Biomechanics and Clinical Functionof the Rotator Cuff
Introduction
Normal Function of Rotator Cuff
1. Glenohumeral Joint Stability
2. Shoulder Motion
3. Force Couples About the Glenohumeral Joint
4. Biomechanical Aspects of Clinical Examination for Rotator Cuff Disorders
Pathologic Condition of Rotator Cuff
1. Changes in Joint Kinematics With Rotator Cuff Pathology
2. Partial-thickness Rotator Cuff Tears (PTRCTs)
3. Full-Thickness Rotator Cuff Tears
4. Massive Rotator Cuff Tears
(From Nguyen ML, Quigley RJ, Galle SE, et al. Margin convergence anchorage to bone for reconstruction of the anterior attachment of the rotator cable. Arthroscopy. 2012;28(9):1237–1245.)
5. Rotator Cable and Crescent
(From Park MC, McGarry MH, Gunzenhauser RC, Benefiel MK, Park CJ, Lee TQ. Does transosseous-equivalent rotator cuff repair biomechanically provide a “self-reinforcement” effect compared with single-row repair? J Shoulder Elbow Surg. 2014;23(12):1813–1821.)
Biomechanics Related Rotator Cuff Repair
1. Different Rotator Cuff Repairs and Their Biomechanical Effects
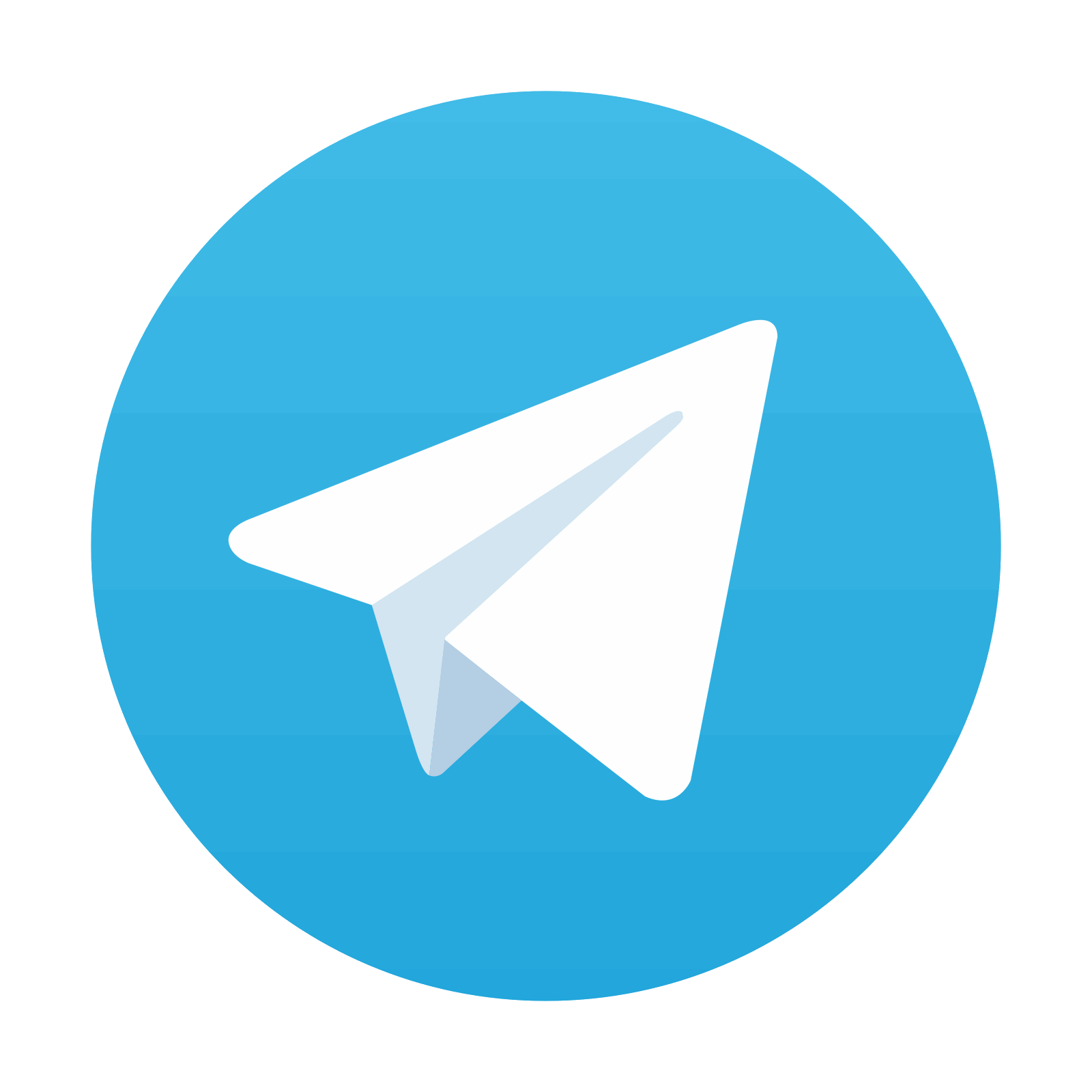
Stay updated, free articles. Join our Telegram channel

Full access? Get Clinical Tree
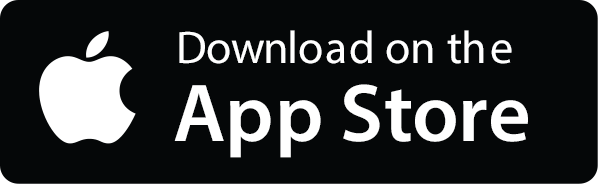
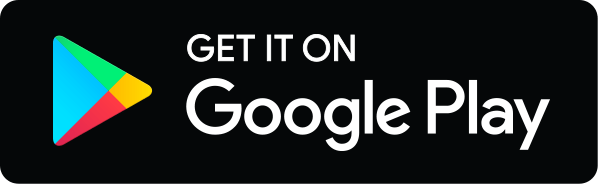