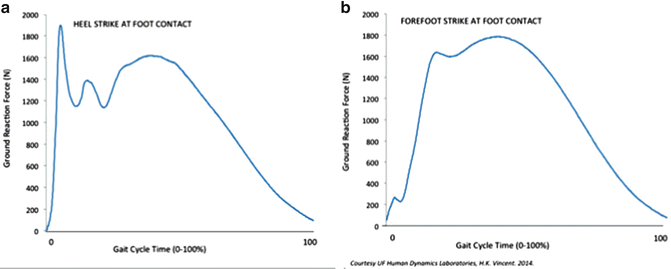
Fig. 3.6
Examples of ground reaction curves demonstrating the (a) rear foot strike significant impact peak and rapid vertical loading rate of a rear foot strike pattern and (b) smoother curvature, lack of impact transient, and slower loading rate of a forefoot strike pattern. Courtesy of University of Florida Health Sports Performance Center, Running Analysis
Different strike patterns will affect how loads are placed on the body along with the runner’s strategies at attenuating these forces. Specifically, the biomechanical demands at the ankle and knee are altered. Compared to a rear foot strike pattern, by landing on one’s forefoot, a runner with a forefoot strike will have decreased overall work at the knee with an increased work at the ankle. This increased demand at the foot and ankle over successive foot strikes may predispose an athlete to stress injuries about the metatarsals and bones of the forefoot [55]. However, the decrease in work at the knee may allow an athlete to resist fatigue and shift the strain away from the knee, which is the most common site for running related injuries.
Hip and Knee
Gaining an appreciation of how impact loads are absorbed by the body is critical when quantifying the risk factors associated with stress fractures. Peak joint flexion ranges at the hip and knee can easily be observed in the sagittal plane. Alterations in the hip and knee joint have been linked to the amount of impact shock during loading at initial contact of the running cycle [59]. It has been determined that an increase in knee flexion during loading enhances dampening of ground reaction forces, thus decreasing injury risk [60, 61]. However, controversy arises as to the negative effects of knee flexion at contact [62]. Though a certain amount of knee flexion is desired to help attenuate shock, excessive knee flexion throughout stance may result in an increase in tibial shock, and a greater risk for stress injury [63]. A more flexed knee at contact will decrease overall knee range of motion excursion and possibly contribute to greater amounts of tibial shock. In conjunction, more knee flexion at loading will result in a more vertical tibial shaft and closely align the long axis of the tibia with the vertical component of the ground reaction force and subsequent tibial shock [62].
Stride Characteristics
Step length is the distance measured from the contact of one foot from that of the other, while stride length is a measure of the distance covered between successive ground contacts of the same foot. Stride length can be readily measured by marking designated increments on the side of the treadmill or along the running surface and calculating the distance between successive foot strikes. Running stride has been found to me much more smooth in competitive runners when compared to their recreational counterparts [64]. Generally, runners also tend to utilize a preferred stride rate and length, however they may not be the most optimal in regards to running economy and force attenuation [65]. Overstriding and an increased braking force has been linked to a history of stress fractures [66, 67], while shortening stride length decreases the risk of stress related injuries [68]. This may be due to the influence of the ground reaction force [69], positive effects on the amount of work required of the hip and knee [70], shorter times spent in the support phase, and pre-activation of leg musculature [65]. Magnitude and direction of ground reaction force vectors have been associated with tibial stress fractures by increasing the bending load acting on the tibia. It has been determined that a more posteriorly directed sagittal plane ground reaction force vector will increase the bending load acting on the tibia [69]. This posteriorly directed force is seen in runners who contact the ground in front of their base of support, regardless of strike pattern [67].
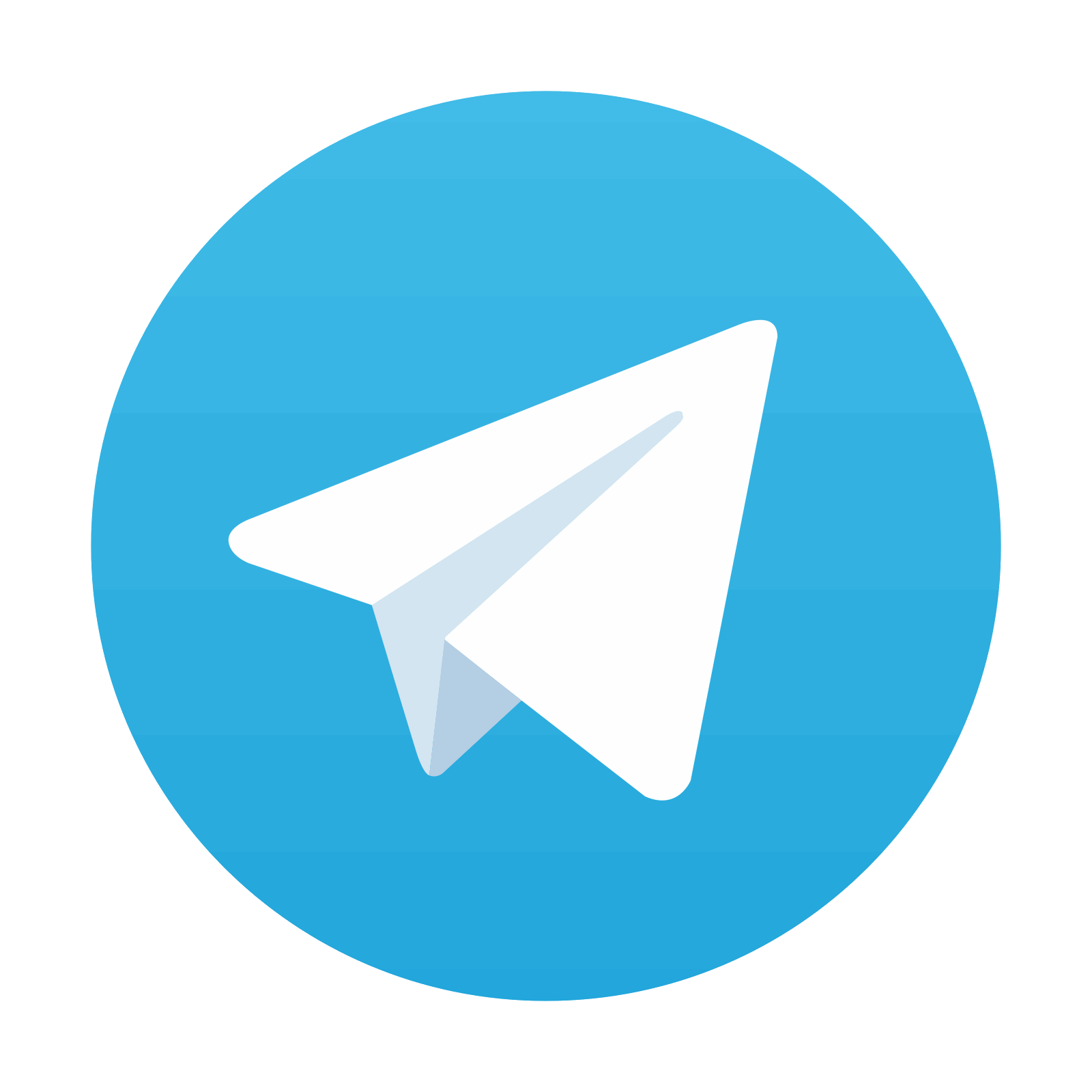
Stay updated, free articles. Join our Telegram channel

Full access? Get Clinical Tree
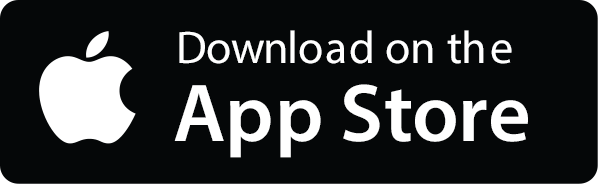
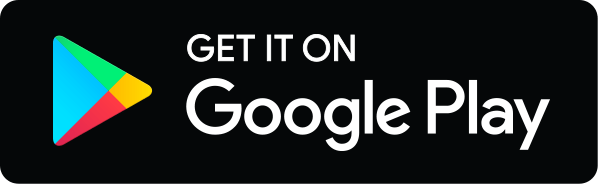